What was it like at the beginning of the Big Bang?
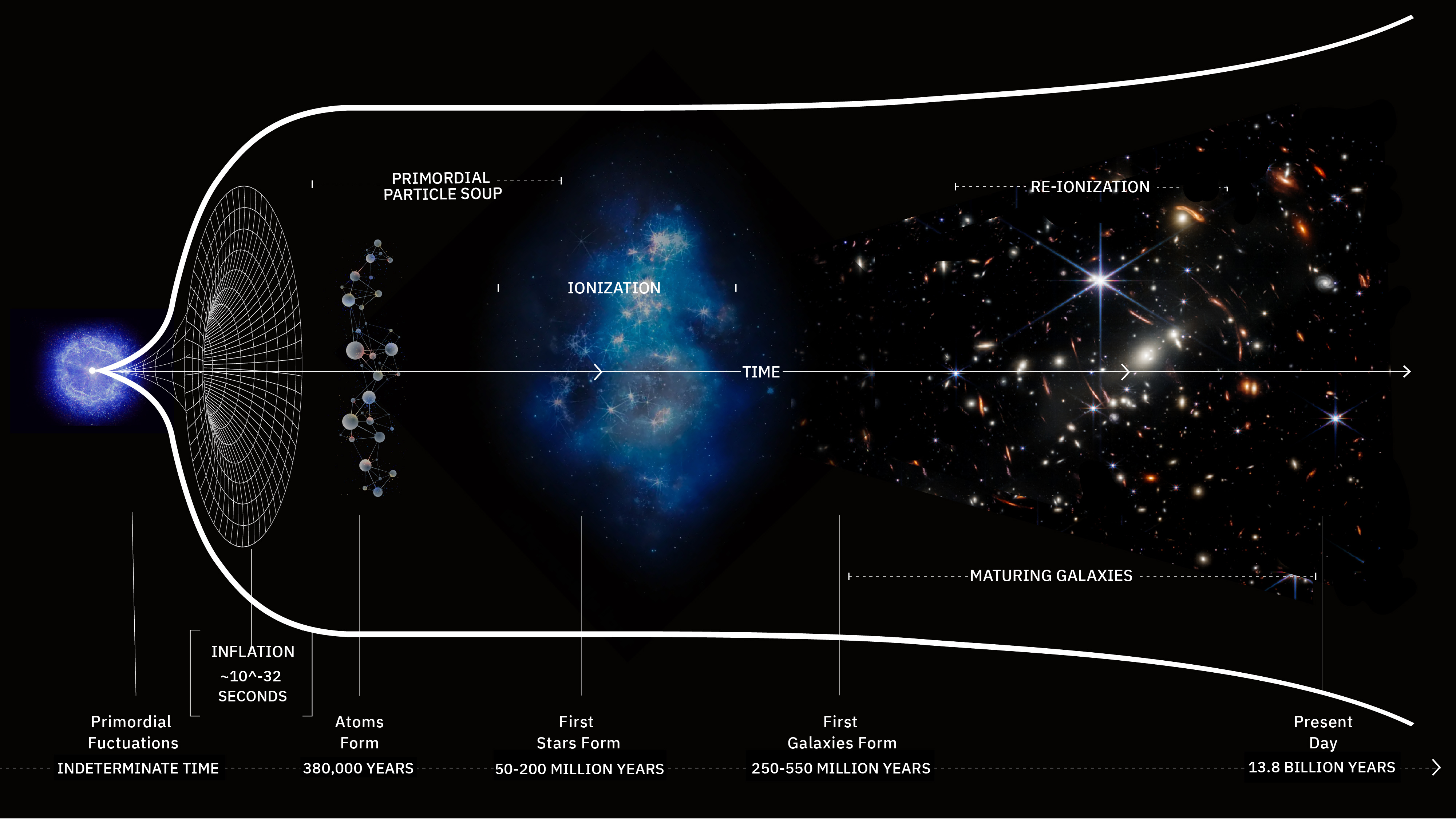
Looking out at our Universe today, we not only see a huge variety of stars and galaxies both nearby and far away but also a curious relationship: the farther away a distant galaxy is, the faster it appears to move away from us. This continues as far as we’ve ever looked, and remains true on average for all galaxies: the farther away they are, the greater their observed redshift (corresponding to recession) is. In cosmic terms, the Universe is expanding, with all the galaxies and clusters of galaxies getting more distant from one another over time. In the past, therefore, the Universe was hotter and denser, and everything in it was closer together.
