Ask Ethan: Are we the children of neutron stars and black holes?
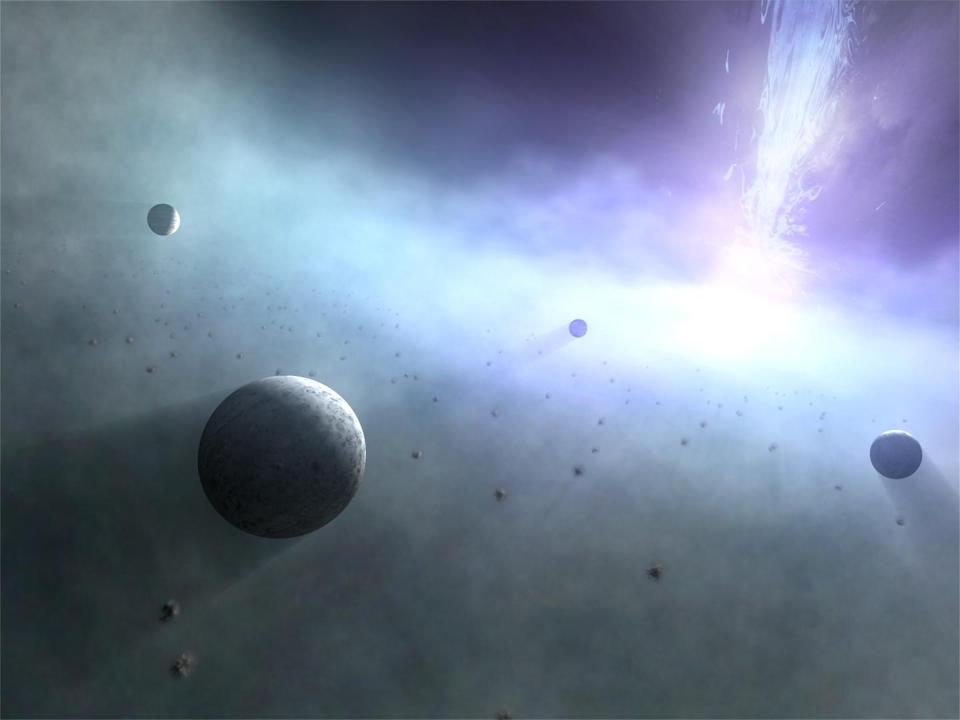
- Our solar system could only exist after numerous generations of previous stars had lived and died.
- The corpses of these prior generations — white dwarfs, neutron stars, and black holes — still litter the galaxy today.
- But do the ones we find nearby have anything to do with us? We have yet to reach a definitive conclusion.
As far as the universe goes, our own solar system is a relative latecomer to the game. The overwhelming majority of stars and planets that exist today formed long before our own sun did, with the most massive of those stars having already completed their lifecycles. When stars are born, they can arise with a wide variety of masses, and the most massive ones burn through their fuel the fastest and quickly die. Upon their deaths, they expel much of their processed material back into the interstellar medium, giving rise to future generations of stars and planets, while simultaneously leaving stellar remnants behind: corpses of now-deceased stars such as white dwarfs, neutron stars, and black holes.
Does that mean, then, that when we find these stellar remnants nearby, we’re potentially seeing our cosmic parents, grandparents, and other ancestors? It’s a fascinating possibility that Miguel Ramirez wants to know about, writing in to ask:
“[I]s it possible that when the closest neutron stars to the Earth blew up as supernovas, were they direct contributors of the materials that provided our planetary nebula with the elements necessary for our Sun, planets and ultimately, life? Are we the children of neutron stars and black holes?”
We certainly arose, in large part, due to prior generations of stars. But are the nearby neutron stars and black holes truly our cosmic ancestors? Let’s find out.
