The sober truth about finding the Universe’s first stars
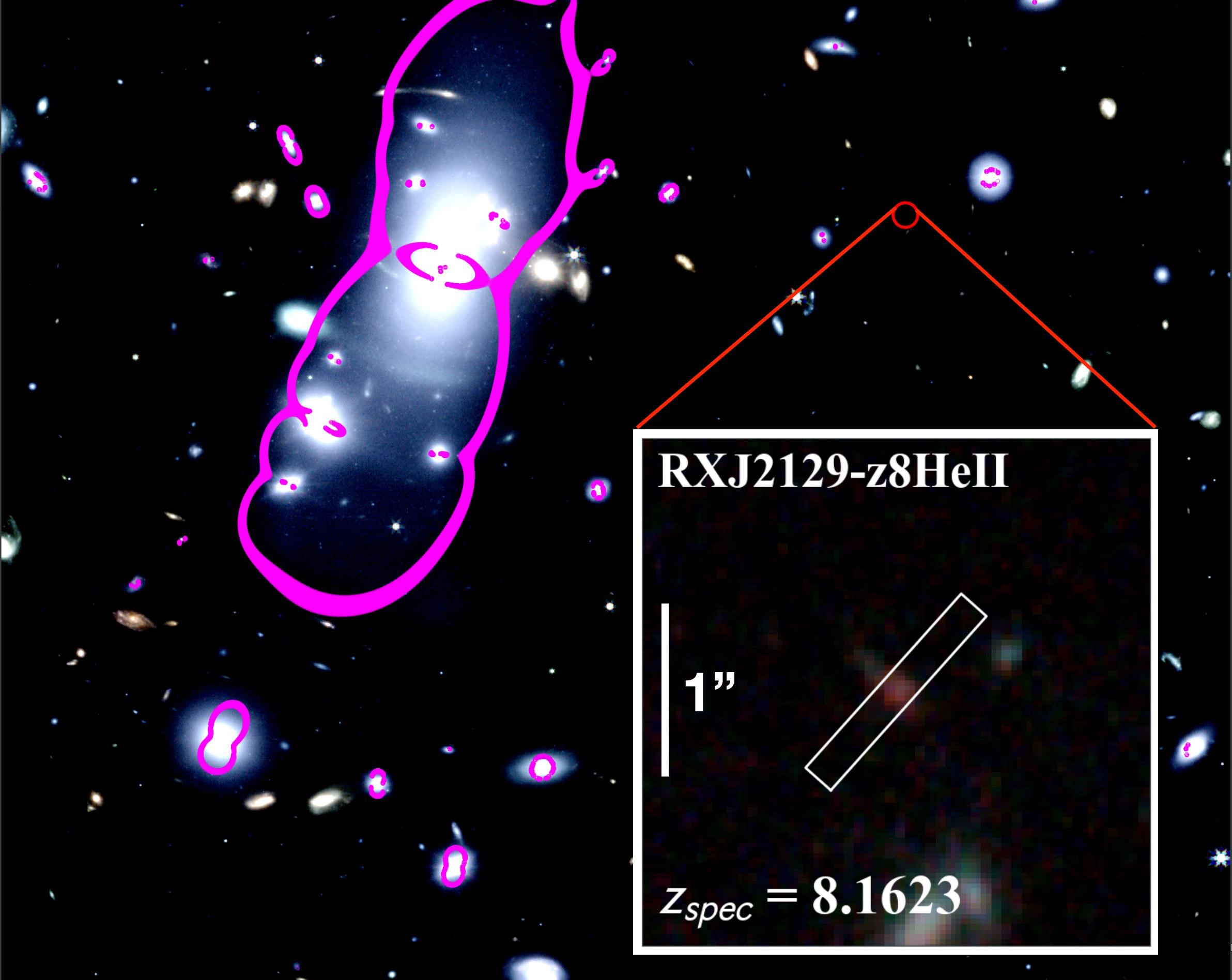
- In a wolf-crying move without sufficient evidence, a team of astronomers in December of 2022 claimed to have discovered "Population III" stars: the first type of star ever to form in the Universe.
- However, the signature that they claimed to have detected is insufficient, on its own, to determine whether they've detected pristine or enriched stars.
- The normally responsible Quanta magazine, botching a high-profile report for the second time in two months, fell for many bogus claims. Here's what you should know if you want the correct info.
In this Universe, there are many things that we’re certain must exist, even though we haven’t discovered them just yet. These gaps in our understanding include the very first stars and galaxies: objects that didn’t exist in the early stages of the hot Big Bang, but that exist in great abundance later on. Although the Hubble Space Telescope and, more recently, the JWST have brought us back very close to the earliest objects of all — with the current record-holder being a galaxy whose light comes to us from just 320 million years after the Big Bang — but what we’re finding isn’t quite pristine.
Instead, the most distant, ancient objects we see are still quite evolved, showing evidence that stars have formed within them previously, rather than what we’re still seeking: gas that’s forming stars for the very first time. Just like many “firsts” in science, there are many teams out there making very strong claims that the evidence doesn’t quite support, like the claim that we’ve just spotted an example of these pristine, so-called “Population III” stars in a distant galaxy: evidence for the Universe’s first stars. Despite an uncharacteristically error-riddled article by Quanta Magazine lauding this possible detection, the evidence simply isn’t there to make such a claim.
Let’s cut through the breathless hype, and expose the sober truth behind it.