Imagine what this means if the Universe is, and has always been, expanding: not just for the future, but for our cosmic past as well. If we extrapolate back as far as possible, we’d come to a time:
- before the first galaxies formed,
- before the first stars ignited,
- before neutral atoms,
- or atomic nuclei,
- or even stable matter,
could exist. The earliest moment at which we can describe our Universe as hot, dense, and uniformly full of stuff is known as the Big Bang. Here’s the story of how it first began.
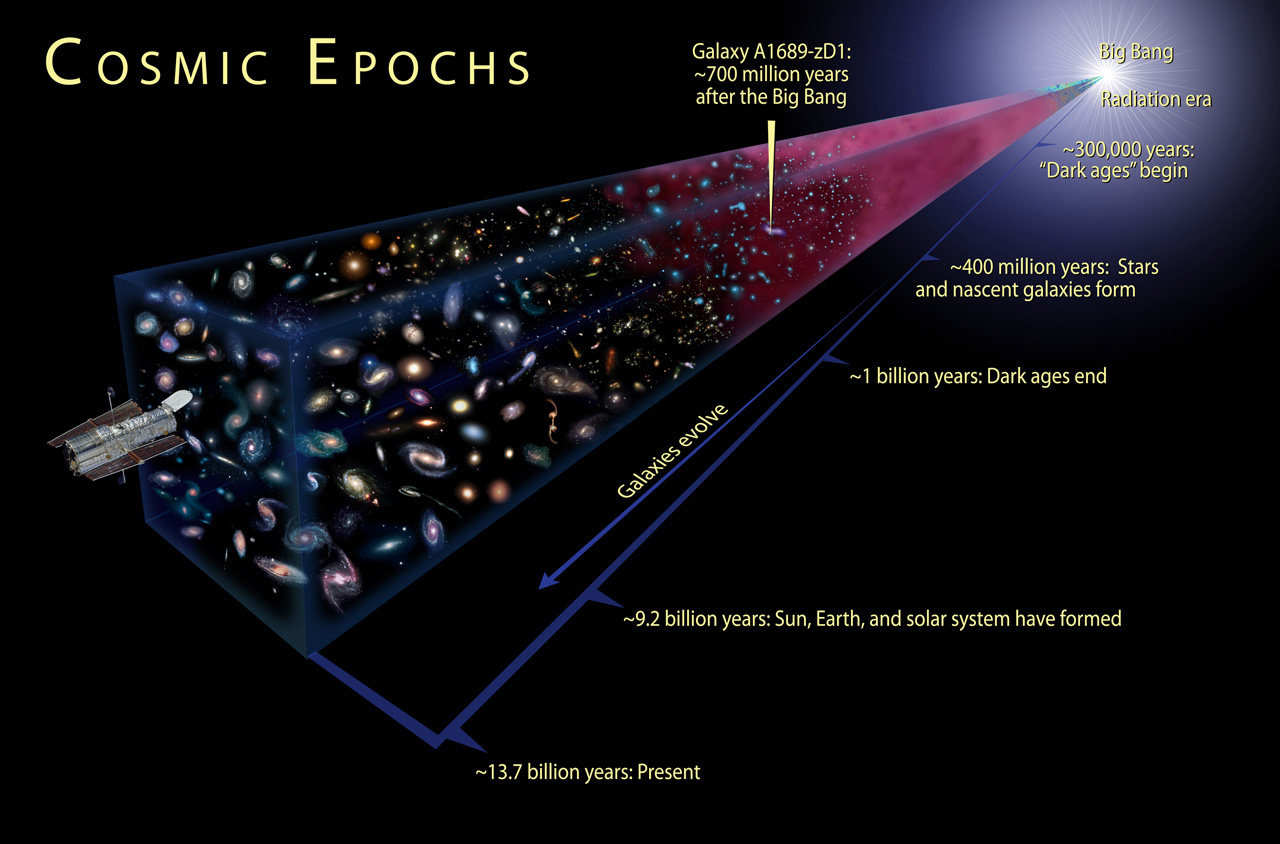
Some of you are going to read that last sentence and be confused. You might ask, “Isn’t the Big Bang the birth of time and space?” And this is a view that many modern cosmologists can be sympathetic to, as at one point in the history of cosmology, that’s how the Big Bang was originally conceived. Take something that’s expanding and of a certain size and age today, and you can go back to a time when it was arbitrarily small and dense. When you get down to a single point, where all the matter and energy in the Universe come together at once, that event corresponds to what we know as a singularity: a point from which space and time originally emerge.