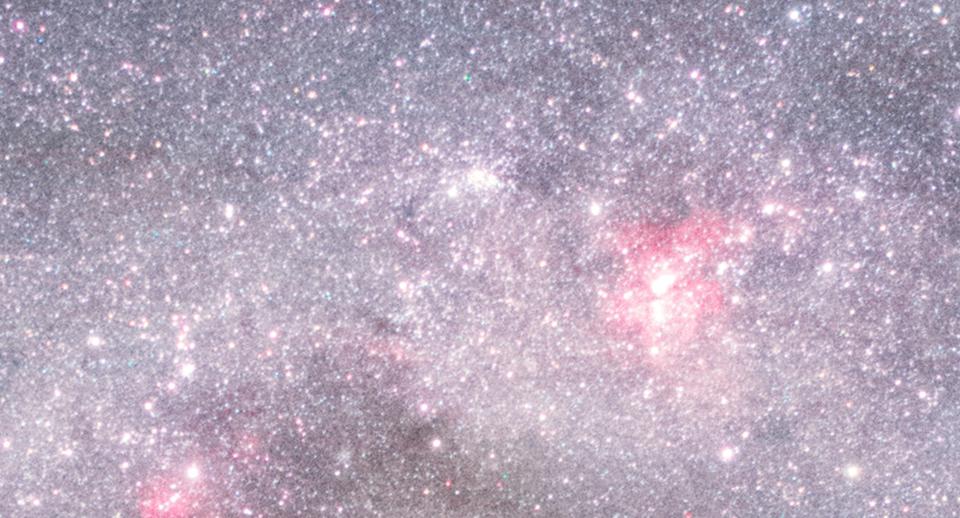
A star is born
When you look up at a clear, dark night sky, the most prominent features are stars, which are by far the most numerous of the heavenly objects that we can perceive. To the naked eye, approximately 6,000 stars are visible to humans on Earth, but there are far more out there beyond the limits of human vision. A simple pair of binoculars reveals over 100,000 stars, while our best space-based observatories, such as the ESA’s Gaia mission, can identify and measure the properties of more than 1 billion of the Milky Way’s stars.
All told, there are perhaps around 400 billion stars within our own galaxy, and as many as 2 trillion galaxies contained within the observable universe. Yet when it comes to the question of where these stars came from, all have a common origin.
Today, every star that exists in the universe formed from the gravitational contraction of a cloud of gas, which was composed of a mix of hydrogen and helium left over from the Big Bang, in addition to material that was reinjected into the interstellar medium from prior generations of stars. The stars emerged after radiating enough energy away so that at least one object could collapse sufficiently to ignite nuclear fusion in its core
Today, every star that exists in the universe formed from the gravitational contraction of a cloud of gas, which was composed of a mix of hydrogen and helium left over from the Big Bang, in addition to material that was reinjected into the interstellar medium from prior generations of stars. The stars emerged after radiating enough energy away so that at least one object could collapse sufficiently to ignite nuclear fusion in its core.
