What was it like when the Sun was born?
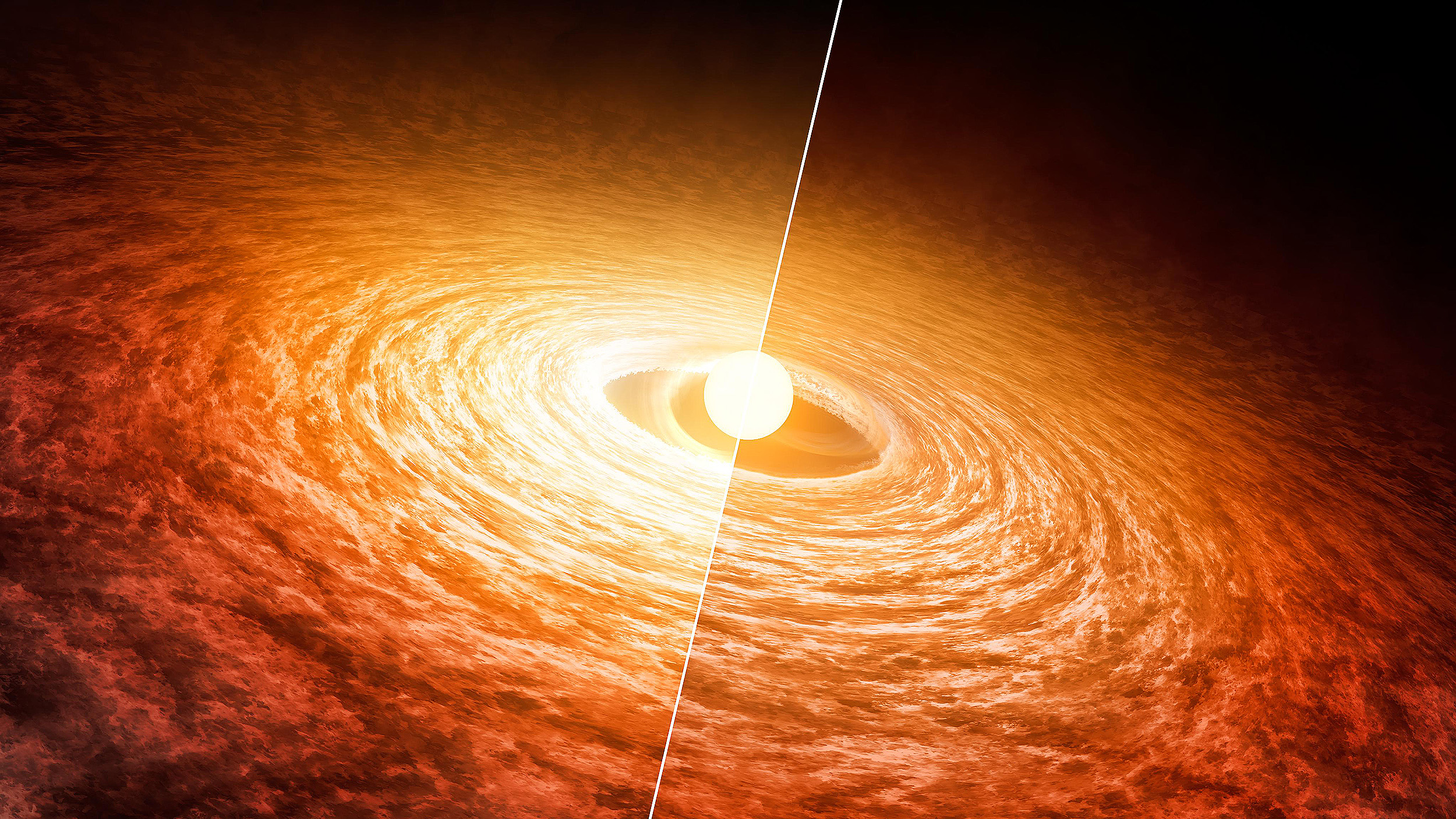
- About 4.6 billion years ago, in an unremarkable corner of a run-of-the-mill spiral galaxy, an event that occurred billions upon billions of times before took place: a new star, with its own stellar system, was born.
- Although most stars never see life arise within their stellar systems, our Sun would wind up providing the energy that gave rise to and sustained life on at least one of the bodies that orbit it: planet Earth.
- Today, a whopping 13.8 billion years after the Big Bang, humanity, a species that arose on that very planet, grew to prominence, and reconstructed our past cosmic history. Here’s what the Sun’s birth was like.
