Ask Ethan: What were the “dark ages” of the Universe?
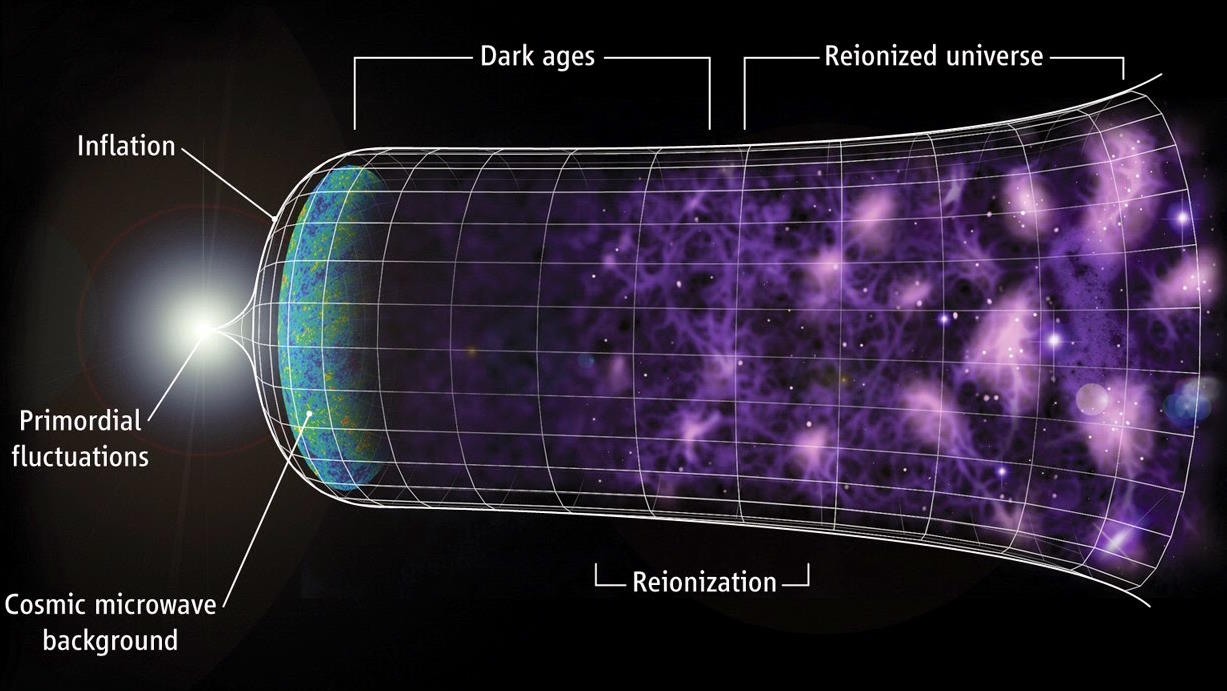
- When we look out at the Universe today, in every direction we observe, there are stars and galaxies lighting up the vast expanse of the cosmos.
- But beyond a certain point, even with the power of JWST, there are no stars, galaxies, or any other luminous sources of light to behold.
- That in-between time, after the Big Bang but before there are visible stars and galaxies, is called the “dark ages” of the Universe’s history. Here’s what they were really like, plus why.
Today, in all directions, no matter where we look, there are luminous sources of energy to behold. Stars, galaxies, nebulae, and even energy-emitting black holes populate the Universe wherever matter has clumped and clustered together sufficiently. Even though there are great cosmic voids that span up to around a billion light-years in diameter, they’re merely holes in the cosmic “Swiss cheese” of structure. From all directions, the light still gets in, and illuminates even the darkest corners of the Universe.
But that’s what things are like now, 13.8 billion years after the Big Bang. As we look deeper and deeper into the Universe, we see that the story gradually begins to change. Past a certain threshold, galaxies appear redder and fainter than expected: as though something were in the way, blocking that light. That effect gets more severe with distance, where only the brightest of galaxies can be perceived at all. At last, we run out of light to see, suggesting that there were “dark ages” beyond a certain point. What were those dark ages like? That’s what Predrag Branković wants to know, asking:
“How was the dark age of the universe really dark?”
The darkness was real, but there are actually three things at play, all together, that caused them. Here’s how to understand the dark ages, and why they finally came to an end.