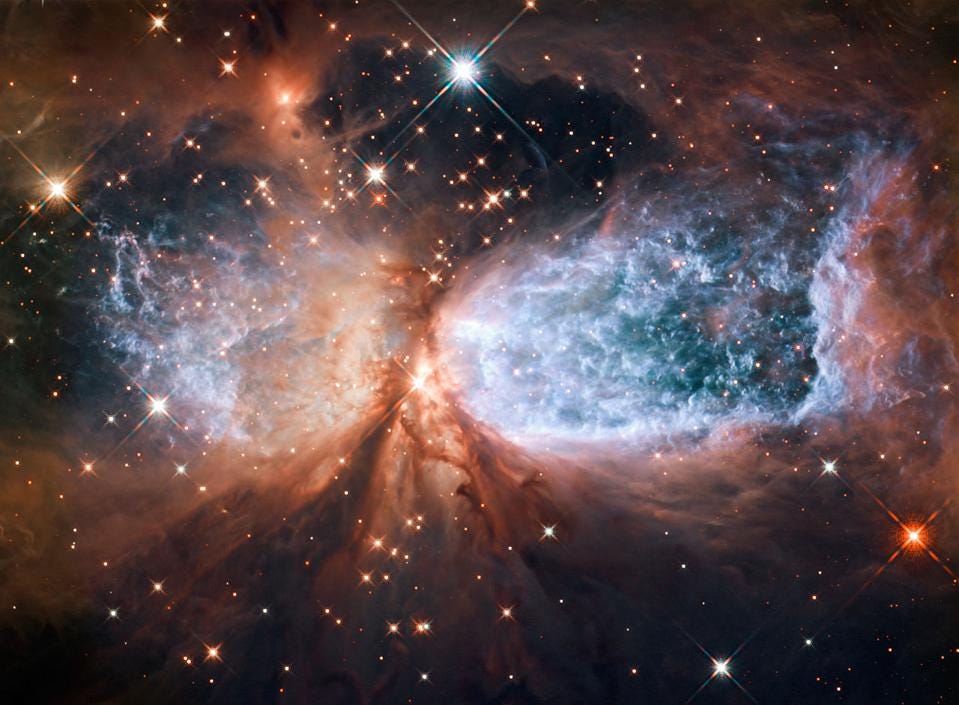
Credit: ESA/Hubble and NASA
That last step — the ignition of nuclear fusion — can only occur if temperatures in the core of a mostly hydrogen object reaches a whopping ~4 million K. This happens naturally if you put approximately 1.5 × 1029 kg of mass (about 7.5% the mass of our sun) together in one place. Although objects of all different masses form from these originally large gas clouds, it’s only the ones that cross this critical mass threshold that wind up becoming stars with their own solar systems.
It would take roughly 9.2 billion years of cosmic evolution before the gas cloud that gave rise to our solar system would first gravitationally contract, giving rise to not only our sun and planetary system, but probably thousands of other stars at the same time. Star formation doesn’t generally occur in isolation, but rather in great bursts where thousands or even hundreds of thousands of stars are created all at once. As far as we can tell, the vast majority of stars formed in nebulae alongside great numbers of stars, practically simultaneously.
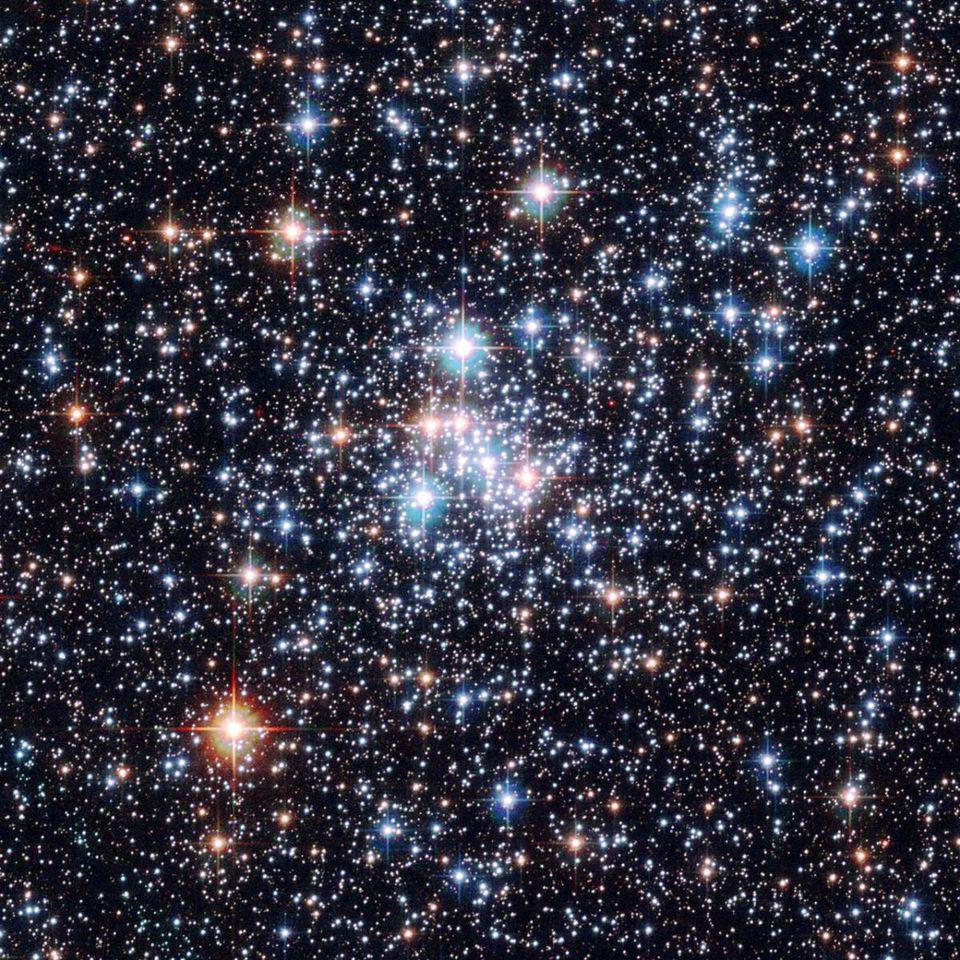
Credit: ESA & NASA; Acknowledgement: Davide de Martin (ESA/Hubble) and Edward W. Olszewski (University of Arizona)
Multiple generations