Although the Universe was only two-thirds of its present age back when the Sun was first forming, things back then were already very similar to how they are today. The Milky Way appeared relatively isolated: the second-largest member of a relatively modest group of galaxies. Low-mass dwarf galaxies would be seen slowly merging and being gobbled up by larger ones, just like they do all over the Universe. And throughout the Milky Way, hundreds of billions of stars are already shining, with gas clumps occasionally contracting along its spiral arms to trigger new waves of star-formation. There are anywhere from tens to hundreds of these regions active in our galaxy at any time.
This process goes on in all gas-rich quiescent galaxies across the Universe, including run-of-the-mill spiral galaxies like our own: stars form slowly, in little bursts, while gas gets drawn into the spiral arms and star-formation propagates outward from the center in these objects. In one of those regions within the Milky Way, 9.2 billion years after the Big Bang, a cloud of gas collapsed, giving rise to many new stars and likely a star cluster. It was from one of those clumps, that a particular star — our Sun — as well as the planets and the remainder of our Solar System, formed. Here’s what it was like when the Universe made what would eventually become our cosmic home.
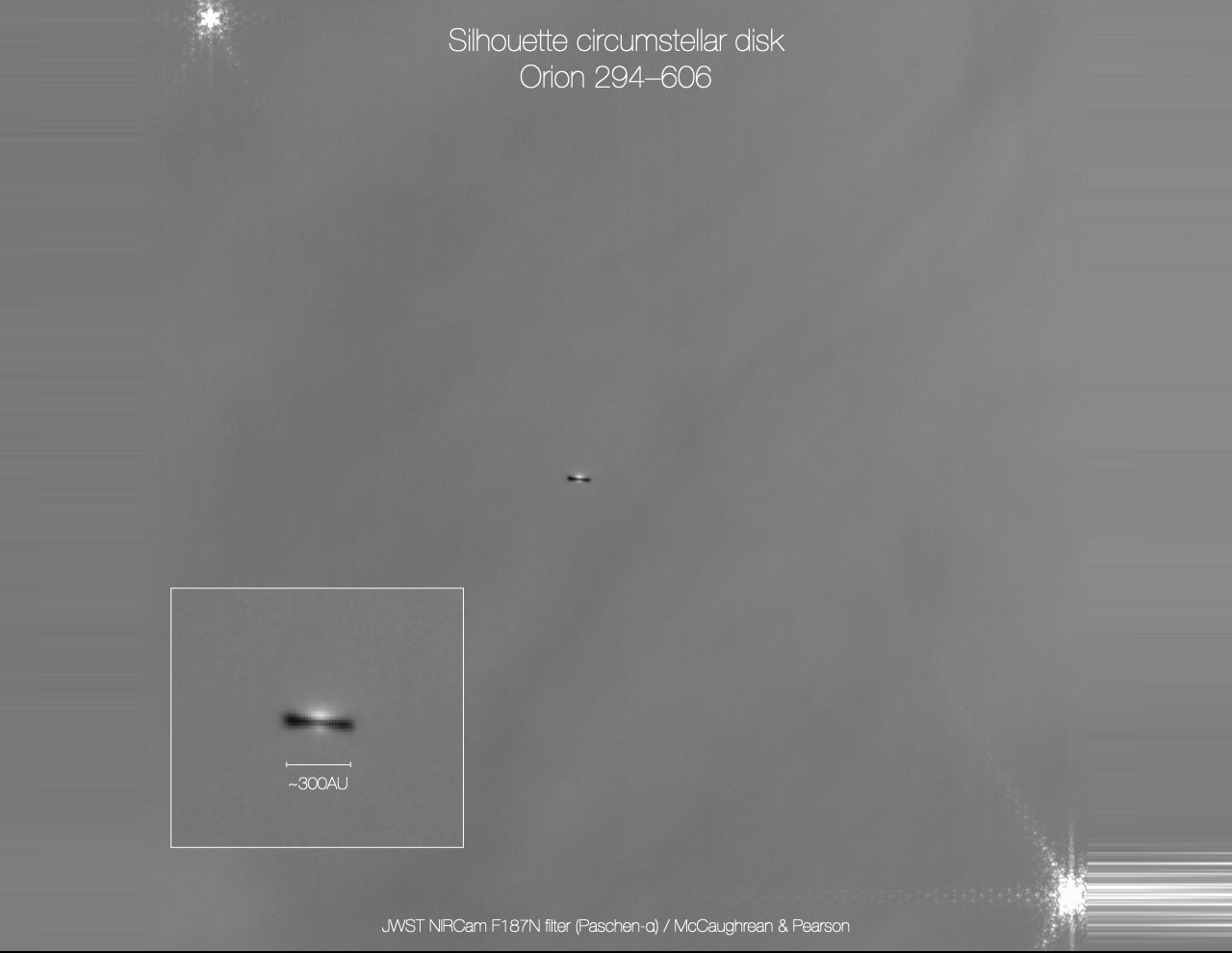
The story of star-formation has been occurring for nearly all of cosmic history: except for the first 50-100 million years, the Universe has always been forming stars somewhere, in some way. Gas clouds contract, undergo a process known as core fragmentation, and give rise to stars — about half of which form in singlet systems, while the other half form as members of multi-star systems — for over 99% of the Universe’s history. However, it took quite a bit of time and cosmic evolution before stellar systems like ours, with rocky planets, the ingredients for life, and potentially habitable conditions, became possible.