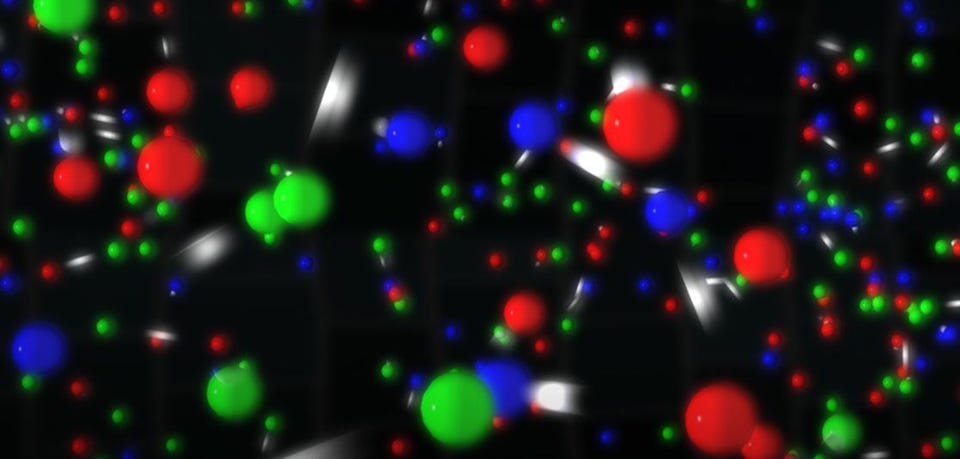
Initial light fades away
Back at the start of the Universe as we know it — during the earliest stages of the hot Big Bang — everything was brilliantly hot and dense. Not only was the Universe filled with quanta of light, photons of terrifyingly high energies, but all the other particles (and antiparticles) that the laws of physics allowed to come into existence. Given that:
- energies were tremendous, possibly as high as trillions of times what the Large Hadron Collider at CERN can achieve,
- conditions were very dense, causing enormous numbers of high-energy collisions to occur at every instant,
- and that any particles or sets of particles/antiparticles that could be created would have come into existence as a result of those collisions, so long as they obeyed Einstein’s E = mc²,
a hot, dense, energetic “primordial soup” of particles (and antiparticle) must have been what existed back then: in the beginning stages of the Universe.
But this hot, dense Universe is also expanding very rapidly, which causes it to cool. The reason is simple: photons (and all massless particles) have a wavelength, and even massive particles have a wavelength associated with them, and the size of that wavelength determines the particle’s energy. As the Universe expands, the stretching of cosmic length scales causes these wavelengths to be stretched as well, to longer and longer values. Longer wavelengths mean lower energies, and so as the Universe expands, it also cools.
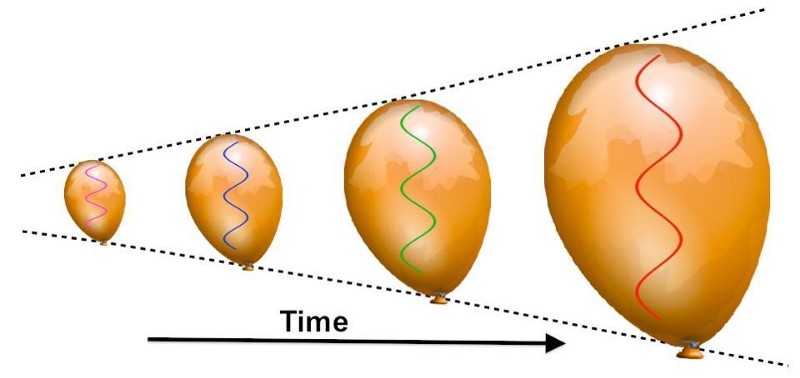
In the initial stages, practically all of the photons that existed were at extraordinarily high energies: in the gamma-ray portion of the spectrum. But as the Universe continues to expand (and cool) over time, the energy inherent to everything drops.
The heavier particles and antiparticles can still annihilate away, but it gets more difficult to create them via E = mc², since there’s less energy in each particle to have a chance of creating them.
The unstable particles and antiparticles, as the Universe expands and collisions/interactions become less frequent, begin to radioactively decay into lighter, more stable particles.
Reactions that couldn’t stably occur at higher energies — like protons and neutrons fusing into heavier elements, or electrons binding onto atomic nuclei to make neutral atoms — now occur, with the former taking place at ~a few minutes after the hot Big Bang and the latter occurring a few hundred thousand years after the hot Big Bang.