You might wonder why it is that every star that exists today includes material that was once part of a previous generation of stars, particularly if star-formation events led to stars of widely varying masses. Today, for example, when star-forming events occur, they typically give rise to:
- a few very massive stars
- a larger number of medium-mass stars
- an extremely large number of low-mass stars
- and even more objects that never quite become stars, such as brown dwarfs and rogue planets
Of the stars that are produced, only about 0.1% of them are massive enough to end their life in a supernova, leaving a black hole or neutron star behind when they die. Despite being more massive and having more hydrogen fuel than all the others, these stars are incredibly luminous and consume their fuel far more quickly than their less massive counterparts. In fact, the most massive stars, hundreds of times the mass of our own sun, live only for a couple of million years before exhausting their fuel and dying in a catastrophic supernova explosion.
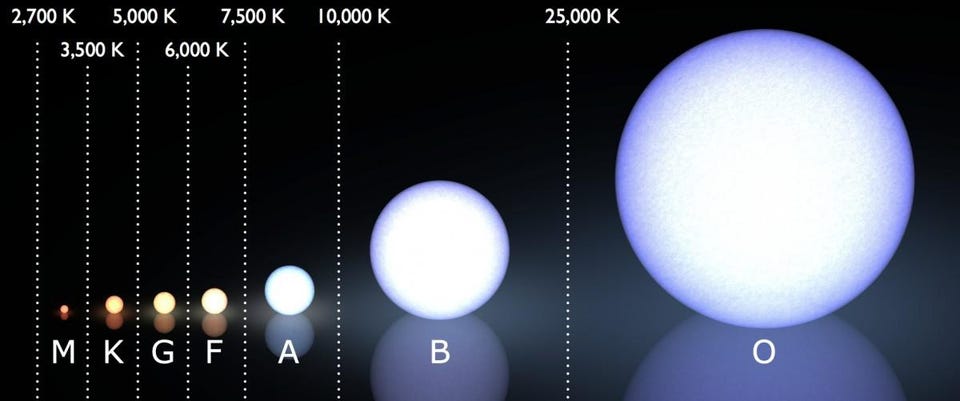
Credit: LucasVB/Wikimedia Commons; Annotations: E. Siegel
On the other hand, approximately ~20% of stars are, in some way, like our sun. These stars will burn through the hydrogen fuel in their cores, and then those cores will contract and heat up to fuse helium into carbon — the same process that occurs in their more massive counterparts. However, unlike the more massive stars, when they run out of helium, no further fusion events — and hence, no supernova — will occur.
