What was it like when the first “polluted” stars formed?
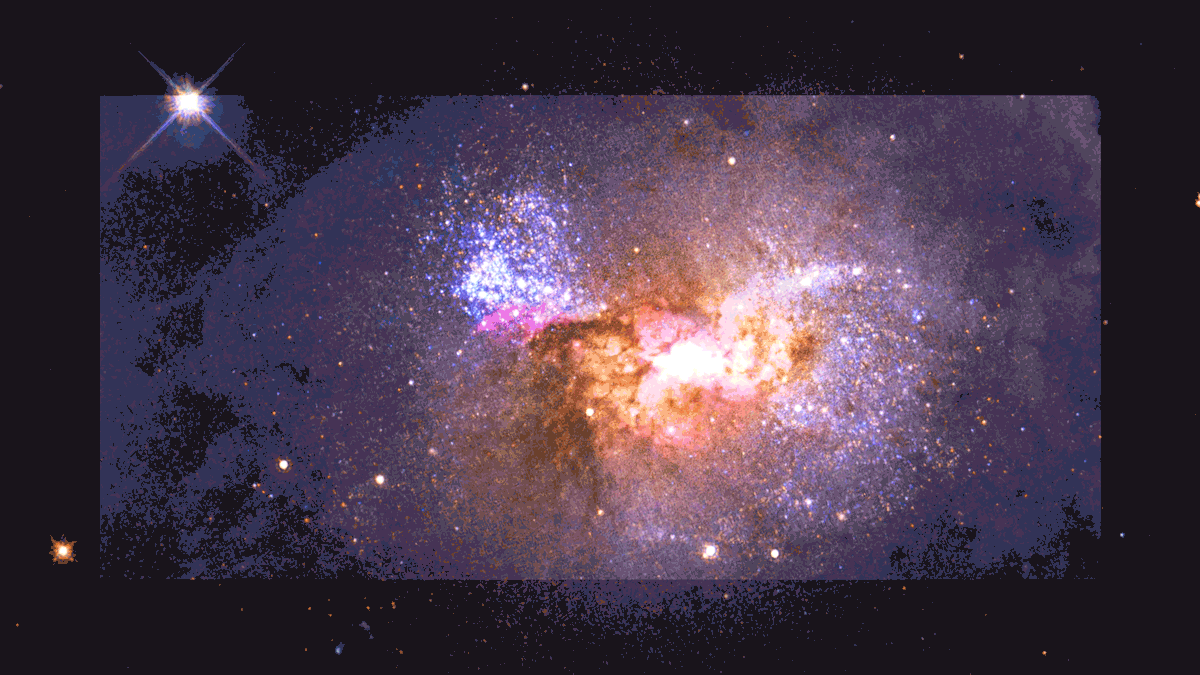
- The first stars to form were made of hydrogen and helium alone, but when they die, they rapidly fill the interstellar medium with recycled, enriched stellar material: full of heavy elements.
- When new stars then form from the ashes of the previous generation, they are no longer pristine, but polluted with the material arising from the corpses of their predecessors.
- Those new stars, the second (and subsequent) generations of stars, are fundamentally different from the pristine ones that came before. Here’s the science of precisely how.
When you look out at the Universe today, and see the vast, dark, backdrop littered with points of light that correspond to stars and galaxies, it’s difficult to imagine that it used to be almost identical everywhere. The Universe, back at its inception, was almost perfectly uniform on all cosmic scales. It was the same high temperature everywhere, the same large density everywhere, and was made up of the same quanta of matter, antimatter, dark matter, and radiation in all locations. At the earliest times, the only differences that existed were minuscule, at the 0.003% level, seeded by the quantum fluctuations imprinted during inflation.
But gravity and time have a way of changing everything. Over time, the excess antimatter annihilates away; first atomic nuclei and then neutral atoms form; over millions of years, gravity pulls matter into overdense regions, causing them to grow. Because overdensities differ by such great amounts on all scales, there are regions where stars form rapidly, within 100 million years or fewer, while other regions won’t begin forming stars for billions of years. But wherever the earliest stars form, that’s where the most interesting things happen first, including the existence of the second generation of stars: the first polluted stars in all of cosmic history.