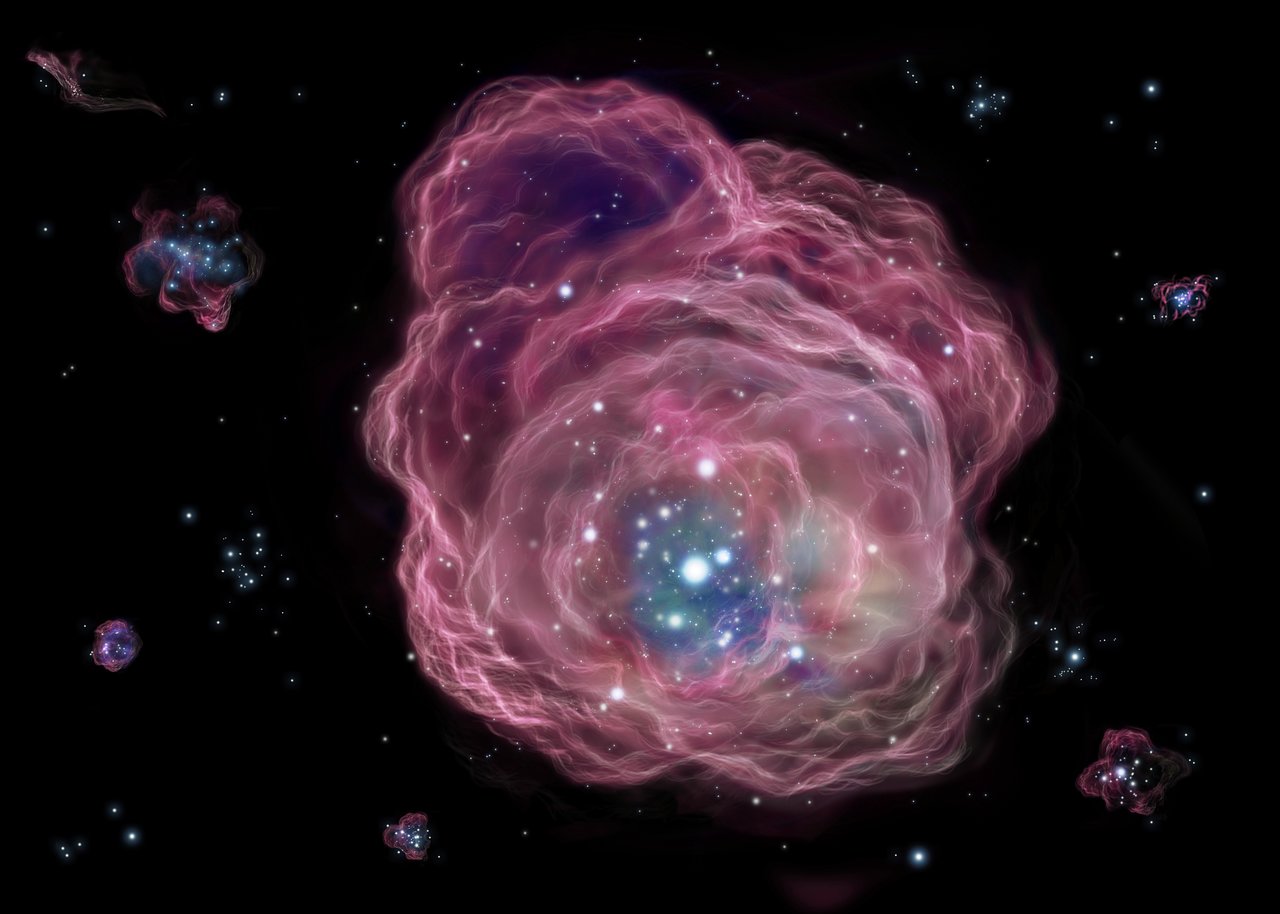
A very brief history of the Universe — at least, the Universe according to our best current theories and observations — might look as follows:
- cosmic inflation occurs, seeding the Universe with quantum fluctuations on all scales,
- inflation ends, giving rise to a matter-and-radiation-filled Universe in an event known as the hot Big Bang,
- wherein the quantum fluctuations (in energy) get turned into density fluctuations on all cosmic scales,
- and the Universe then expands, cools, gravitates, and experiences matter-and-radiation interacting,
- giving rise to the stable formation of protons and neutrons,
- which experience nuclear fusion, forming and hydrogen and helium nuclei, plus a tiny amount of lithium,
- which, as part of a plasma, gravitationally attract while the radiation pushes back against this attraction,
- and then the Universe cools enough so that neutral atoms stably form,
- followed by the neutral matter gravitating and attracting matter, in the overdense regions, from the surrounding average and below-average density regions,
- until a critical threshold is reached, so that the matter collapses to trigger the formation of stars,
- which live, burn through their fuel, and die, enriching the surrounding environment,
- and then accrete more matter and even merge together with other stars, star clusters, and overdense regions, building up the earliest proto-galaxies and galaxies,
- which then continue to grow, evolve, and merge within the expanding Universe.
As you might suspect, we have observational evidence, both direct and indirect, for many of these steps having occurred, but there are many gaps as well: where we strongly suspect these exact steps occurred, but don’t have the surefire observational evidence.