When sun-like stars die, which can take billions of years, they simply blow off their outer layers, forming a planetary nebula, while their core contracts down to a white dwarf.
The even lower-mass stars that exist, red dwarf stars, take far longer than the present age of the universe to complete their life cycle. In fact, even though approximately 80% of existing stars are red dwarfs, not a single one has burned through all of its hydrogen fuel. While the most massive stars have already lived and died, enriching the interstellar medium and contributing to subsequent generations of stars, the low-mass stars all still exist today.
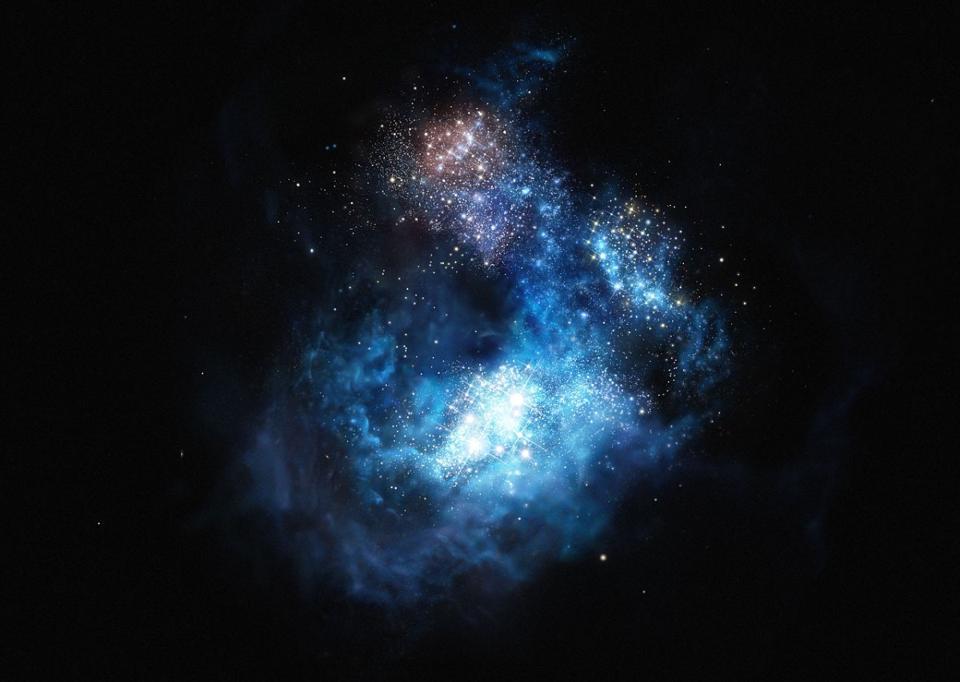
(Credit: ESO/M. Kornmesser)
But the very first generation of stars was different. Without anything appreciable other than hydrogen and helium composing them, these stars had a tremendously difficult time forming. Sure, gravity worked the same way then as it does today, as did nuclear fusion. The temperature and density thresholds needed to trigger important physical processes also remained unchanged.
With only hydrogen and helium, however, these early stars were terribly inefficient at radiating energy away, which means they could not contract down into a collapsed state the way modern stars do. As a result, the very first generation of stars was universally much more massive — what astronomers call top-heavy — than the stars that form today.
Whereas the “average” star that’s formed today may only be about 40% the mass of the sun, meaning that it’s still alive today, the “average” star that emerged during the first generation of star-formation was 10 times the mass of our sun, with a lifetime of only a few tens of millions of years. As a reslt, every member of the first generation of stars completed its lifecycle billions of years ago, dying in a supernova explosion and enriching the surrounding gas clouds for all the subsequent generations that came after.