At last, the Universe, some ~380,000 years after the cosmic story began, is filled with neutral atoms, and the light left over from the Big Bang has cooled down tremendously: to about ~3000 K, with the photons making this bath of radiation up following a blackbody spectrum in their energy distribution.
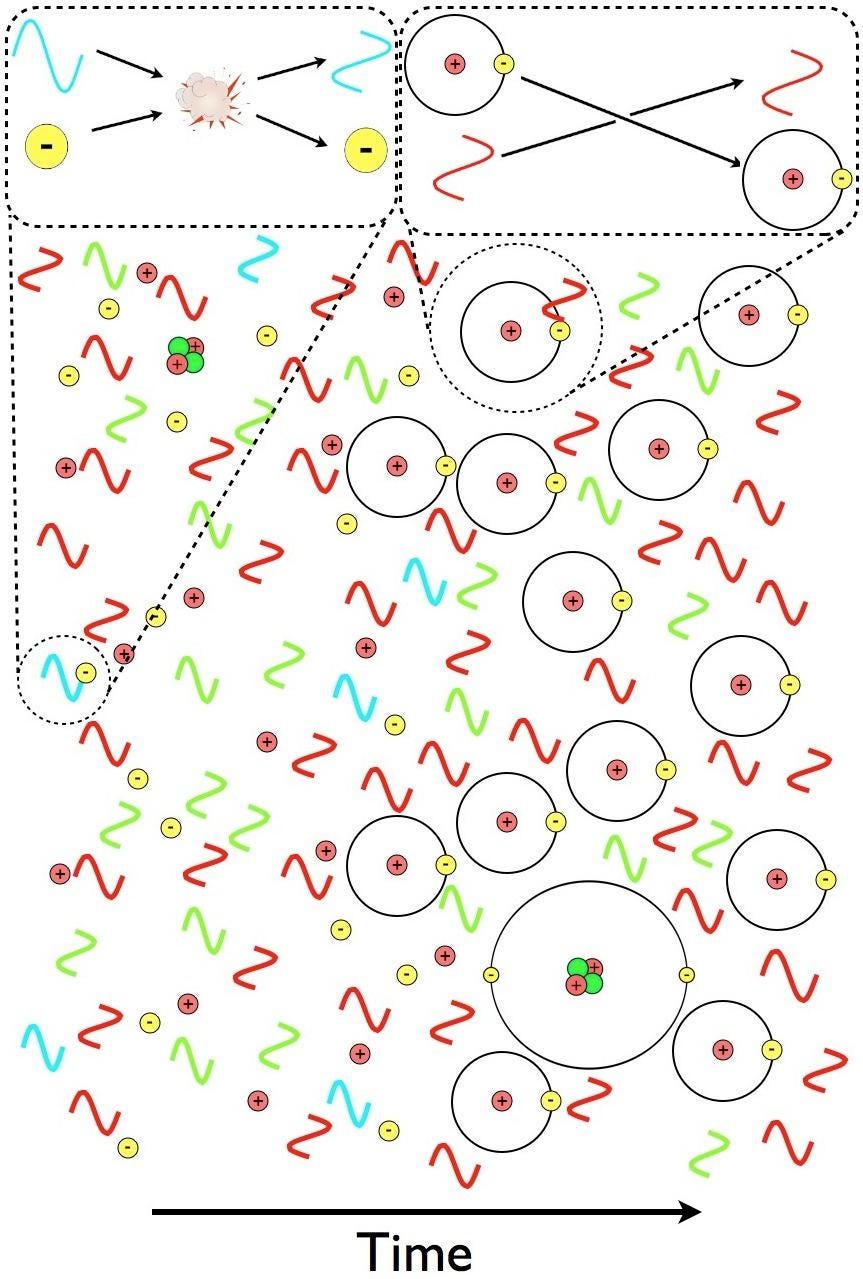
So all of this radiation still exists, and it’s luminous: ~3000 K would appear as bright red visible light to human eyes (if there were any humans or human eyes around back then), but the Universe is still expanding and cooling. As the Universe continues to age, it:
- expands,
- cools,
- and gravitates,
where those gravitational effects will eventually pull matter into sufficiently large clumps that stars can form. However, that will take time: considerably longer time periods than it takes for the leftover radiation from the Big Bang to continue to cool down past the threshold of being visible to human eyes.