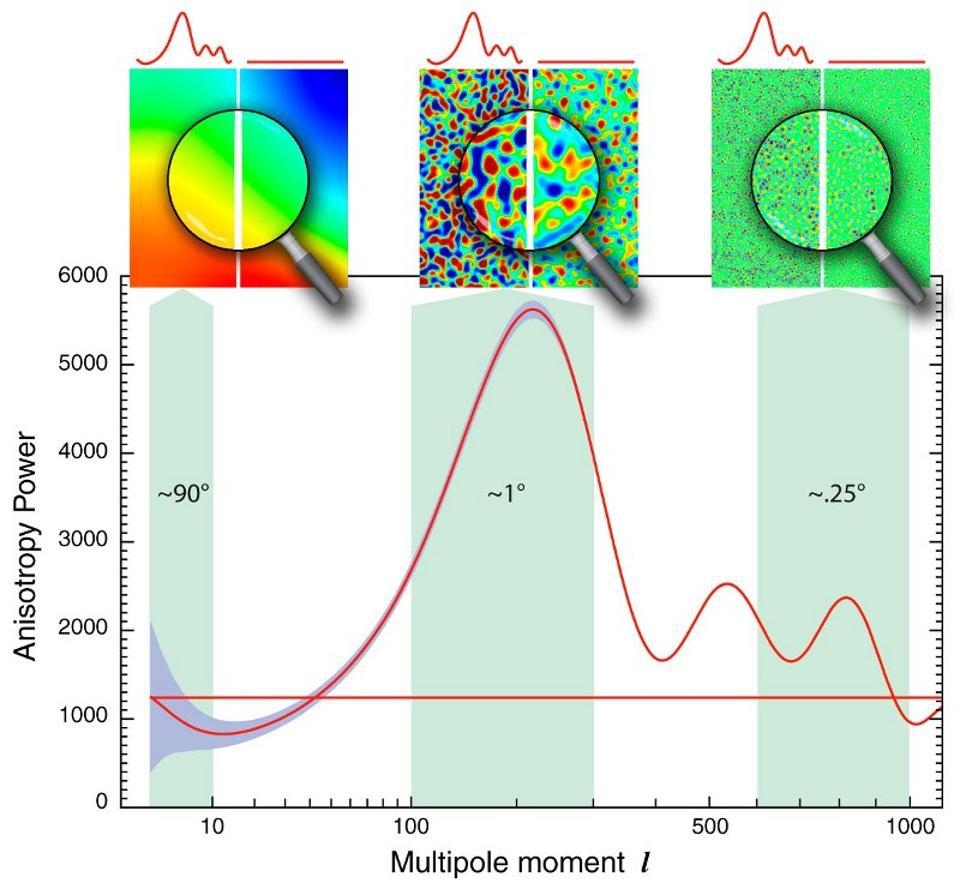
We do, however, have strong evidence for a number of these steps in the Universe’s past. We know about the spectrum of density fluctuations that the Universe was born with shortly after the Big Bang (above, straight line) because of what we observe when neutral atoms first form (above, wiggly line) and the physics of how matter-density imperfections evolve in an expanding, ionized, radiation-rich Universe.
We also know, from the science of Big Bang Nucleosynthesis and the observed abundance of the lightest elements (hydrogen, deuterium, helium-3, helium-4, and lithium-7), what the pristine ratio of these various elements was to one another prior to the formation of the very first stars.
And finally, from the stars and galaxies we do see, both nearby and at great cosmic distances, we know that we’ve only identified galaxies where other, heavier elements that require prior generations of stars — elements such as oxygen, carbon, and the other so-called “alpha” elements that go up two-at-a-time on the periodic table from oxygen (neon, magnesium, silicon, sulfur, etc.) — are also present along the more pristine hydrogen and helium.
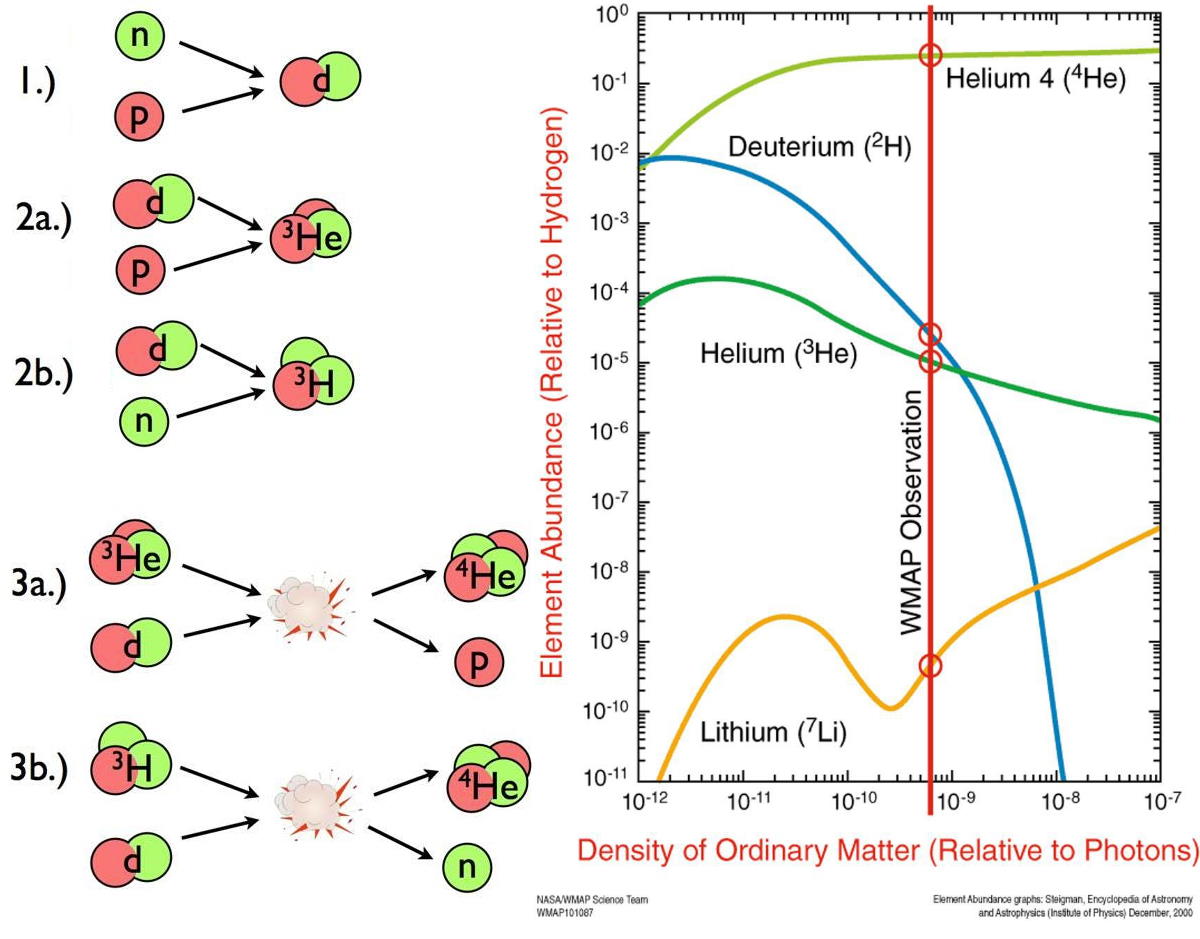
One of the things that the Quanta Magazine piece reported on — partially correctly — is that there has been an idea floated within the community seeking the first stars for how to possibly detect them: through a signature of ionized helium. They incorrectly report that this is a signature of helium-2, which is not even close to the truth. Let’s disentangle what’s true from what’s not.