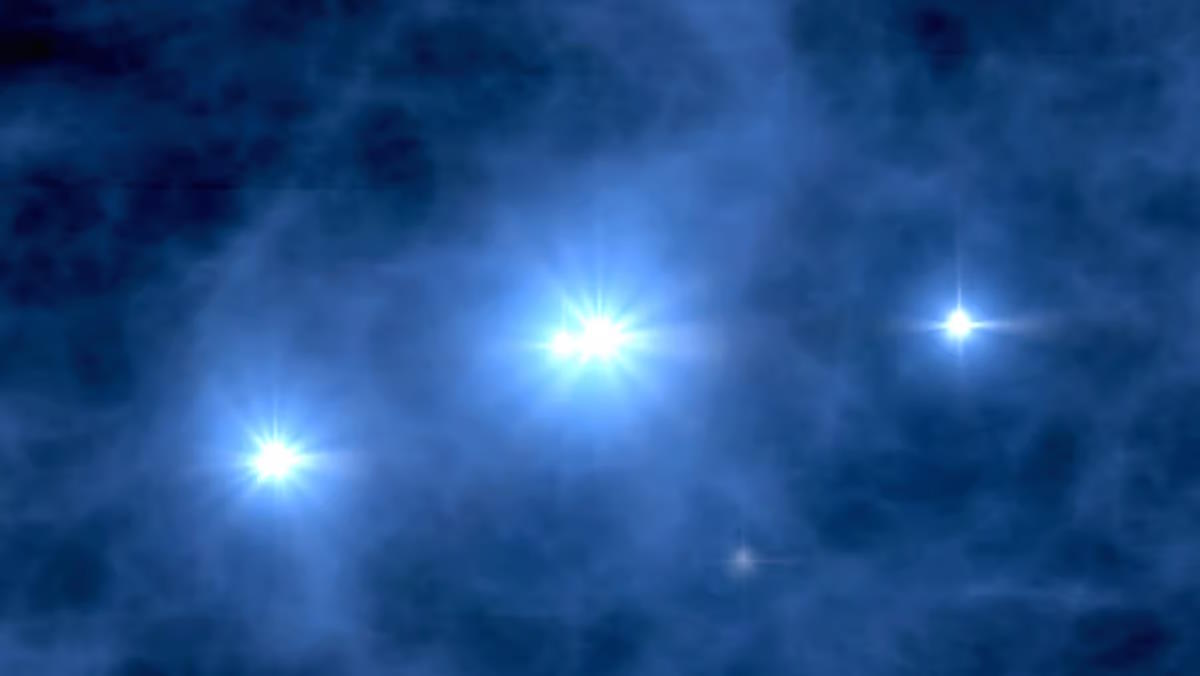
The very, very first stars are born in the most initially overdense regions of all, which grow by attracting surrounding matter the fastest. The gravitational growth of matter leads to the first stars forming somewhere between 50 and 100 million years after the Big Bang, with those stars being much more massive than the stars we see today. Because there’s so much mass inside them, undergoing the rapid, high-temperature reactions of nuclear fusion, they live fast. Within just a few million years, they’ve burned through all of their core’s fuel, leading to their dying in either a supernova or by directly collapsing to form a black hole.
And wherever this happens, that’s the end of the line for this population of “first stars,” which are the stars made exclusively of the pristine material (hydrogen and helium) forged in the crucible of the hot Big Bang. The outer layers of the stars that went supernovae, making up the majority of the former star’s mass, get blown off back into interstellar space, further enriched by the rapid absorption of neutrons. The supernova remnants that leave behind neutron stars, many of which are in binary systems, have a chance to collide with other neutron stars, giving rise to gamma-ray bursts, kilonova events, and the heaviest of the elements. All of a sudden, the Universe, in these regions, is not solely composed of hydrogen and helium anymore.

After all the time required for the first stars to form — perhaps as little as 50 million in the densest places, typically between 200 and 550 million in most locations, but not for 2 or 3 billion years in the rarest, longest-lived pristine regions — they run out of fuel and die in as little as 1-5 million years. These very first stars, made out of the pristine elements formed just 3-4 minutes after the Big Bang, cannot survive for very long, as they’re all quite massive compared to the types of stars that primarily exist today. Once you make a sample of the “first stars,” you only have a short window before something other than hydrogen and helium exists in those regions.
Once the most massive stars among the “first stars” die, the interstellar medium becomes enriched with those heavy chemical elements. The atoms that exist are no longer solely hydrogen and helium (plus one-in-a-billion parts lithium) with nothing heavier. Suddenly there are abundant levels of carbon and oxygen, with copious amounts of silicon, sulfur, iron, nickel, and cobalt, plus all the elements made in supernovae and kilonovae, rising all the way up the periodic table. It’s not just from hydrogen and helium, but also with these enriched materials, which now flood the interstellar medium, that the next generation of stars will form.