It took many generations of stars living and dying, burning through their fuel, going supernova, blowing off their outer layers, and having stellar remnant (white dwarf-white dwarf and neutron star-neutron star) collisions occur, just to fill our galaxy with the heavy elements we’d need for rocky planets and life. Unless a star forms with at least 8-25% of the Sun’s heavy elements, rocky planets may well be impossible: a condition still impacting stars in the galaxy’s outskirts and in the galactic halo. It was only once these raw ingredients were in place that our Solar System could form, with the potential to give rise to us. In order for us to exist with the properties that we came to possess, a whole slew of other things had to line up just right.

Spiral galaxies are shaped roughly like a pancake: the gas within them is in a thin disk that’s denser toward the center and less dense at the outskirts. As they rotate, the inner parts spin around a greater number of times than the outer parts; galaxies rotate differentially, rather than like a spinning record. Additionally, gas, dust, and other material tends to get funneled inward over time, drawn in from the galactic outskirts and halo toward the galactic center, where it participates in rounds of star-formation by mixing with the elements and material already present in the innermore regions.
These processes cause the heaviest elements to preferentially wind up located near the central regions, while the lighter elements wind up farther away from the galactic center. Our Solar System formed from a gas cloud about halfway toward the edge of the disk, about 27,000 light-years from the center, in the central part of the disk if you were to slice it lengthwise. When our Solar System first formed, we were made out of about 70% hydrogen and 28% helium, and only about 2% of everything else combined. Still, this represents coming a long way since the Big Bang, where everything was 75% hydrogen, 25% helium, and less than a millionth-of-a-percent everything else.
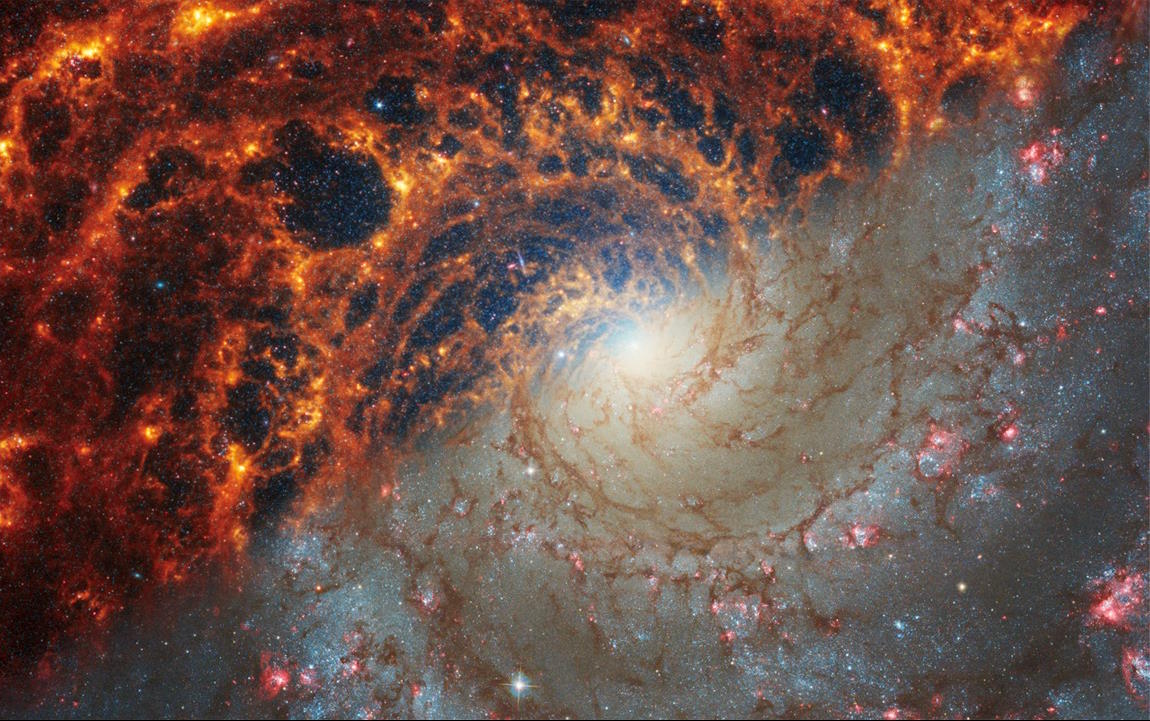