But we know that’s not correct today, in 2024. In fact, there’s a ton of evidence that points to a non-singular origin of our Universe. We never achieved those arbitrarily high temperatures; there’s a cutoff. Instead, our Universe is best described by an inflationary period that occurred prior to the Big Bang, and the Big Bang is the aftermath of what occurred at the end of inflation.
Let’s walk through what that looked like.
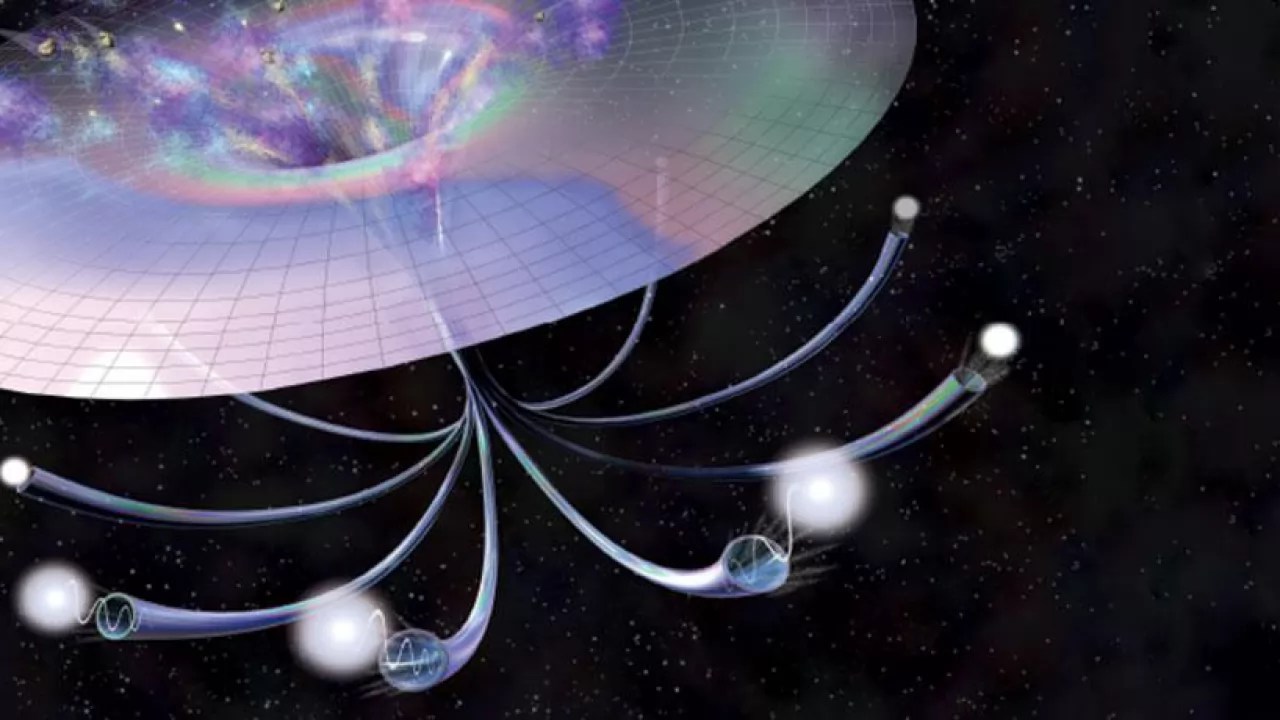
During inflation, the Universe was completely empty. There were no particles, no matter, no photons; just empty space itself. That empty space had a huge amount of energy in it at every location, with the exact amount of energy slightly fluctuating over time: by about 1 part in 30,000, on average.