When scientists talk about the elements, we commonly refer to them by their name with a number after them: helium-2, helium-3, and helium-4, for example. The name of the element, helium in this case, tells you how many protons are in its atomic nucleus: 2, since helium is the second element on the periodic table. The number after the name tells you the total mass of the atomic nucleus, which is the number of protons plus the number of neutrons. Therefore, helium-2 is two protons and no neutrons, helium-3 is two protons and one neutron, and helium-4 is two protons and two neutrons.
Helium-3 and helium-4 are stable; once you make them, they live until they participate in a nuclear reaction: the only type of reaction capable of destroying or altering them. Helium-2, on the other hand, is known as a diproton and is only produced in the nuclear fusion that takes place in stars: the first step in the proton-proton chain.
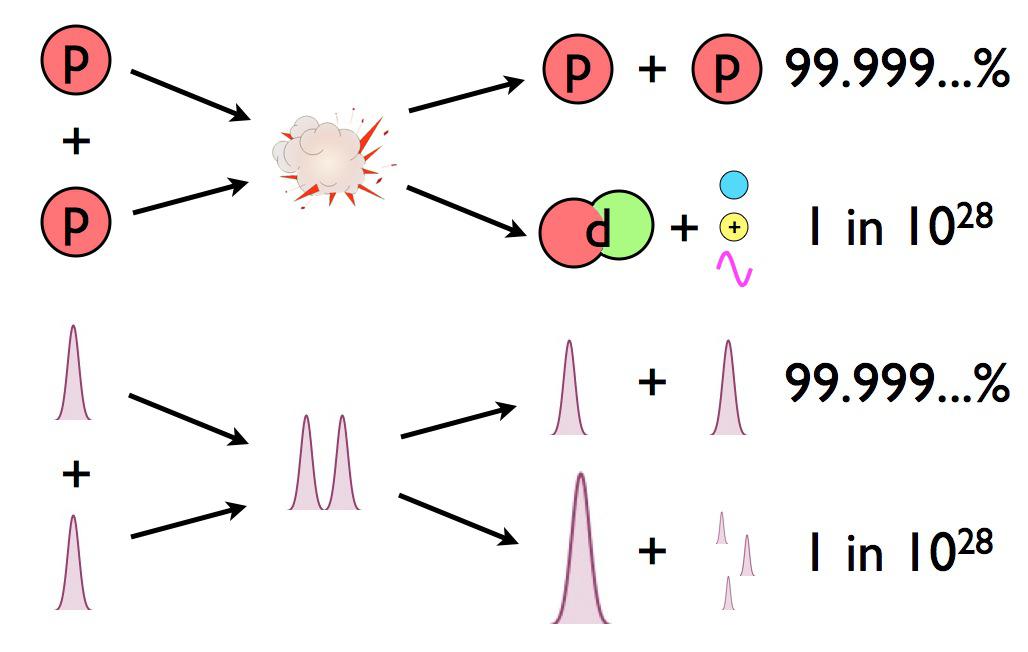
A diproton, or a helium-2 nucleus, has a mean lifetime of less than 10-21 seconds: a blink of an eye on both cosmic and nuclear scales. Most frequently, this unstable nucleus simply disintegrates back to the two protons that originally formed it; however, one out of a very large number of diprotons will instead undergo a weak decay, with one of the protons decaying into a neutron, a positron, an electron neutrino, and (often) a photon as well. The fact that a diproton, or helium-2, can decay into a deuteron, or hydrogen-2 (with one proton and one neutron), is what enables nuclear reactions to occur inside most stars, including our Sun.