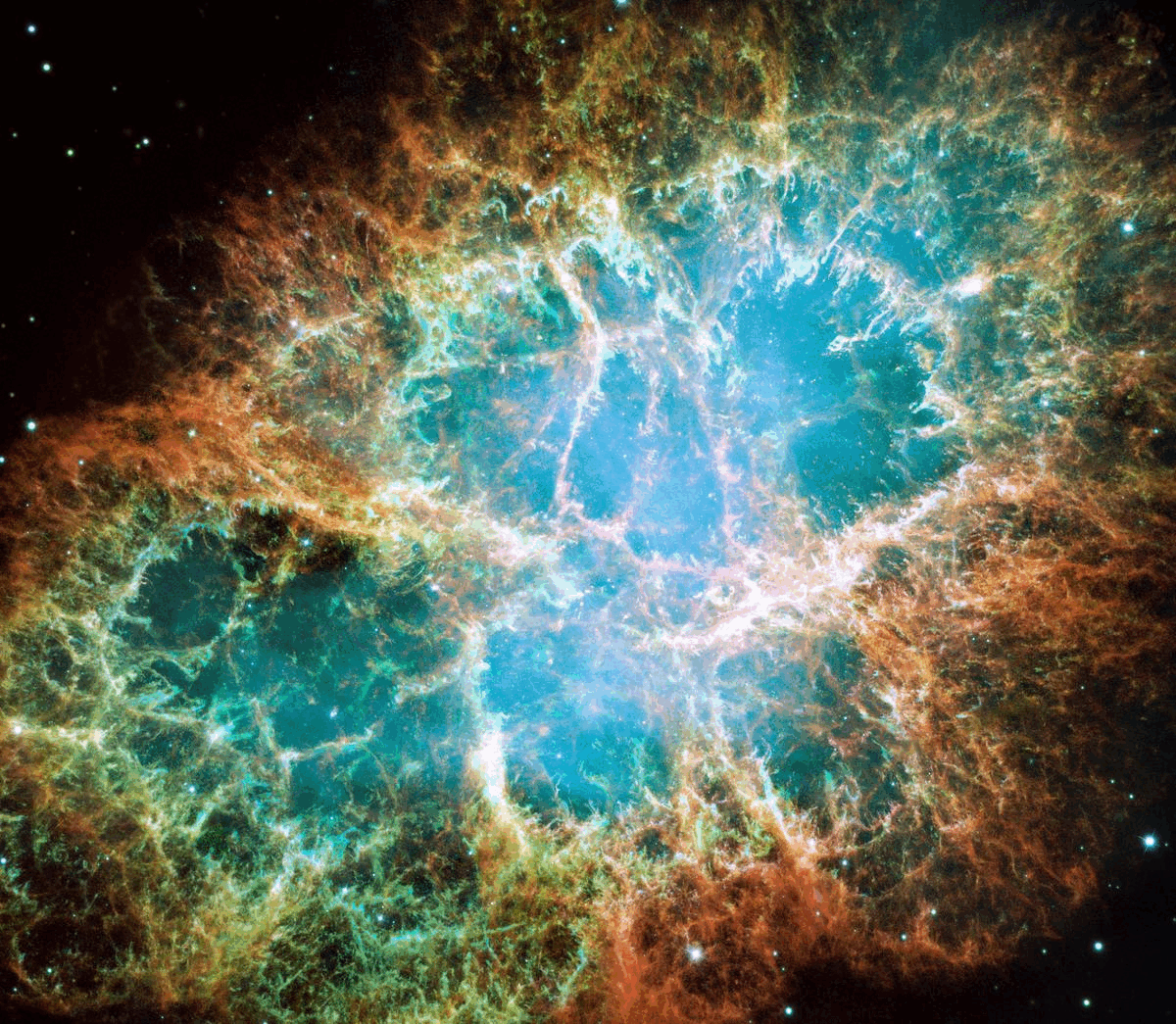
From examining the closest supernova remnants to us, including the Crab Nebula, we can infer that every supernova explosion pushes material outward at approximately the rate we observe there: creating a nebula 10 light-years across after approximately only 1,000 years, indicating that it’s expanding at around 1% the speed of light. Wherever the debris from the deceased first generation of stars cannot yet reach, the stars that eventually form there will still be pristine, since there’s no way for that processed material to make it into those pre-stellar nebulae. The existence of stars in one region of space doesn’t preclude pristine stars from forming nearby, just not in an overlapping region.