The way most stars form in galaxies like ours — i.e., in evolved spiral galaxies that are relatively quiet — is when clouds of gas in the disk pass through one of the spiral arms. Material gets funneled into these clouds, while density waves propagate through the galactic disk, causing that gas-and-dust to bunch up, achieving an even greater-than-average density than before. Once a critical density is achieved and the gas/dust can cool itself sufficiently fast by radiating heat away, gravitational collapse becomes an inevitability. When that collapse finally happens, these clouds of gas, which can range from thousands to millions of times the mass of the Sun, begin to fragment into hundreds, thousands, or even greater numbers of tiny clumps.
The largest clumps to first form begin attracting the most matter, and they grow into the largest, most massive stars: typically found toward the central, dense regions of these gas clouds. Smaller-magnitude clumps grow more slowly, and achieve lower masses, forming lower-mass stars and being more likely to have their infalling gas-and-dust blown away by external radiation from the more massive, brighter, hotter stars. Meanwhile, clumps that merge together will see their growth accelerate. Inside every star-forming region, a race occurs: between gravity, working to form and grow stars, and radiation, emitted by the hottest stars, working to evaporate that star-forming material away.
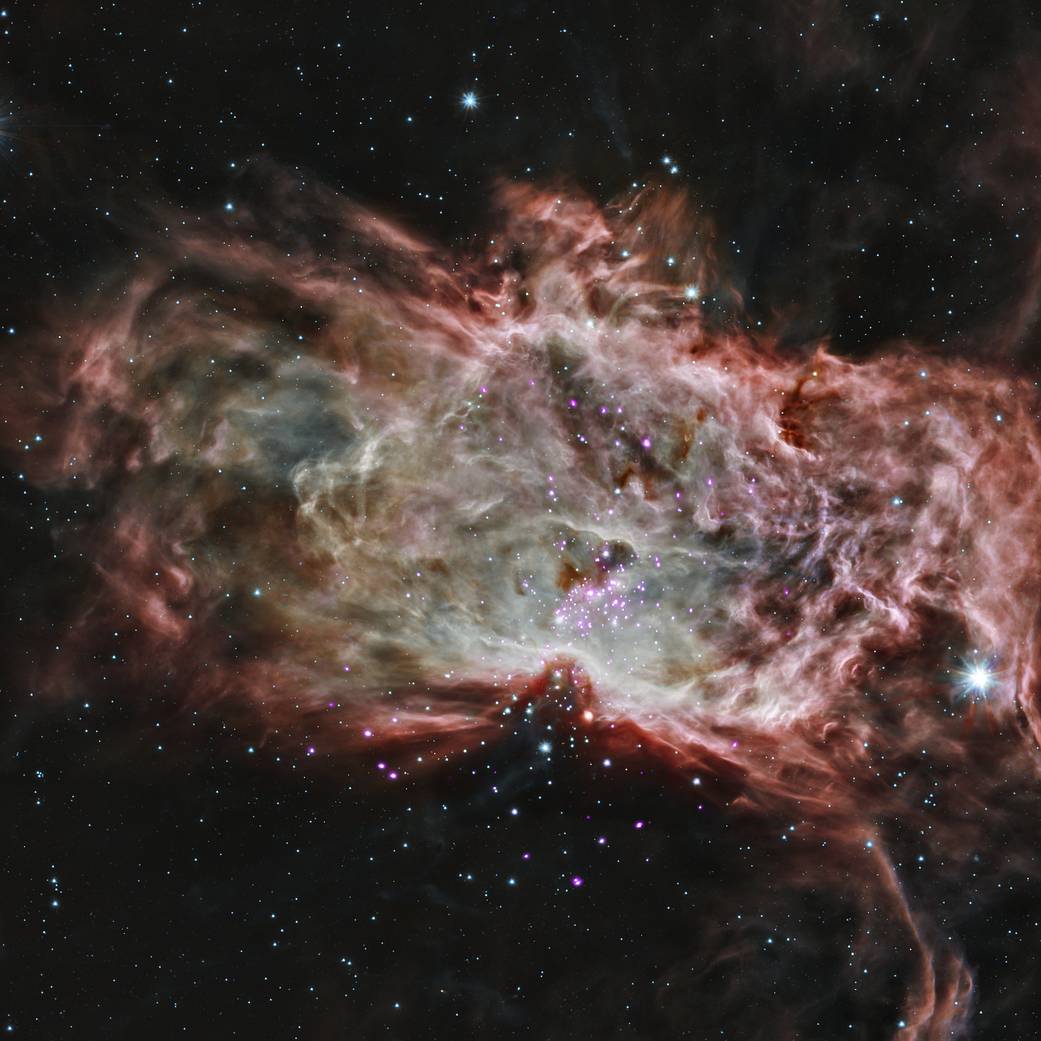
Over time, the Universe itself makes it clear who the big winners will be. You might think that it’s the most massive stars, which can reach masses that are tens or even hundreds of times as massive as our Sun, and can give off radiation thousands to millions of times as luminous as our own star. In terms of mass, temperature, brightness, and overall emitted energy, these are the grand winners. These behemoths will, in relatively short order, destroy the active star-forming regions they were born in by evaporating the surrounding gas away, preventing the lower-mass stars and protostars from growing beyond a certain point.