But there is no source or reservoir of helium-2 that’s stable and/or detectable; that has nothing to do with what astronomers are searching for. Instead — and this is a vitally important distinction — astronomers are searching for ionized helium, which is sometimes written as either He II or He[II] in the literature. That’s because:
- He[I] refers to neutral helium, or a helium nucleus with two electrons around it (to balance the electric charge of the two protons in the helium nucleus), which applies to all helium atoms at temperatures below ~12,000 K.
- He[II] refers to once-ionized helium, or a helium atom with only one electron around it, which occurs for helium at temperatures between ~12,000 K and ~29,000 K.
- And He[III] reverse to doubly-ionized helium, or a bare helium nucleus with no electrons around it, which occurs at temperature of ~29,000 K and up.
Heavier elements, of course, can be ionized more times with more energy, but helium can only be ionized twice, at most, because of the number of protons in its nucleus.
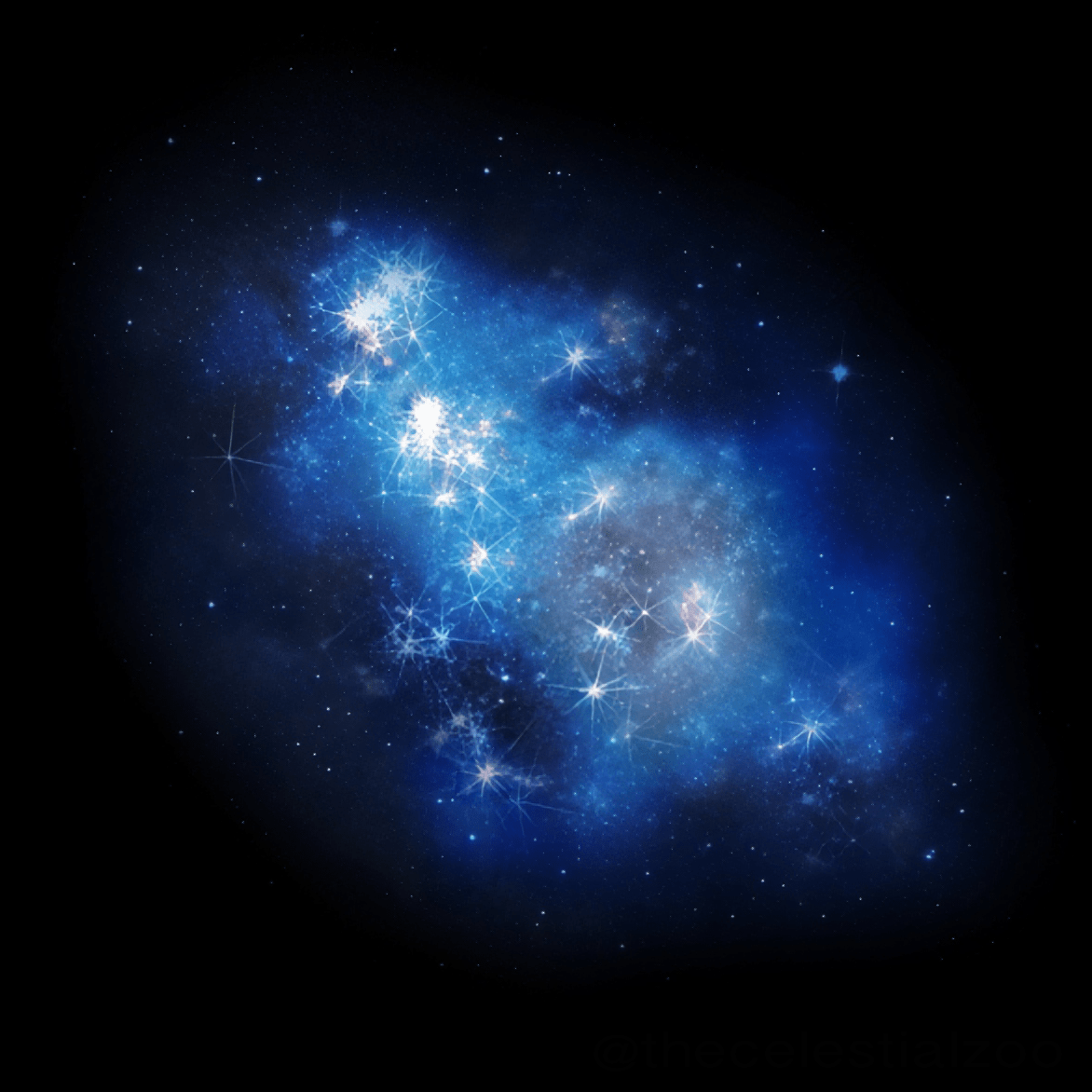
We fully expect that the Universe must have formed stars out of the earliest, pristine material available to it, and that only once that first generation of stars has already lived-and-died can subsequent generations, made with the enriched, heavier elements that were created in that first generation, come into existence.
There’s a lot that we don’t know about these very first stars: the stars that we call Population III stars. (Why? Because stars that have lots of heavy elements, like our Sun, was the first population of stars discovered: Population I. The second type of star we found, by examining globular clusters, is much poorer in heavy elements, and represent an entirely different population: Population II. In theory, there must have been stars with no heavy elements at all: Population III. That’s what we’re looking for!)