As the Universe inflates, expanding in a rapid, relentless fashion, those fluctuations get stretched to larger scales, while new, small-scale fluctuations are created atop them. This superposition of fluctuations, of small-scales atop intermediate-scales atop large-scales atop superhorizon-scales, is one of the defining predictive features of cosmic inflation. (We described what the Universe looked like during inflation previously.)
This continues as long as inflation goes on. But inflation will come to an end randomly, and not in all locations at once. In fact, if you lived in an inflating Universe, you’d likely experience a nearby region where inflation came to an end, while the space between you and it expanded exponentially. For a brief instant, you might even be able to detect what happens at the start of a Big Bang, before that region disappeared entirely from view.

In an initially, relatively small region, perhaps no bigger than a human-sized hamster ball (but perhaps much larger), the energy inherent to space gets converted into matter and radiation. The conversion process is relatively fast, taking approximately ~10-33 seconds or so: a brief amount of time, but nonetheless one that is not instantaneous. As the energy bound up in space itself gets converted into particles, antiparticles, photons, and more, the temperature starts to rapidly rise: from just a few degrees above absolute zero to perhaps ~1020 K or so, over that same brief time interval..
Because the amount of energy that gets converted is so large, everything will be moving close to the speed of light. All quanta will behave as radiation with so much kinetic energy inherent to them, regardless of whether the particles are massless or massive; it doesn’t matter under these conditions. This conversion process is known as reheating, and signifies when inflation comes to an end and the stage known as the hot Big Bang begins.

In terms of the expansion speed, you’ll witness a tremendous change from all prior behavior when the hot Big Bang first commences.
In an inflationary Universe, space expands exponentially, with more distant regions accelerating away relentlessly as time goes on. But when inflation ends, the Universe reheats, and the hot Big Bang starts, more distant regions will now recede from you more and more slowly as time goes on.
From an outside perspective, the part of the Universe where inflation ends sees the expansion rate there drop, while the inflating regions surrounding it see no such drop. Under inflation, the distance to any object would double after a certain amount of time, and once that same amount of time elapses, that distance doubles yet again, and again, and again. The process is relentless. But once the Big Bang begins, all of that changes, as the expanding Universe immediately slows down once the first moment of expansion elapses.
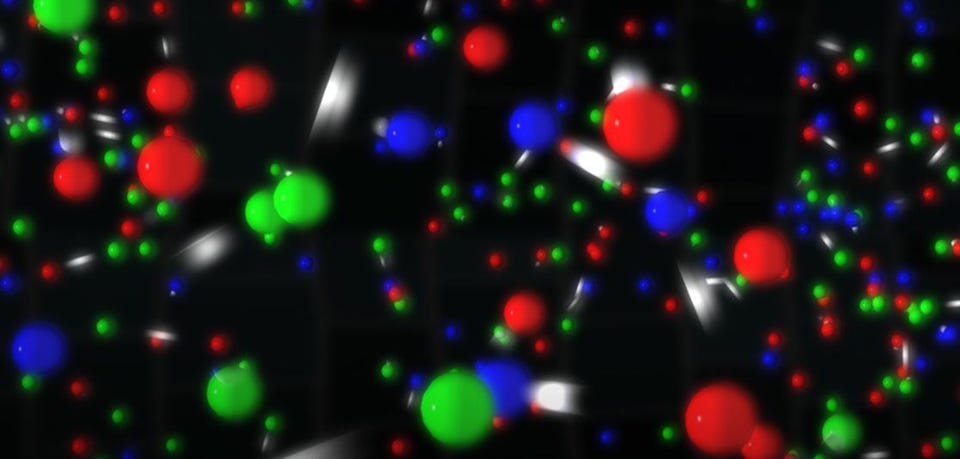
Probability-wise, it’s extremely likely that from the perspective of whatever region of inflating space you’re in prior to the Big Bang, you’ll experience inflation ending in nearby regions many times. These locations where inflation ends will quickly fill with matter, antimatter, and radiation, and expand more slowly than the still-inflating regions do, leaving you — in the inflating region — as a “typical” region within spacetime, dominating its volume.