But for those stars — the most massive ones — the victory is short lived and Pyrrhic in an important sense. While the most massive stars wind up being the brightest, most luminous, and most easy to see, they’re also the fewest in number. Gravitation turns out to be a very tenacious beast, as it draws gas into a large variety of regions, including regions where the matter is much sparser than in the densest regions. While a large, star-forming nebula might form tens or even hundreds of high-mass stars, it’s going to form hundreds of low-mass stars for each high-mass star that forms. While the brightest, hottest, bluest stars get all of the attention early on, they’re mere flashes-in-the-pan on cosmic scales. After only a few million years pass by, they’ll all be gone.
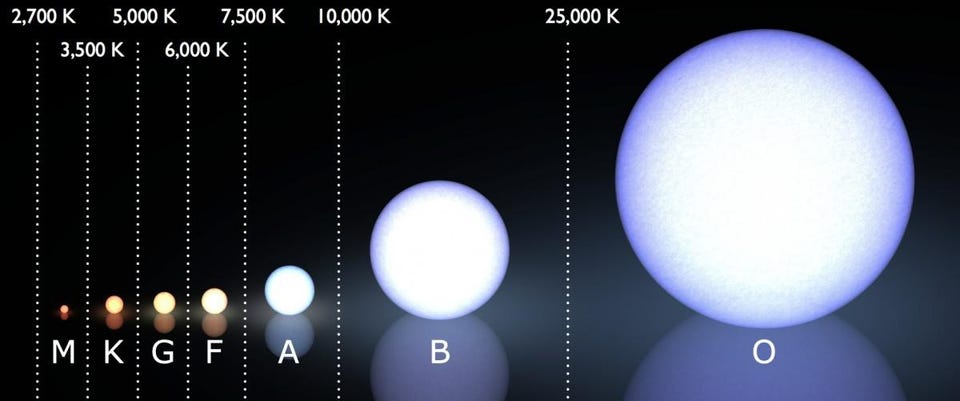
You might have heard the old saying that “the flame that burns twice as bright burns only half as long,” but for stars, the situation is far worse than that. A star that’s twice as massive as another burns through its fuel about eight times as quickly, and one that’s ten times as massive as another will live less than 1% as long as the lower-mass star. Compared to a star like our Sun, which might last for 10-12 billion years, a star that’s tens or even hundreds of times as massive as our Sun will reach the end of its life after only a few million years have gone by, at most. And unlike the Sun, which will die gently in a planetary nebula, the most massive stars will die in violent stellar cataclysms, such as core-collapse supernovae.
Early on in the star-forming episode that gave rise to our Solar System, the protostar that would become our Sun was still pulling in matter, growing, and working to collapse down to form a central star orbited by a disk of matter: a circumstellar disk from which planets and more would eventually arise. Meanwhile, the most massive stars around it furiously burn through their fuel, exploding in supernovae events, and putting an end to star-formation in any surrounding environments where they successfully evaporate all of the gas away. The Universe is a violent place, and star-forming regions are some of the most violent places of all: a mix of birth and death on mind-boggling scales.