Just as heated materials will glow red, but will fail to glow red if they’re below a certain temperature, this blackbody radiation left over from the Big Bang will cease to be visible after the wavelength has lengthened by a specific amount. As the glow of the Big Bang fades away, the last appreciable amount of photons leave the visible spectrum when the Universe is a little more than 3 million years old: about 3.62 million years, to be precise. Once it reaches that point, the Universe has entered the dark ages.
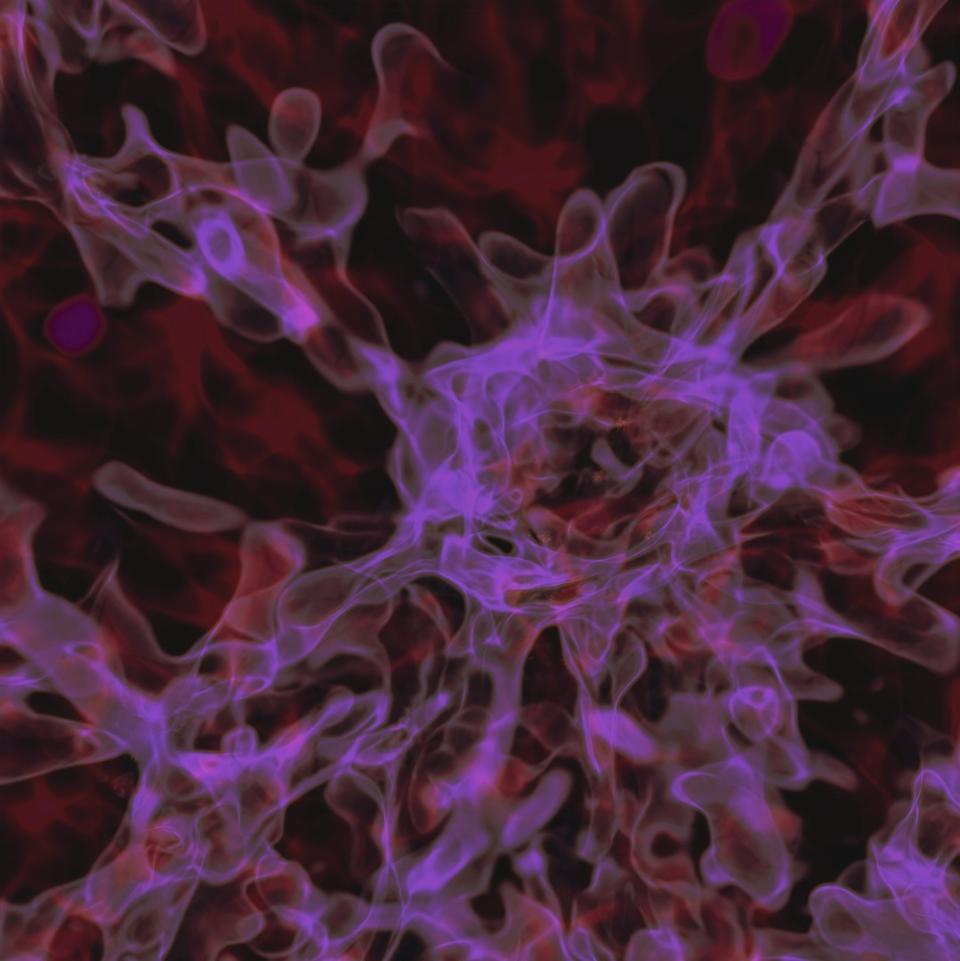
It takes time to form stars
Before any stars form, there will still be reactions inside atoms and between atoms occurring, and while those reactions will produce light, it won’t be visible light, but rather radio waves. The biggest culprit here is the humble hydrogen atom: the most common element in the Universe. If you were to take every atom that exists in the Universe at this time and count it, you would find that about 92% of all of your atoms were plain, normal hydrogen: with a proton for its nucleus and with one electron orbiting it. About 8% of the atoms would be helium-4, a few hundredths of a percent would be helium-3 and deuterium (hydrogen-2), and about one atom in a billion would be lithium-7. Nothing else, at this early epoch, yet exists.