These regions, where hot Big Bangs occur, will expand away from all the other locations where inflation still goes on exponentially, meaning they will very quickly recede from one another’s view. In the standard inflationary picture, because of this expansion rate change, there’s virtually no chance that any two Universes, where separate hot Big Bangs occur, will ever collide or interact.
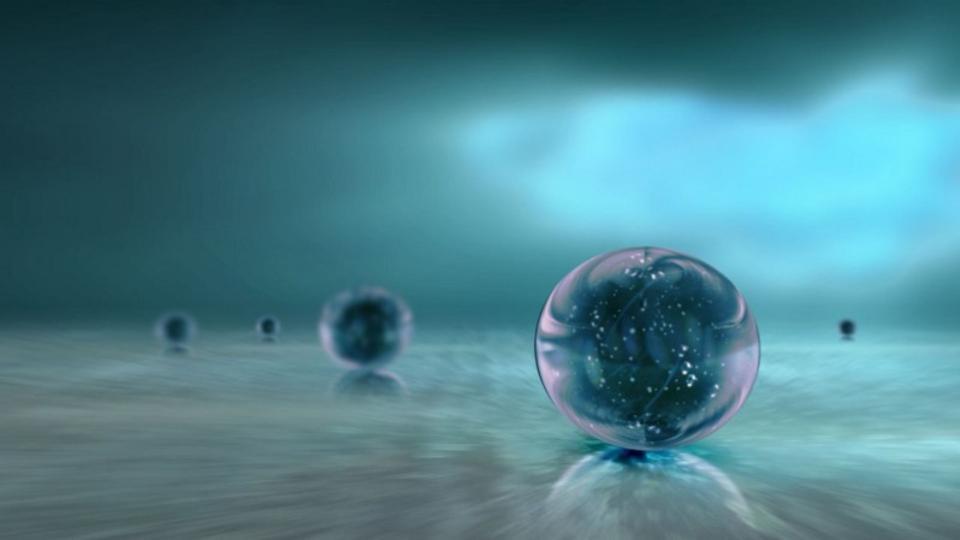
Finally, the region where we will come to live gets cosmically lucky, and inflation comes to an end for us. The energy that was inherent to space itself gets converted to a hot, dense, and almost uniform sea of particles. The only imperfections, and the only departures from uniformity, correspond to the quantum fluctuations that existed (and were stretched across the Universe) during inflation.
The positive-energy quantum fluctuations will correspond to initially overdense regions, while the negative-energy fluctuations get converted into initially underdense regions. The typical difference might only be at the ~0.003% level, but that’s still enough to serve as the eventual seeds of cosmic structure.