But wherever the debris does reach, all of a sudden the material that’s available to form stars is now full of atoms that contain heavier atomic nuclei. It might seem silly to you, under most circumstances, that astronomers classify “hydrogen” and “helium” separately, and then throw every element heavier than helium into its own class — and that we call them “metals” regardless of their other physical or chemical properties — but the existence of these heavier-than-helium elements really plays a tremendous role in the formation and evolution of stars.
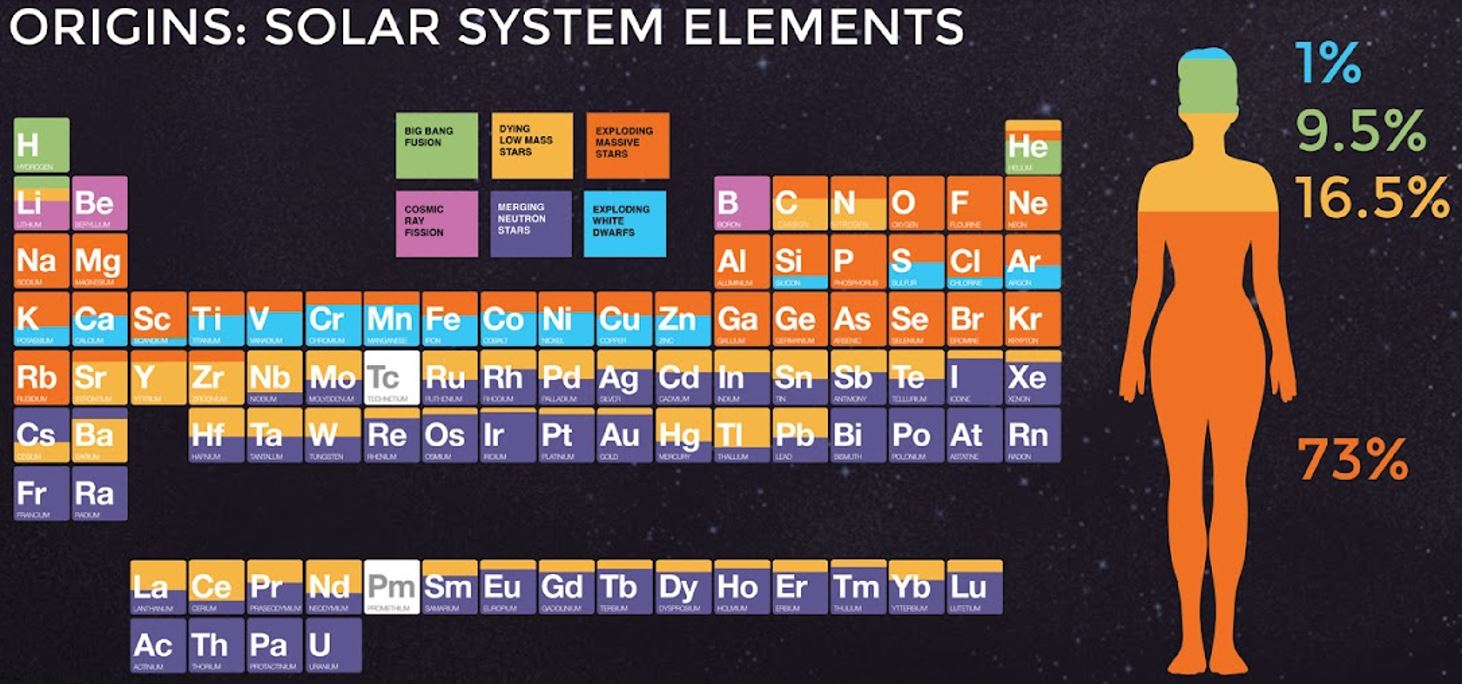
You see, whenever stars are formed out of hydrogen and helium alone (i.e., in what astronomers would call a metal-free environment), there’s no efficient way for them to radiate away the heat generated by gravitational collapse. As mass from the outskirts falls in toward the center, potential energy gets converted to kinetic energy, and that kinetic energy, or the energy of motion, causes matter to heat up. If you can radiate that heat away, you can form stars from that material. If you can’t, you need to gather comparatively enormous clumps of matter in order to overcome that kinetic energy and trigger gravitational collapse, which means that the pristine first stars must have been extremely massive stars, even on average.
But once you have metals present, even if they’re just 0.001% (one part in 100,000) of the total fraction of atoms, they can serve as the excellent energy-radiators that the first stars lacked. As a gas cloud containing these heavy elements collapses, those heavy elements efficiently radiate that excess heat away much more efficiently than before, allowing the clumps of matter that exist to collapse into proto-stars not only much more quickly, but also possessing much lower masses. The first “polluted” stars are much more like the stars that form today than the very first stars of all.