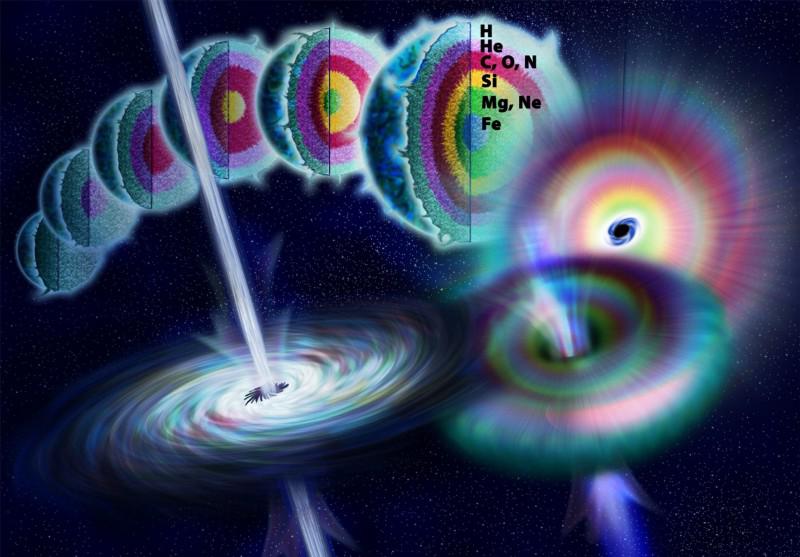
By the time we get to us
Our modern universe is full of not only stars, but the stellar remnants — i.e., the corpses of prior generations of stars — of stars that lived and died previously. Every time we find a white dwarf, neutron star, or black hole that’s older than our solar system, there’s a non-zero probability that some of the material from the once-living star that gave rise to that particular remnant now makes up our sun, Earth, and solar system. Based on how white dwarfs and neutron stars evolve, changing their temperature and spin as they age, we can measure an individual object and estimate its age. (We cannot, however, do this for black holes; we do not yet know how to reliably date them.)
The stars that we see today have a wide variety of properties:
- they range in mass from 0.075 solar masses up to ~260 solar masses
- they’re made from as little as ~0.001% and as much as ~3% of elements heavier than helium
- and they range in age from being newly born to more than 13 billion years old
However, when a major event that gives rise to new stars occurs, the stars formed in that event vary in mass only, as they all have the same fraction of heavy elements (what astronomers call metallicity) and also have the same age.
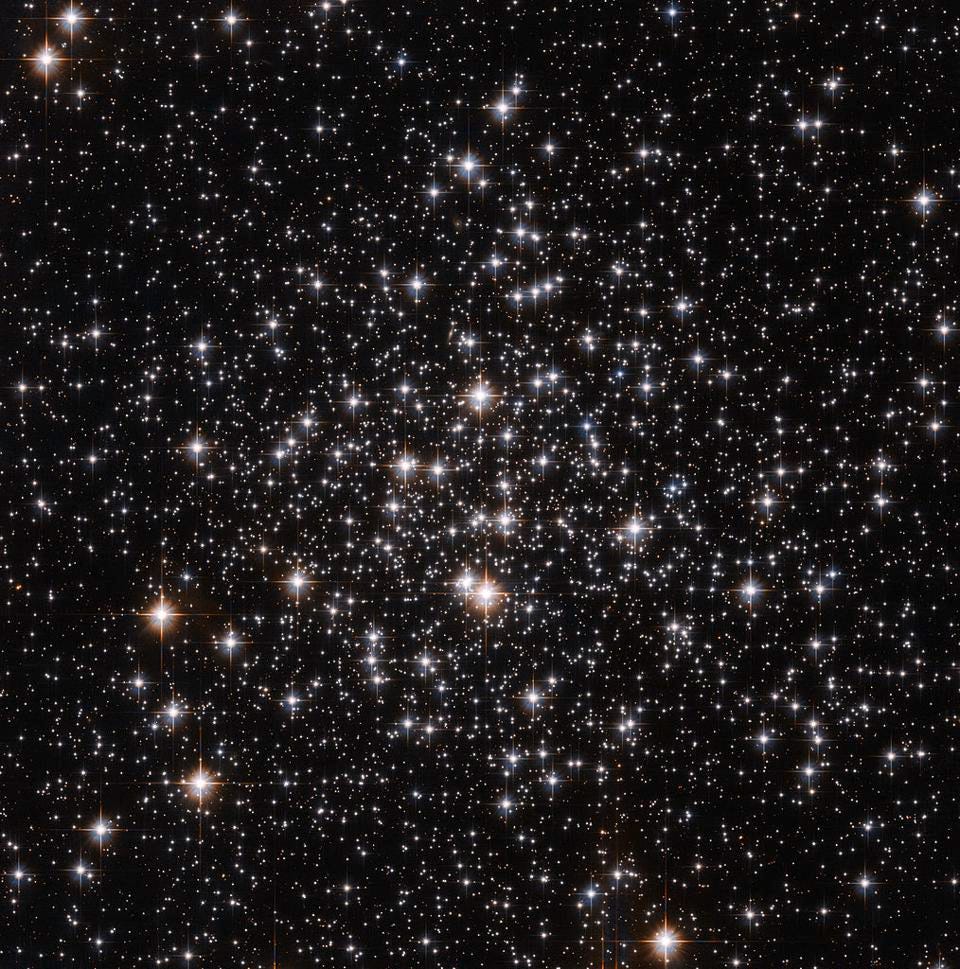
(Credit: ESA/Hubble and NASA)
In other words, it’s extraordinarily tempting to look for other stars in our nearby vicinity with the same age and the same metallicity as our own sun. If we can find a star that’s the same age and metallicity, even with a wildly different mass, it’s possible that it formed from the same cloud of gas that gave rise to our own sun. You might even have the very clever idea to measure the motion of that star through the galaxy, relative to us and the other stars, and try to reconstruct its position and our position some ~4.6 billion years ago, to see if we all originated from the same part of the galaxy.