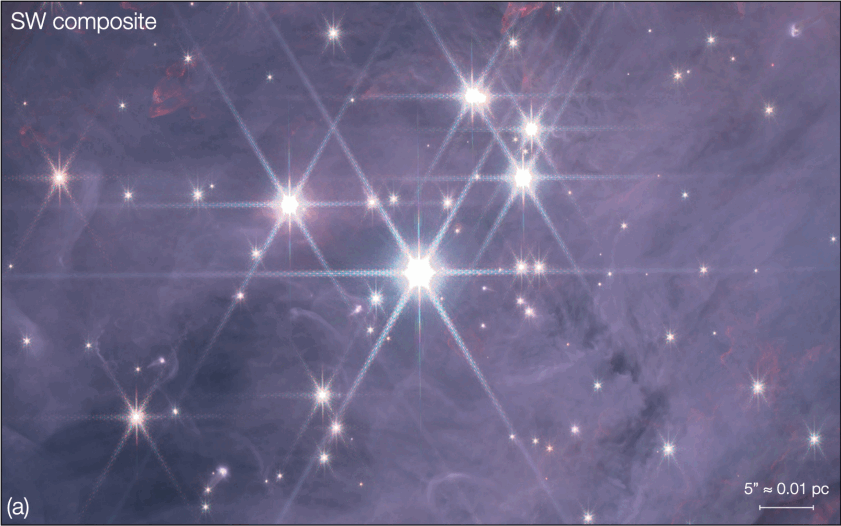
When we look at our Sun in comparison to all the other stars that form in the Universe, we’re a far cry from the most massive ones of all, which have weighed in at up to 260 times the present mass of the Sun. However, when we compare our Sun to the low-mass end of the stars we find, we learn we aren’t exactly among the least massive stars, either. The central clump of matter that will grow into our Sun started out larger, earlier, and grew faster than the vast majority of clumps that are present. If we were to take a look at our Sun, today, and compare it to all the other stars in the Universe, here’s a surprising fact about it: it’s more massive than 95% of all the stars that are out there.
In fact, somewhere between 75% and 80% of all stars are red dwarf (M-class) stars: the lowest-mass, coolest, and smallest class of star out there, with no more than around 40% of the Sun’s mass. Of the rest of the stars, more than half of them are the next class up: K-class, which is still smaller, less massive, and cooler than our Sun (from about 40-80% the Sun’s mass). The amount of matter that clumped together to lead to our parent star was above average in terms of mass, but was typical in one very important fashion: we were alone, without any other stellar companions in our system.
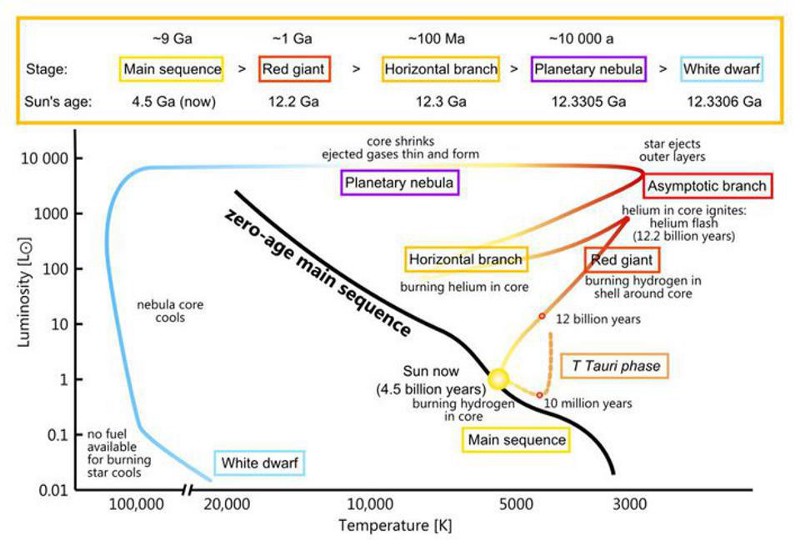
In most of the large star-forming regions we find in Milky Way-sized galaxies, thousands of new stars are born. Of these, many of them will be bound together in multi-star systems, while approximately half of them, total, will be single stars without another stellar companion. We learned this relatively recently, by looking at the nearby stars to Earth, thanks to a collaboration known as RECONS. The REsearch Consortium On Nearby Stars (RECONS) surveyed all the stars they could find within 25 parsecs (about 81 light-years), and discovered 2,959 stars total. Of those, 1533 were single star systems, but the remaining 1426 were bound into binary, trinary, or even more complex systems.