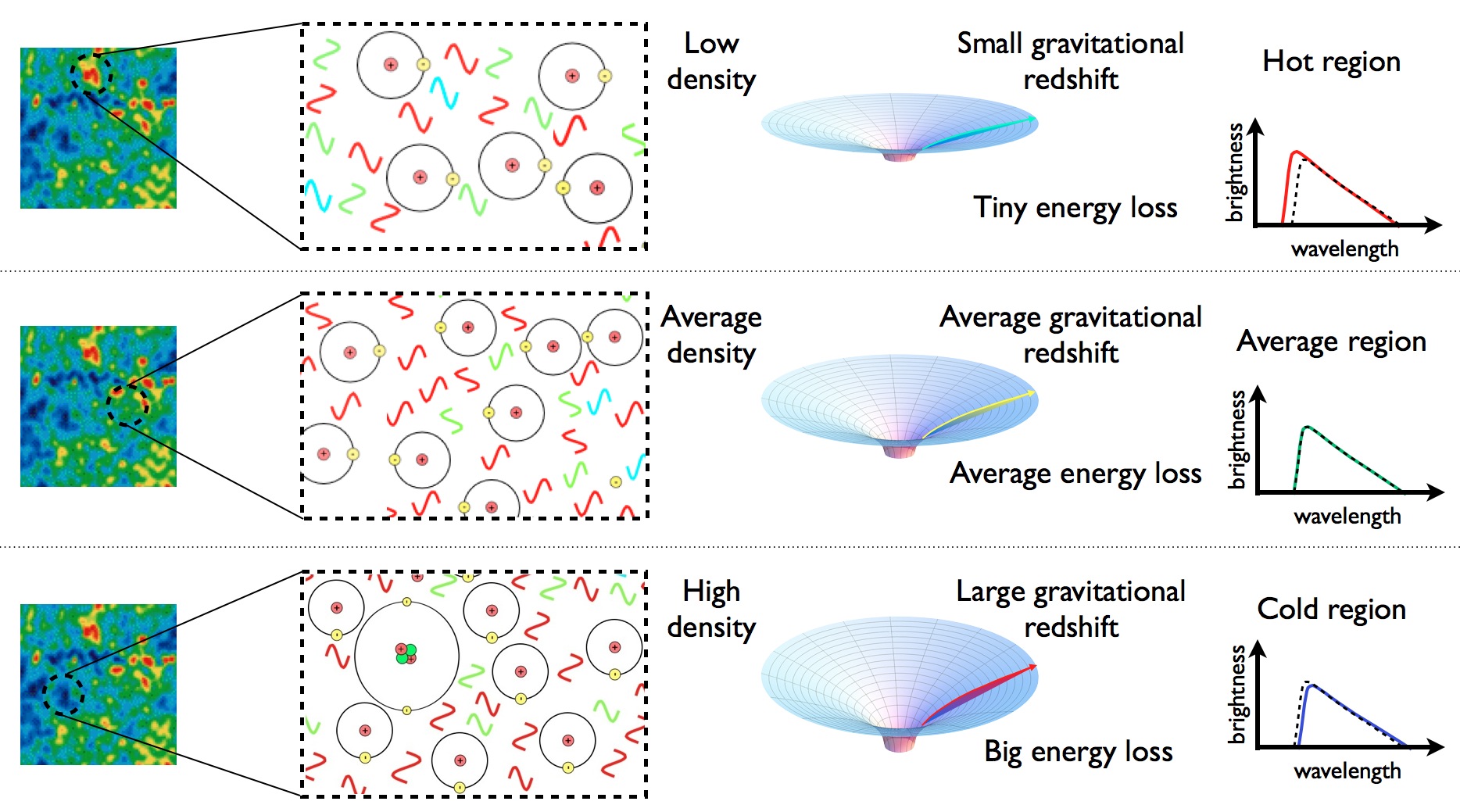
We cannot observe these density fluctuations, today, as they were when the Universe first underwent the hot Big Bang. There are no visual signatures we can access from that early on; the first one we’ve ever accessed comes from 380,000 years later, after they’ve undergone countless interactions.
Even at that, we can extrapolate back what the initial density fluctuations were, and find something extremely consistent with the story of cosmic inflation. The temperature fluctuations that are imprinted on the first picture of the Universe — the cosmic microwave background — gives us confirmation of how the Big Bang began.