Similarly, you might also think to look in our own backyard for white dwarfs, neutron stars, and (if we ever learn how to date them) black holes that are at least 4.6 billion years old. If you can accurately measure their motion through space, you can imagine extrapolating their orbits through the galaxy back ~4.6 billion years ago when our sun and other stars formed, and perhaps even earlier to see whether the star that lived, died, and gave rise to these stellar corpses could have contributed to the gaseous nebula from which we formed.
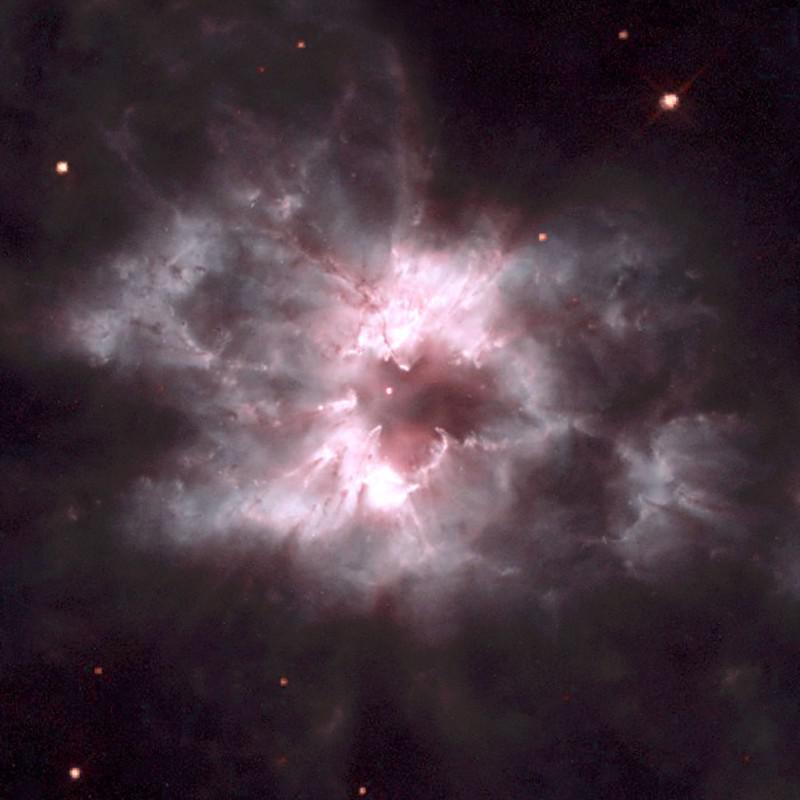
(Credit: NASA/ESA and the Hubble Heritage Team (AURA/STScI))
But if we follow this sensible and intuitive prescription, we wind up with answers that are so unreliable we might as well have resorted to random guessing. The tremendous problem with this method is that there are roughly 400 billion stars in the Milky Way, and every few hundred thousand years, on average, each star passes close enough to another star that its orbit changes appreciably. With each little gravitational “kick” that occurs, the uncertainty in a star’s previous position increases — to the point where extrapolating back even ~100 million years is unreliable, much less 4.6 billion years or more.
In fact, we have yet to identify even a single star or stellar corpse that we can confidently conclude originated from the same star-forming nebula or star cluster as our own sun. Star clusters, which result when a large number of stars all form from the same nebula, have internal gravitational interactions that cause them all to dissociate within about a few hundred million years. Many of the