But when hydrogen forms, containing both a proton and an electron, there’s a 50/50 chance that the quantum spins of those particles — the proton and electron — will be aligned, or facing in the same orientation as one another, and a 50/50 chance that they’ll be anti-aligned, or facing in opposite directions from one another. If they happen to form anti-aligned: great, that’s the lowest energy state, and no further transition will occur. But if they form aligned, with a half-life of about ~9 million years, they’ll spontaneously transition to the anti-aligned state, emitting a single photon in the process.
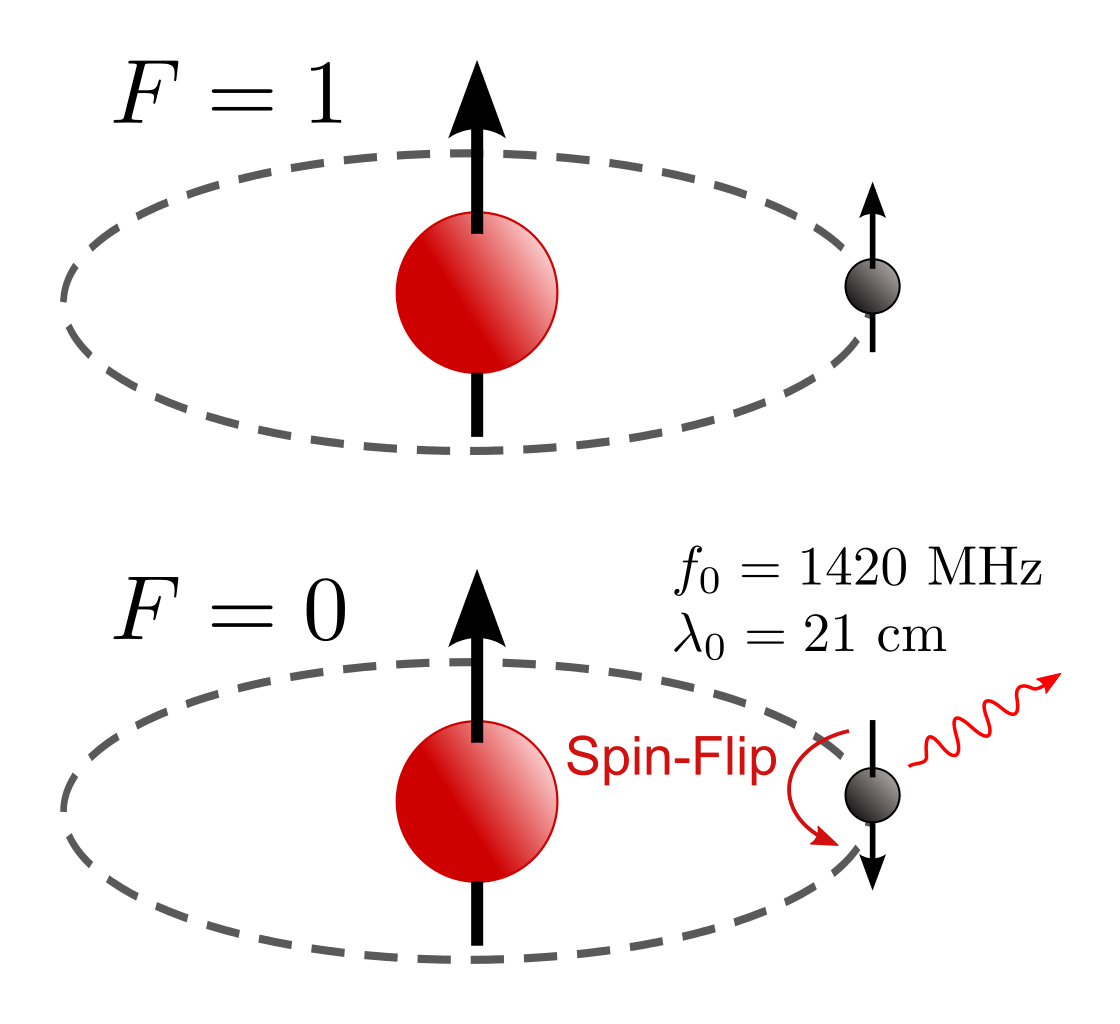
That transition, known as the spin-flip transition of hydrogen, will produce a photon of approximately 21 centimeters in wavelength every time. This happens to every proton and electron that spontaneously form a neutral hydrogen atom at any point: 50% of them will form in the spin-aligned state, and then those atoms will eventually all undergo this spin-flip transition, emitting long-wavelength photons in the process. However, because these photons are too long in wavelength to fall into the visible light portion of the spectrum, the Universe will remain dark.