But what we fully suspect is that Population III stars are going to be incredibly high in mass, with an average mass of about 10 times (or 1000%) the mass of the Sun. Today, for comparison, the average star that’s born has just 40% of the mass of the Sun; the reason for the difference is that heavy elements — the ones made within stars — are what gas needs to radiate energy away, allowing it to cool and gravitationally collapse. Without these heavy elements, it’s up to the very inefficient and relatively rare hydrogen (H2) molecules to radiate the energy away, resulting in very large, massive gas clouds that collapse to produce very massive stars.
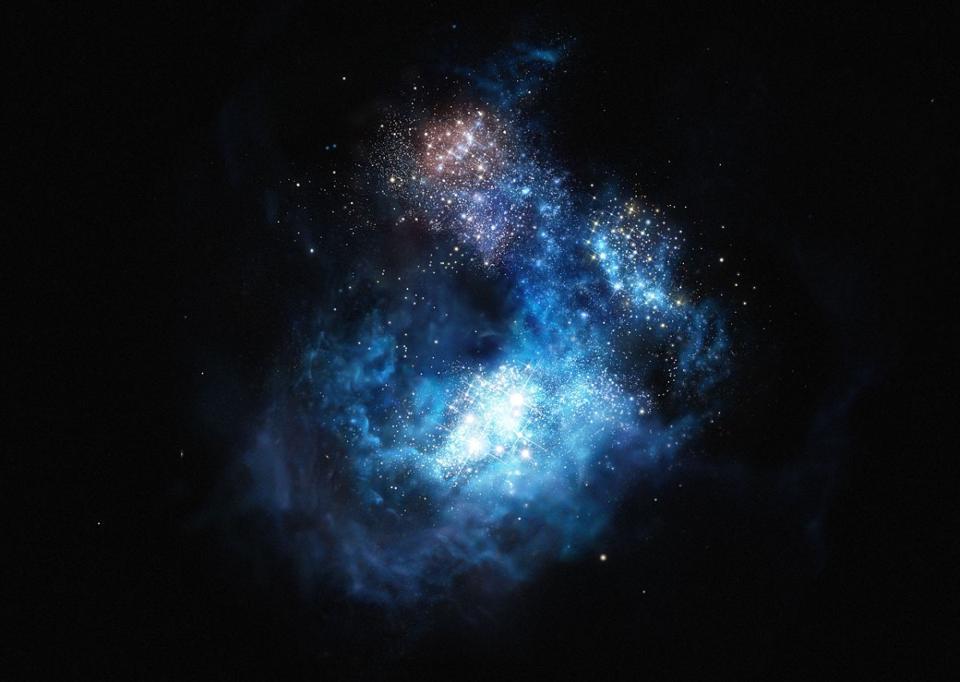
This is where the physics gets interesting. The more massive your star is, the brighter and bluer it is, the hotter its temperatures are, and, perhaps counterintuitively, the shorter its lifetime is, as it burns through its nuclear fuel far more quickly than its lower-mass counterparts. In other words, we expect that wherever we do form Population III stars, they should only exist for a very short time before the most massive among them die, significantly enriching the interstellar medium and giving rise to subsequent generations of stars that do contain heavy elements: Population II and even, after enough enrichment has occurred, Population I stars.
However, even though the very “first” stars that come into existence are made of this pristine, never-before-enriched material, those aren’t the only places that Population III stars should exist. In any location that has never been enriched by material that’s been ejected from prior generations of stars, pristine material should be what’s located there. Although we haven’t yet detected evidence for stars forming from such pristine material just yet, we have detected pristine material itself. In fact, the pristine material we found wasn’t from the first few million years of the Universe’s history, but rather was discovered 2 billion years after the Big Bang: found in a relatively isolated set of locations.