stars that form get gravitationally kicked so hard that they’ve since been ejected from the Milky Way. Without a comprehensive and accurate map of the stars and stellar corpses of both what was and is in the Milky Way, we lack sufficient information to draw a reasonable conclusion.
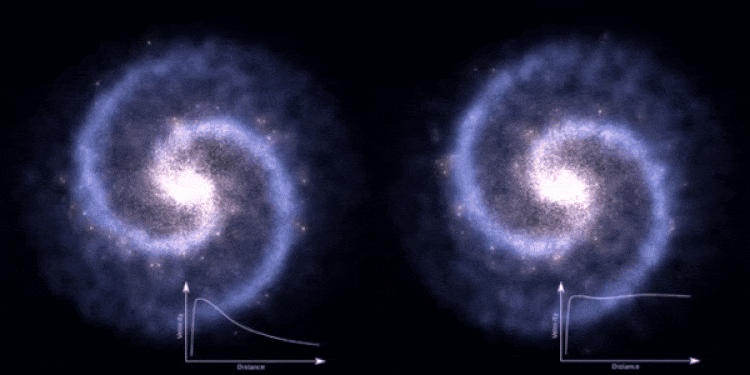
Credit: Ingo Berg/Wikimedia Commons; Acknowledgement: E. Siegel
This is part of the great frustration with an observational science such as astronomy and astrophysics. We don’t have the luxury of investigating nature with controlled experiments. We simply get a snapshot of the cosmos as it is right now: when the light from these distant objects arrives at our eyes. Despite all that we know about gravitation and all the success we’ve had in mapping out the object in our galaxy — including their three-dimensional positions and motions — reconstructing where objects were billions of years ago is an exercise well beyond our present capabilities.
We can be certain that many of the neutron stars, black holes, and even white dwarfs that exist today did, in fact, contribute to the heavy elements present in our solar system. The greater the amount of time that passed between when their progenitor stars died and when our sun was born increases the odds that some of that material got “mixed up” into the nebula that gave rise to us, no doubt. But whether any one particular object contributed to our formation history is an exercise well beyond the limits of our current capabilities. We are the children of black holes, neutron stars, and a whole lot more, but without a better knowledge of where these objects were at critical times in our galaxy’s past, we cannot identify exactly who our cosmic ancestors were.
Send in your Ask Ethan questions to startswithabang at gmail dot com!