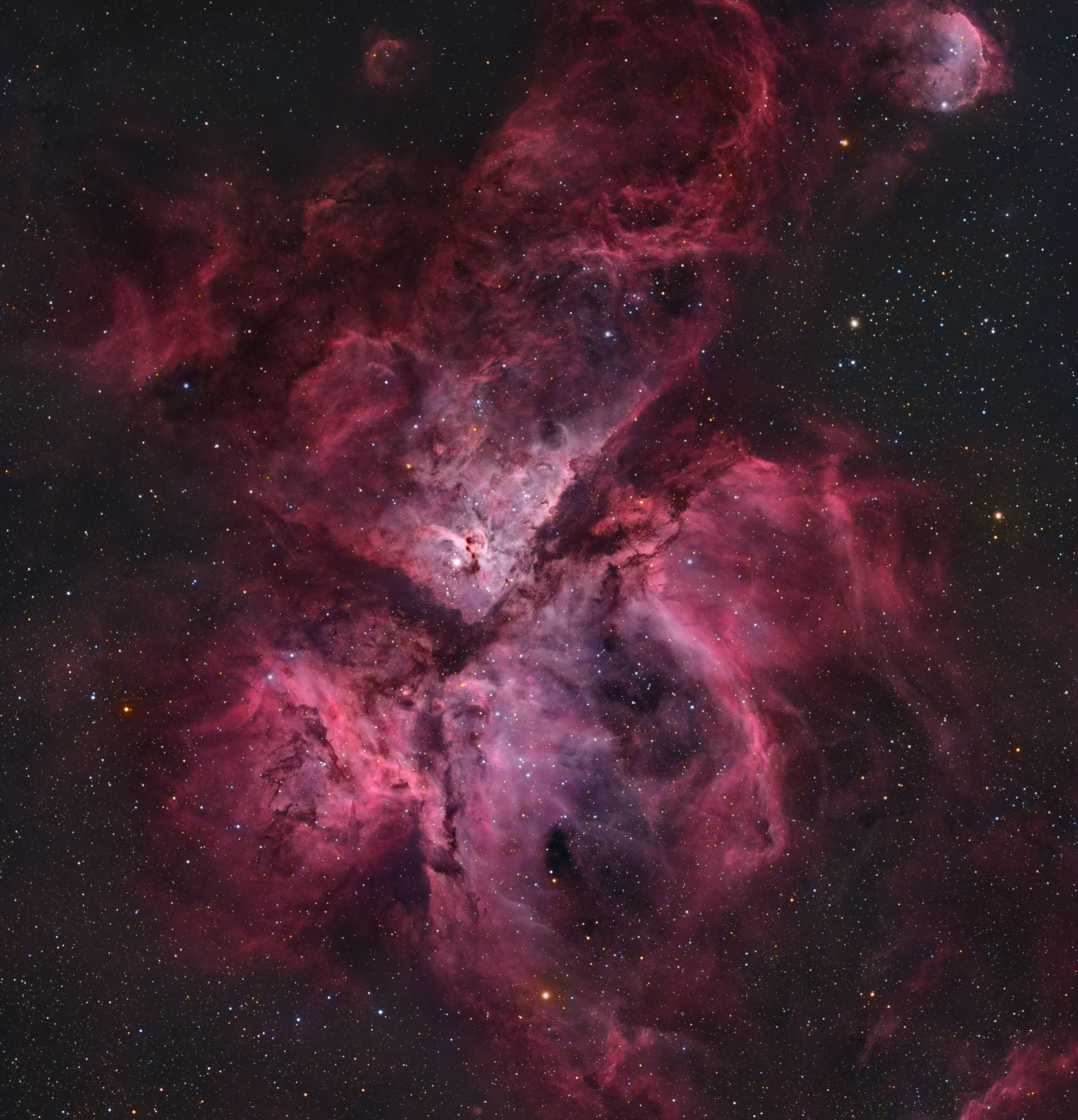
Additionally, wherever you have supernovae, kilonovae, and other violent, cataclysmic events occurring nearby, they don’t just enrich the interstellar medium surrounding them. The shockwaves and propagating waves of matter, as they travel through space, will smack into surrounding gas clouds, where they can serve as a trigger for the gravitational collapse of those clouds, leading to new star formation events. The first stars don’t just provide the materials for a second generation of stars to form, but also the impetus, especially in a gas-rich environment, for their formation to commence almost immediately.