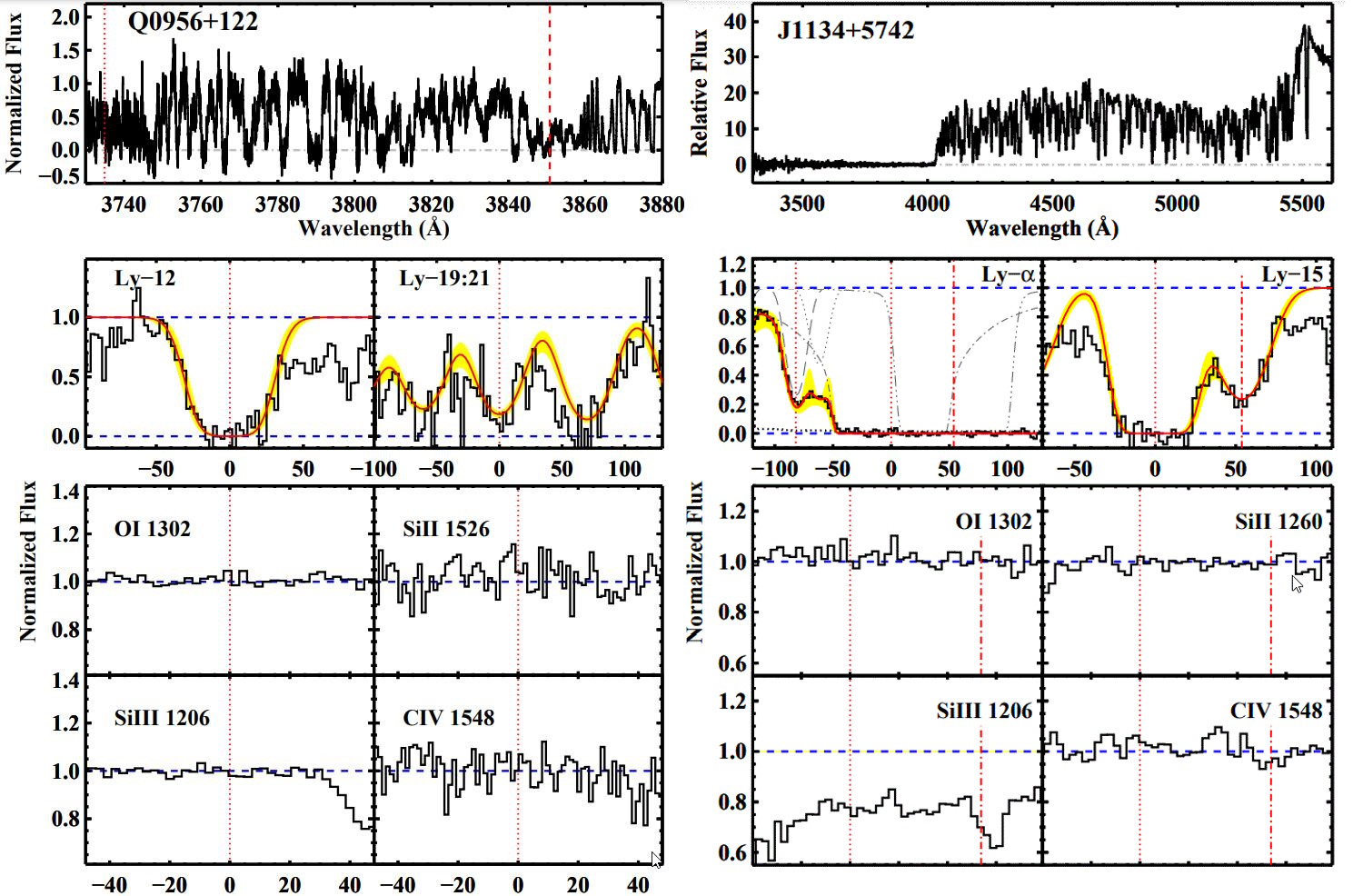
In order to detect a population of these early, most pristine stars, a clever scheme is needed. It’s easy to confuse yourself if you look for the wrong signatures, after all, as this is something astronomers have done before: fooling themselves specifically with a galaxy known as CR7. Initially, they were looking for He[II], or ionized helium, in the absence of any heavier elements, like oxygen and carbon. Although oxygen was indeed present, the authors claimed that there was evidence for a region of this galaxy that had no heavy elements but did have a strong helium signature: Population III stars alongside older, more enriched Population II stars. As a follow-up study with superior instrumentation definitively showed, no, there is no evidence for a pristine population of stars at all, anywhere within this galaxy.
Which brings us to the galaxy in question in this latest study: RXJ2129-z8HeII. At a redshift of 8.16, this corresponds to light that was emitted just 620 million years after the Big Bang. The authors do, in fact, detect the signature of ionized helium.
Unfortunately, they also detect both singly and doubly-ionized oxygen, and in great abundance. In fact, the intragalactic medium of gas within this galaxy is particularly rich in these heavy elements. In this particular galaxy, when the Universe was only 4.5% of its present age, the gas is already 12% as enriched as our modern-day Sun and Solar System are.
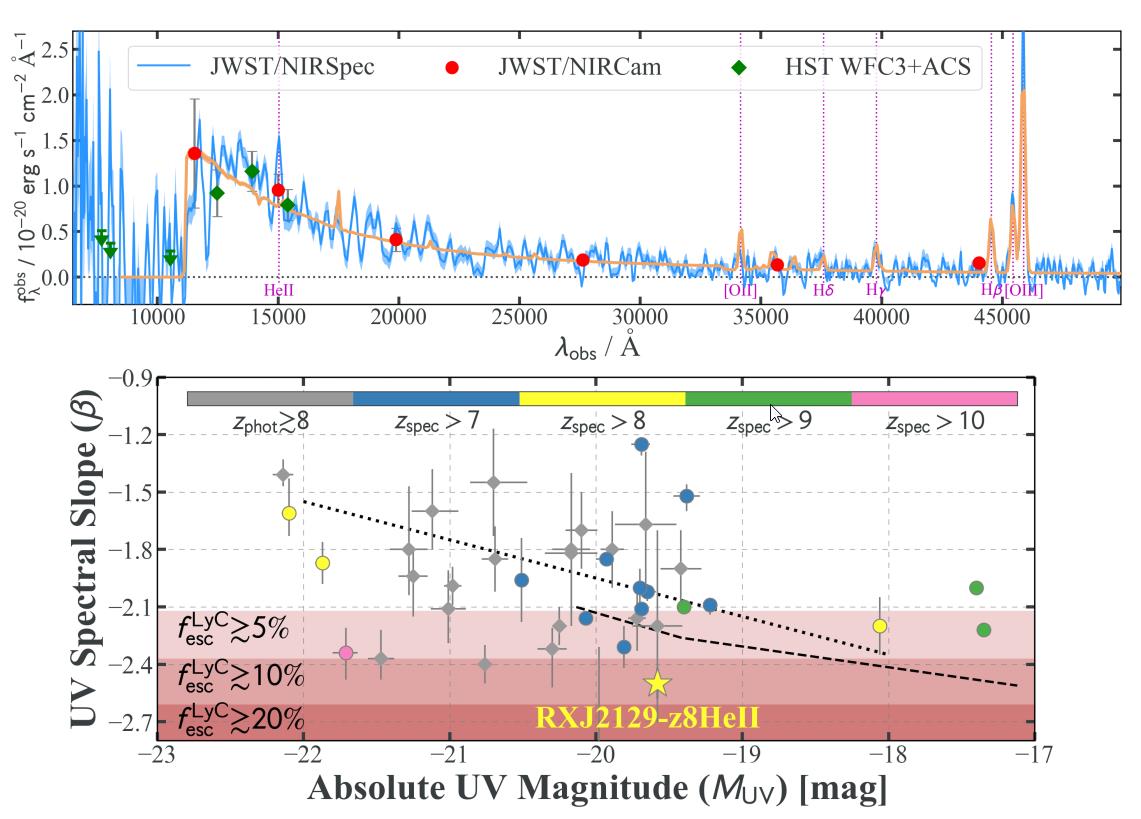
Again, despite a lack of evidence — all they can point toward is the mildly suggestive, severely blue tilt of the observed stellar spectrum — this team again resurrects the old idea that was discredited in the earlier galaxy CR7: that perhaps there is a population of pristine stars embedded within and appearing alongside the more-evolved Population II stars that are certainly present.