We’re going to have to wait until stars form, until clumps of matter in the Universe get dense enough to start emitting their own light — first a little bit via gravitational contraction and then a lot from nuclear fusion — before there’s any way to “light up” this darkness. According to our best, highest-resolution simulations, the very, very first proto-stars ought to begin forming when the Universe is between about 50 and 100 million years old (at a redshift between z ~ 30-50), where nuclear fusion should ignite in their cores.
But, as the very first stars form, the Universe still remains dark, as all of those neutral atoms formed back when the Universe was just 380,000 years old now serve a second, less desirable purpose. In the dense regions that surround these newly-forming stars, they’ve combined to form molecular gas, and that neutral matter absorbs and blocks the starlight, keeping the Universe dark.
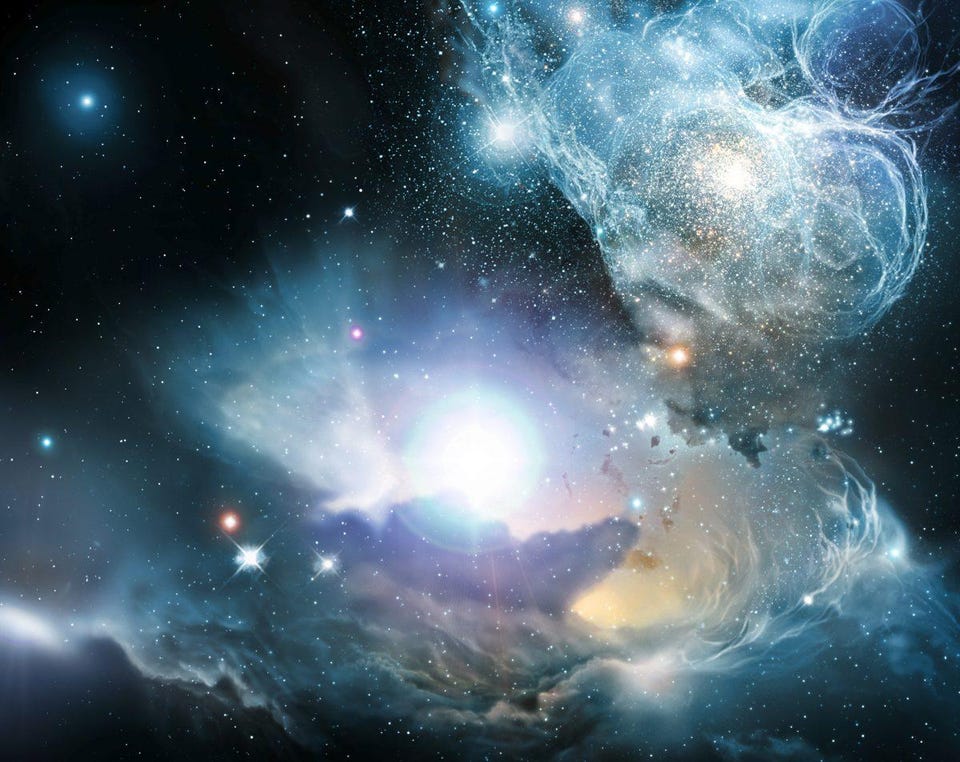
Light-blocking matter needs to be “boiled” away
This is the big problem now: all of those neutral atoms that we formed so long ago are now very effective at absorbing the starlight that’s being produced. Even though the first stars should be:
- made exclusively of hydrogen and helium,
- very high in mass, about 25 times the mass of the “average” star that forms today,
- extremely hot, with surface temperatures between 20,000-100,000 K,
- incredibly rich in their production of ionizing, ultraviolet radiation,
- and very short lived, dying in cataclysmic explosions after only a few million years,
there is so much neutral matter compared to the small number of stars that form early on that their radiation cannot penetrate very far. After traveling only a few thousand light-years, at most, it’s been entirely absorbed — or, as astronomers say, “extincted” — by the intervening neutral matter.