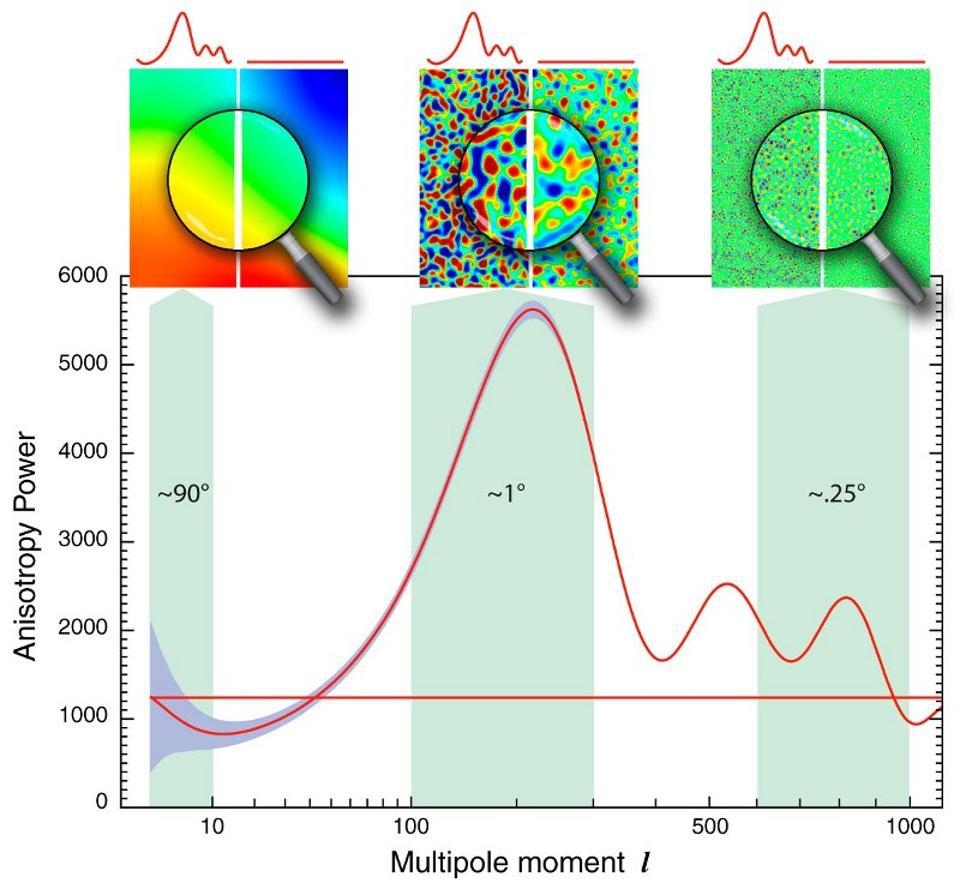
What might someday be observable to us, however, are the gravitational waves left over from the end of inflation and the start of the hot Big Bang. The gravitational waves that inflation generates move at the speed of light in all directions, but unlike the visual signatures, no interactions ever slow them down.
They will wash over us, arriving continuously, from all directions, passing through our bodies and our detectors. All we need to do, if we want to understand how our Universe got its start, is find a way to observe these waves either directly or indirectly. While many ideas and experiments abound, none have returned a successful detection so far. We know what the spectrum of these fluctuations will look like and what imprint they’ll have on the light within our Universe, but we have no idea what their magnitude is. Different models of inflation make different predictions, and only by (eventually) measuring them can we determine which model(s) accurately describe our Universe.

Once inflation comes to an end, and all the energy that was inherent to space itself gets converted into particles, antiparticles, photons, etc., all the Universe can do is expand and cool. Everything smashes into one another, sometimes creating new particle/antiparticle pairs, sometimes annihilating pairs back into photons or other particles, but always dropping in energy as the Universe expands.
The Universe never reaches infinitely high temperatures or densities, but still attains energies that are perhaps a trillion times greater than anything the LHC can ever produce. The tiny seed overdensities and underdensities will eventually grow into the cosmic web of stars and galaxies that exist today. 13.8 billion years ago, the Universe as-we-know-it had its beginning. The rest is our cosmic history.