What does this result in? The biggest implications are that, shortly after the first stars form, live, and die, another new generation of stars will soon crop up, wildly different in character than the first generation that was formed by pristine materials alone. These second-generation stars will no longer weigh in at around 10 solar masses, on average, but rather will run the full gamut of star sizes and masses that we know today. Perhaps, if our understanding of star formation is correct, they’re similar to the stars that form locally: with only about 0.4 solar masses, on average, with many being higher in mass but the majority being even lower in mass.
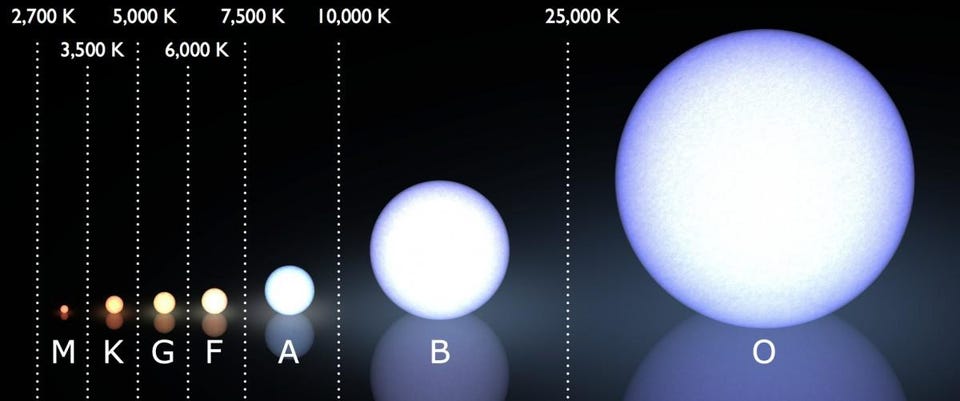
Yes, there will still be a few large, massive stars, extending up into the hundreds of solar masses, but even the most massive among them won’t be comparable to the biggest and most massive examples found among the first stars. There will soon be additional supernovae, neutron stars, and kilonovae that result as well, which again enrich the interstellar medium further and eliminate adjacent “pristine” populations of matter. In very short order, the earliest, first stars that form will wipe themselves out wherever they exist, only to be replaced by this second generation of stars, which are rife with smaller, redder, and less massive members that weren’t present among the first stars.
As a result, in the very young Universe, we expect to see populations of first stars, which are exclusively hot and blue (and short-lived), living alongside regions that contain more evolved populations of stars. Those “polluted” regions, which already have black holes, second-generation stars, and low-mass, low-luminosity stars among them, will swiftly replace the pristine populations that preceded them. The quest to find the truly first stars faces the challenge that one must disentangle them, wherever they exist, from the subsequent polluted generations of stars that will swiftly arise from the ashes of the shortest-lived first stars.
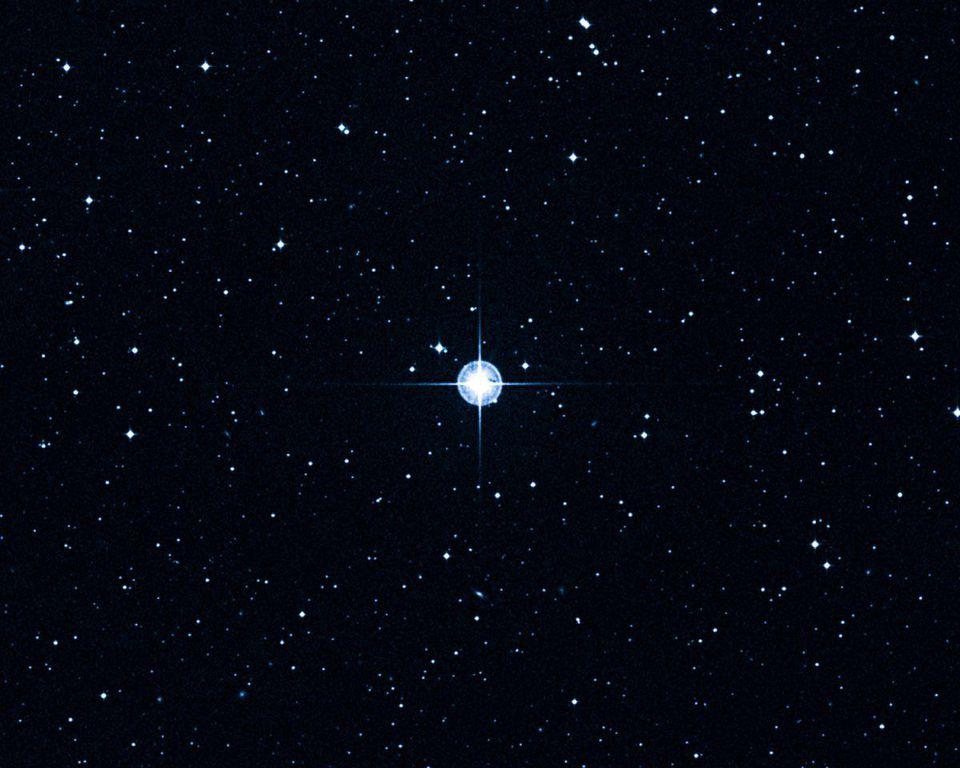
No one, to date, has ever found definitive evidence for even a single first-generation star, as every environment we’ve found contains a mix of red-and-blue stars, indicating that a significant amount of pollution has already infiltrated these stellar populations. The very first stars, perhaps surprisingly, are counterintuitively known among astronomers as Population III stars. Why? Because stellar populations were named in the order they were discovered.