This is a teachable moment, because it’s precisely what “crying wolf” without actually seeing a wolf looks like in a scientific field such as astronomy.
Finding ionized helium, and everyone should know this, only indicates that you have helium present in your gas that’s been heated to temperatures about 12,000 K. To produce doubly-ionized oxygen, you need temperatures that are in excess of a figure that’s more like ~50,000 K. The fact that we see both, in great abundance, is a very strong hint that we have:
- many new, massive stars,
- a very bright, perhaps even starbursting galaxy,
- and the significant presence of both helium and oxygen within the galaxy.
There is no reliable evidence that any of the stars are made of pristine material; it’s pure conjecture. And that’s wildly insufficient to claim a discovery; you need robust evidence, not simply dubious evidence coupled with a healthy-but-uncritical imagination.
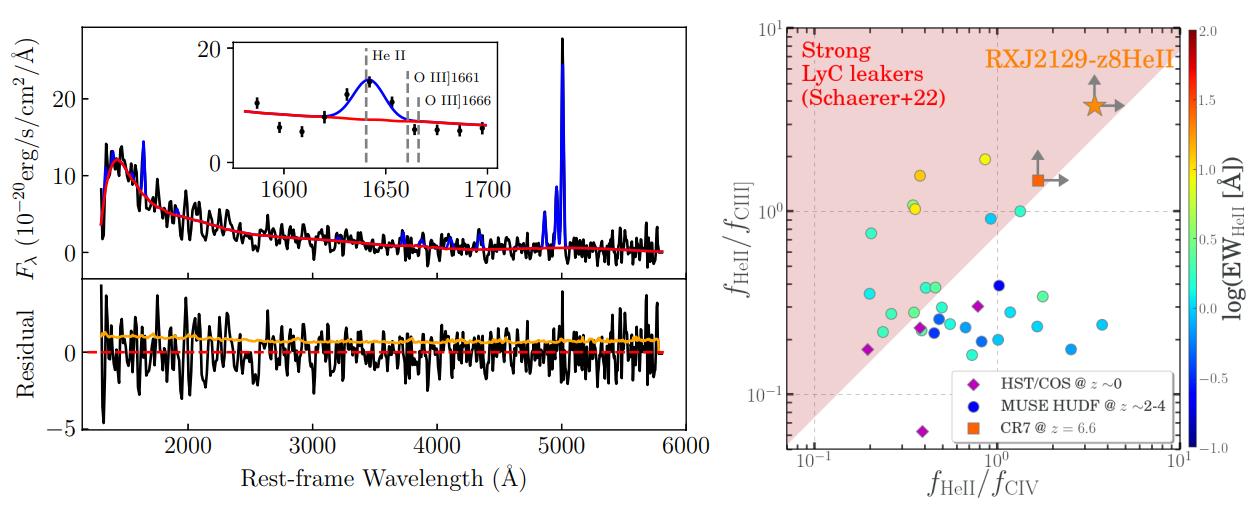
This is unfortunately typical of many groups of researchers caught up in the race to find something “new” for the first time: you can count on many of them reaching for glory before the arrival of convincing, compelling evidence. It’s grossly unacceptable, however, for any responsible journalist working with a vaunted science publication to put out such an error-riddled piece under the title of “Astronomers say they have spotted the Universe’s first stars.” The evidence isn’t there for that, and in the world of science, we don’t care about what anyone — no matter how famous or prestigious — says; we care about what is and isn’t true.
The fact that this is Quanta Magazine’s second high-profile botch-job (with the other on the topic of wormholes and quantum computers) in a two-month span should send alarm bells ringing through the world of science reporting. The moment we stop reporting what is true and instead report what any wolf-crying scientist out for their own vainglorious fame asserts, that’s the exact moment in which we’ve left all of our journalistic scruples behind.
The sober truth is that the first, pristine, Population III stars in the Universe are certainly out there, and there’s no compelling evidence that we’ve yet found them. Until we have something that’s unambiguous and robust — like ionized helium in the utter absence of any form of oxygen — we should all remain appropriately skeptical of this and any such claims. Getting the facts right about our own Universe depends on it.
Note: The Quanta Magazine story referenced in this article has been updated from its original version to correct the helium-2 error.