But there’s a little bit of hope here! When ultraviolet photons strike these intervening neutral atoms, the atoms absorb the light, but at the cost of becoming ionized themselves. In other words, even though there are a tremendous number of neutral atoms in the Universe initially — somewhere around a whopping ~1080 of them, give or take a few — at this late of a stage in the expanding Universe, once you ionize a neutral atom, the “electron” and the “nucleus” it was kicked off from are unlikely to recombine (either with the originals or with another nucleus or electron that’s been ionized) and form another neutral atom further down the road.
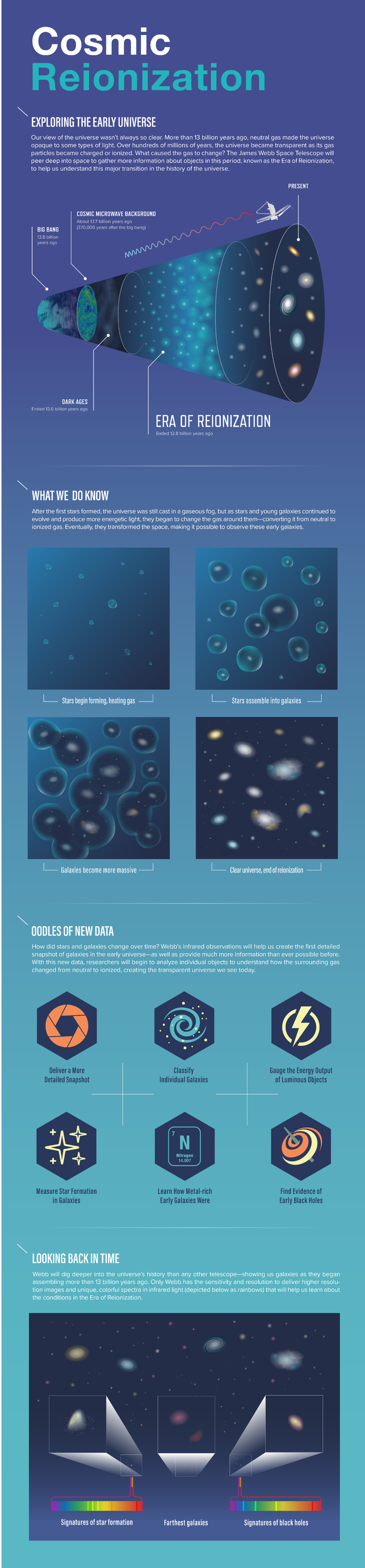
This means that all we have to do is wait for enough stars to form in enough regions of space, cumulatively, to emit sufficient amounts of ionizing, ultraviolet radiation in order to eliminate these neutral atoms, and to turn them into ions: with free electrons and bare atomic nuclei. These atoms, which began as an ionized plasma and only became neutral 380,000 years after the Big Bang, must become reionized in order for the starlight to break free. As a result, we call this process “reionization,” and it’s only when it successfully finishes that we’ll state that the dark ages have come to an end.
Although this process begins when the Universe is very young, it is a gradual process that takes a very long time to complete. According to the best measurements we can make, a typical region in space only becomes fully reionized after some ~550 million years have passed, but becomes “mostly” reionized, where 90% or more of the atoms in their vicinity have been converted into ions, a couple hundred million years earlier. Some regions will get serendipitously reionized a little bit earlier, while others will take longer than average; variations can be a few hundred million years, in general. But it’s only when all of the neutral, light-blocking matter is gone that we can say, “The dark ages have come to an end.”
At last: the darkness ends
Although we have simulations, like the one shown above, to show us how the Universe behaves on average, we have to look to the Universe itself to actually measure how much light gets absorbed along each various line-of-sight that we look at. When Hubble discovered what was (at the time) the farthest galaxy ever, GN-z11, astronomers found that even though its light was coming to us from only ~400 million years after the Big Bang, there was only a very small amount of light-blocking neutral matter in front of it. In other words, this was one of those serendipitously “greater than average” regions, where reionization happened faster than normal.
All of the remaining earliest galaxies discovered, including all the ones seen by JWST, are behind a thicker veil of light-blocking, neutral atoms. The earlier back in time we look, the more difficult it is to see them, and there can be no doubt that even with its longer-wavelength sensitivity and superior light-gathering power, there are no doubt many galaxies that are behind such a thick veil of neutral matter — so deep in the dark ages — that JWST itself will be forever unable to reveal them. The question of when the first stars truly formed, and when the dark ages first began to “brighten up” with starlight of any kind, may not be answerable by JWST.