- The Sun is a Population I star, but it’s highly processed and made out of metal-rich material that has gone through many generations of stellar life-and-death.
- The second population ever discovered, Population II stars, are these metal-poor stars that form as early as the second generation of all stars. They can live an extremely long time, and a few of them, like the famed Methuselah star, are still around in our galaxy today, despite being over 13 billion years in age.
- But Population III stars have yet to be discovered; they ought to exist, but are only theoretical at this point. The observational evidence for them has not yet arrived.
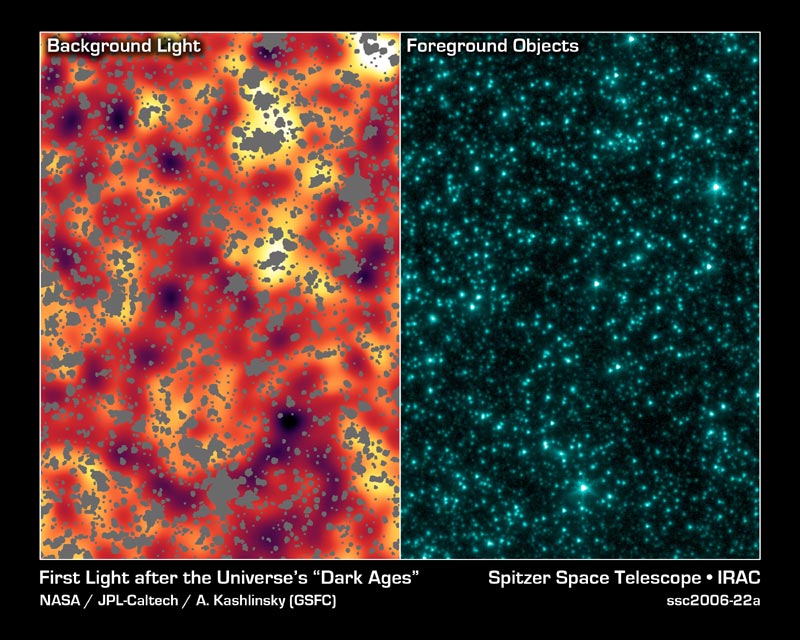
In addition, there’s one more fundamental difference between Population II stars and Population III stars: the possibility of having planets around them. The very first stars, composed of hydrogen and helium alone, could only conceivably create tenuous, massive, puffy gas giant worlds. Without a massive, dense core, they’re easily evaporated and dissociated by the radiation emitted by stars, particularly if those stars are massive, ultra-luminous, and rich in ionizing, ultraviolet radiation. It’s speculated by many that planet-formation around Population III stars may be unsustainable and pragmatically impossible.
But once you have the presence of metals, all of a sudden you can form dense, rocky clumps in your protoplanetary disk, which leads to a mix of rocky and gaseous planets. Once you make the second generation of stars, you can make planets too, complete with complex and even organic molecules. Although it has been demonstrated, observationally, that fewer than 2% of all exoplanets that exist have been found around stars with less than a quarter of the heavy elements found in the Sun, there are some low-metallicity examples of stars with planets. Among the pristine ones, however, it’s likely not even possible.