We long wondered why our Sun is a single-star system, rather than a multi-star system. After all, it seems like it could have been born in almost any configuration, so why did we arise in this one? While it might seem like pure chance, it turns out that we’ve now discovered more than 5000 exoplanets, and rocky planets that exist in what we presently call the “habitable zone” around their stars — with the right conditions for liquid water on their surfaces given Earth-like atmospheric pressures — are practically non-existent in multi-star systems. The reason our Sun is a singlet star, perhaps, is because singlet star systems are by far the most likely to house potentially habitable worlds: something we absolutely required if we were going to arise within this Universe.
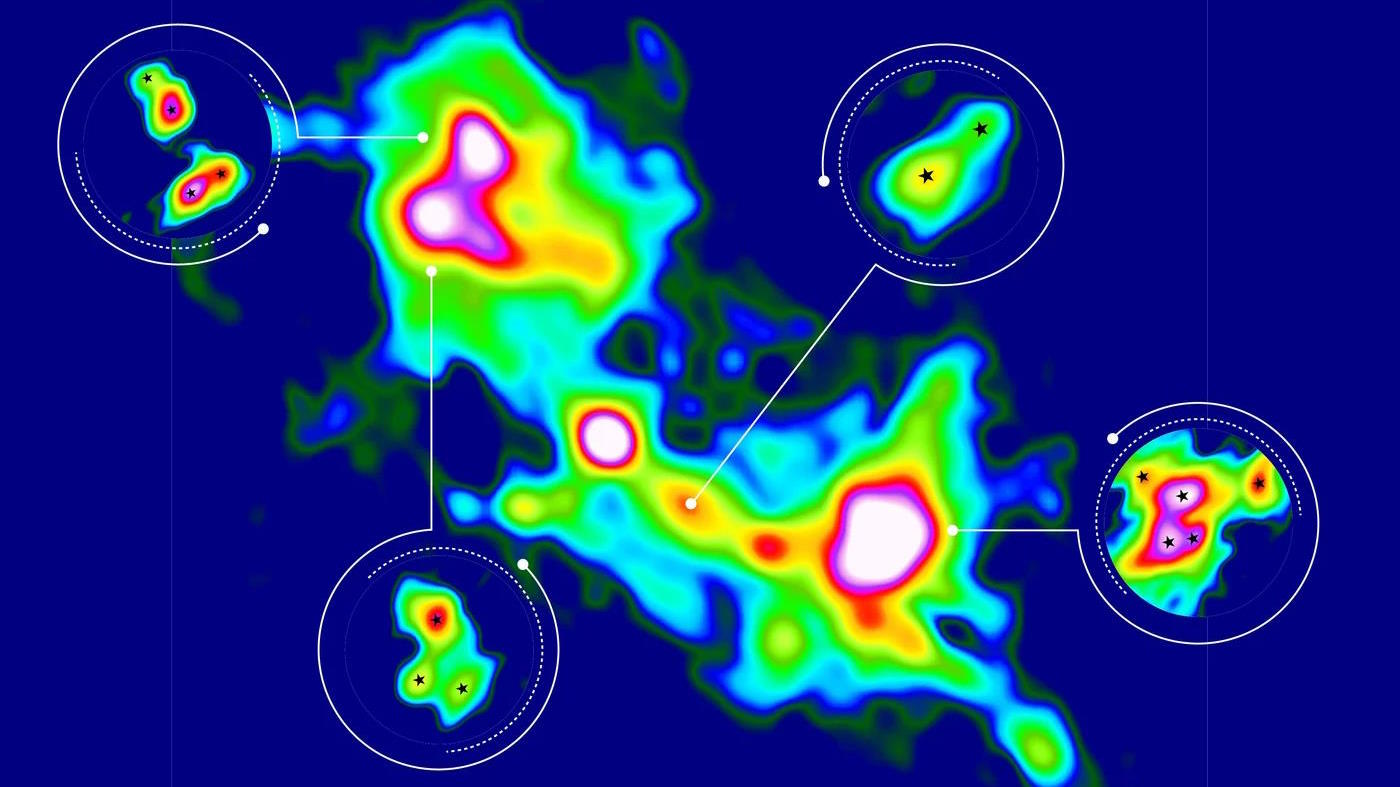
As the years ticked by, the fragment of the gas cloud that turned into our Sun accrued matter largely onto a central clump, forming a protostar. The particles within that protostar radiate heat away, allowing it to collapse and become denser, while gravitational collapse simultaneously causes the temperature to rise and rise in the innermost central core of this clump. At some point, a critical threshold is reached: a temperature of 4 million K, which is the point at which individual protons can begin to fuse into heavier elements through the process of nuclear fusion via the proton-proton chain.