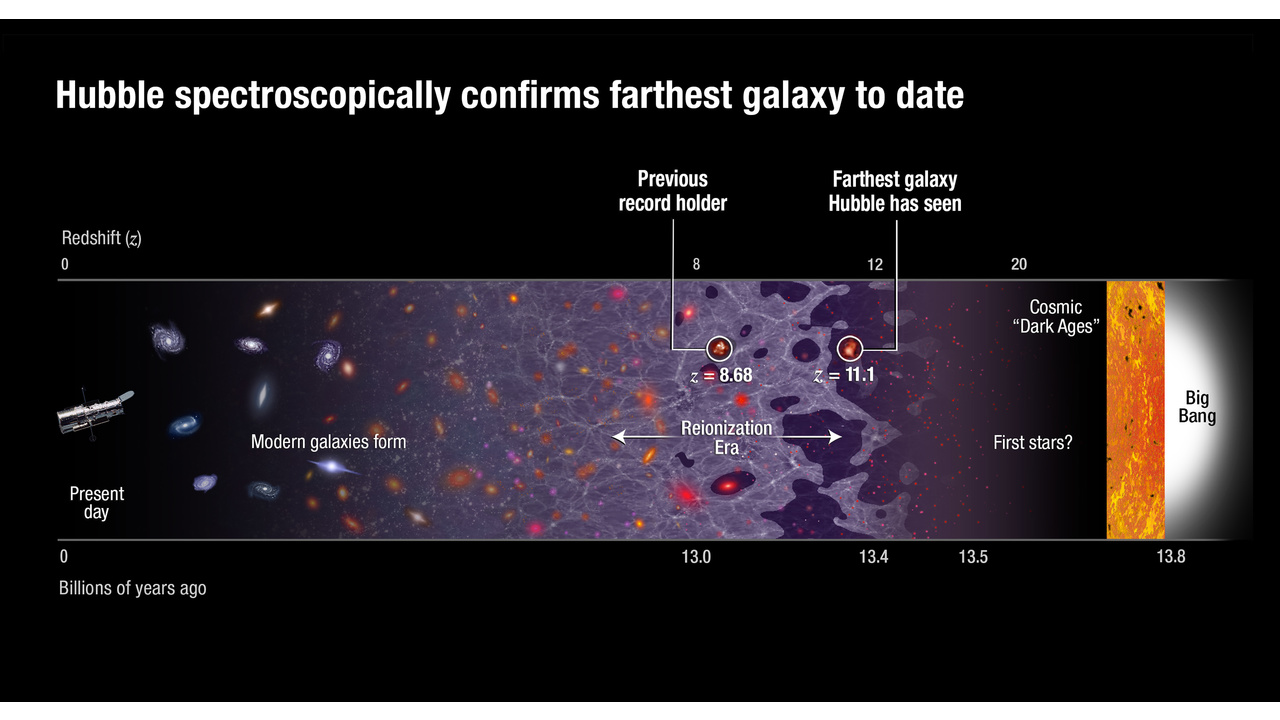
However, one of the more interesting things that both simulations and observation seem to indicate is this: while it’s the biggest, brightest, most luminous and most massive early galaxies that JWST is most sensitive to and most easily able to detect, it turns out that those objects are not primarily responsible for reionizing the Universe! Instead, it’s the far more numerous but much smaller, fainter, and lower-in-mass galaxies and star-forming regions that are responsible for the overwhelming majority of ultraviolet, ionizing photons: at least 80% and up to 95% of them by some estimates.
The dark ages began after the light from the hot Big Bang faded away from view, and the Universe remained entirely dark until the first stars began to form: a process that took tens or even 100+ million years to occur. But even once stars were present, there was so much neutral matter around that needed to be ionized that the Universe wouldn’t become fully transparent to starlight — i.e., reionized — until approximately 550 million years had passed since the Big Bang in most places, and it would take even longer in a few other regions. So that’s the story of the Universe’s dark ages, including how (and why) they came to an end. Be grateful for JWST; it’s the best tool we have for peering behind this dusty veil of neutral matter, and actually probing this “era of reionization” for ourselves!
Send in your Ask Ethan questions to startswithabang at gmail dot com!