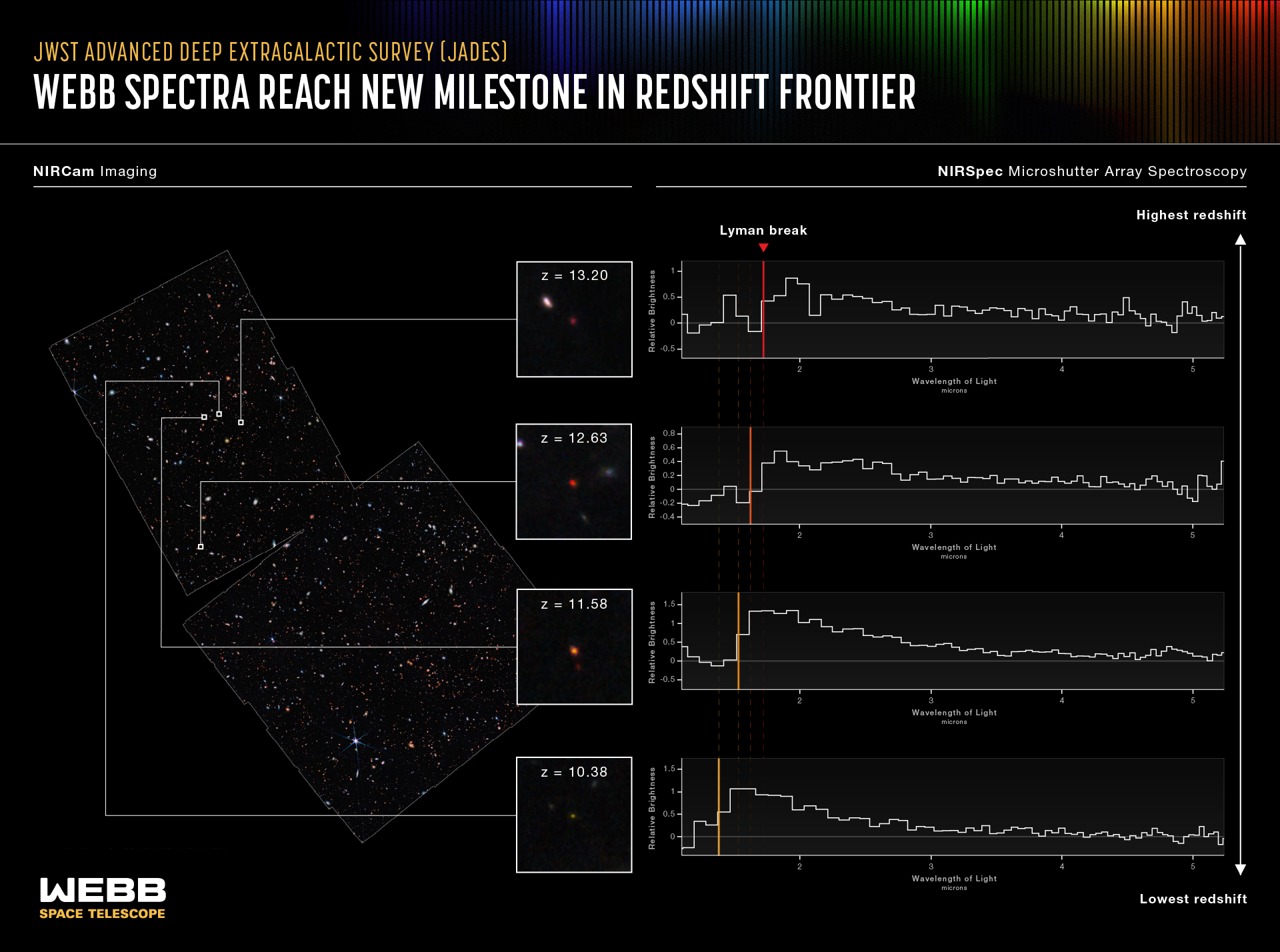
The very first stars live for only an extremely short time, owing to their high masses and large luminosities and rates-of-fusion. Once they die, ejecting enormous amounts of now-processed material back into the interstellar medium, the space around them becomes polluted with the fruits of their lives: heavy elements. These heavy elements enable the second generation of stars to form, but they now cause stars to form in a different manner from before. The heavy elements efficiently radiate heat away, giving rise to a less massive, more diverse generation of stars, some of which can survive even to the present day.
Now that we have observatories like JWST performing their science operations, many astronomers hold out hope that this and other novel observatories (such as ALMA) may yet reveal a population of these first stars. However, because they’re likely to be found alongside polluted, second-generation stars, disentangling those two populations and “proving” that we are seeing Population III stars is a daunting challenge. But once these second-generation stars begin to form, they make something else possible: the first galaxies. And that, as we’re finding out already, is where JWST is truly pushing back the cosmic frontiers.