This marks the moment that our star — the Sun — is officially considered to have been “born.” To the best of our knowledge, this moment happened 4.56 billion years ago, when the Universe was approximately ⅔ of its current age. The central star ignites, and that triggers a violent set of downstream effects.
- The matter surrounding the protostar, distributed in a disk, begins to evaporate away from the inside-out due to stellar radiation and winds.
- The circumstellar disk, after only 500,000 to 2,000,000 years, begins to fragment and form clumps: the first protoplanets.
- And various volatiles are boiled away, creating a series of “frost lines” where various forms of ice can no longer persist in regions interior to that line.
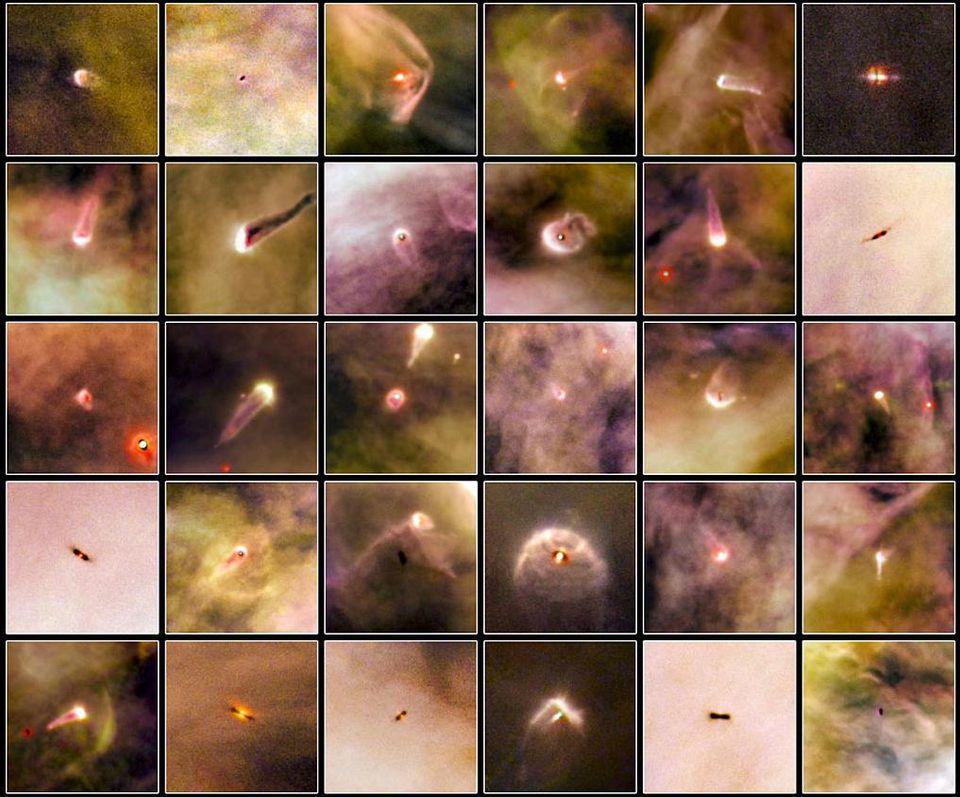
This is a remarkable story we’re finally able to tell, as it’s only in recent years that we’ve been able to observe stellar systems in these very early stages of formation, finding central stars and protostars shrouded by gas, dust, and often protoplanetary disks with gaps in them. These are the seeds of what will become giant and rocky planets, leading to full-on stellar and planetary systems like our own. Although most of the stars that form — including, very likely our own — will have formed amidst thousands of others in massive star clusters, there are a few outliers that form in relative isolation.
Either way, the star clusters that form rarely persist for long, with nearly all clusters ejecting their members and dissociating completely in under 1 billion years. Although the history of the Universe may subsequently separate us from all of our stellar and planetary siblings from the nebula that they formed in billions of years ago, scattering them across the galaxy, our shared history remains. Whenever we find a star with approximately the same age and abundance of heavy elements as our Sun, we cannot help but wonder: is this one of our long-lost siblings? There are likely thousands of them roaming the galaxy, and perhaps someday, we may be able to identify our cosmic brothers-and-sisters that grew up in the same nursery that we did all those billions of years ago.