Ask Ethan: What drives the expansion of the Universe?
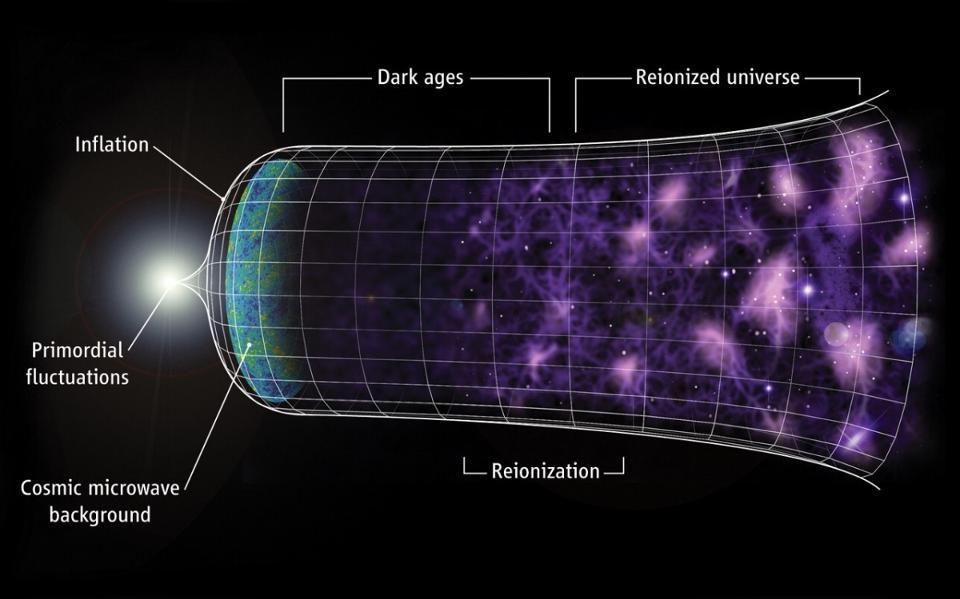
- Back in the 1920s and 1930s, some critical evidence came in to show us that the Universe wasn't a static place, but rather that all of space was expanding and evolving over time.
- In the 1990s and into the 21st century, we've learned that the Universe isn't just expanding, but that the expansion is accelerating, causing distant objects to speed up in their observed recession from us.
- But what does this imply for the cause of our Universe's expansion? It turns out to be a combination of two things: an initial expansion rate plus the effects of everything in the Universe, including dark energy. Here's what we know today.
Even though it’s been nearly 100 years since its initial discovery, the expanding Universe still puzzles almost everyone who thinks about it. What causes the Universe to expand? Why did it start off expanding in the first place? What determines the rate of expansion, and how does that rate translate into something that we can actually go and observe? What do we mean when we say “the expansion is accelerating,” and why is that such a profound, revolutionary statement that we only started making in the 1990s? And, behind the scenes, what is the ultimate cause of each aspect of our cosmic expansion, and how confidently can we state such things?
There’s a remarkable scientific story to be told here, and even seasoned scientists and science communicators frequently make mistakes when trying to provide answers to these questions. It makes educating the general public, especially young people, a particular challenge for father-of-a-curious-13-year-old, Philip Gee, who writes in to ask:
“I read everything you write, absorb 5% and talk to my kids like I’m an expert haha… I so often read this sort of thing:
‘Well, our cosmological model predicts an expanding universe, and as a consequence, the existence of an event that we call Hot Big Bang. Yet, the current state of the expansion is not constant in time, instead is increasing; thus this growing rate in the expansion has to be driven by a different factor, something that wasn’t predominantly acting during the early stages of the Universe or at times where galaxies formed.’
The expansion of space isn’t accelerating right? Well, it is, but not because the Hubble Constant is increasing? Shouldn’t we just be saying: ‘the Hubble Constant, which is a measure of expanding space over time, has to be driven by something?'”
Let’s break down and answer the different parts of this question, because even though it’s complicated, we can come to an understanding by unpacking it one step at a time. Let’s check it out, one step at a time.

The theoretical background
Imagine yourself as an astrophysicist just over 100 years ago. Einstein has just published his theory of general relativity, and with the next well-measured solar eclipse, it’s validated, as light bends and deflects according to his theory’s predictions, not Newton’s. Then, you come along and want to apply these equations to the entire Universe, in an attempt to figure out what sort of predictions it has in store.
This is exactly what Soviet scientist Alexander Friedmann did back in 1922, approximating the Universe as any spacetime that’s (roughly) uniformly filled with matter, radiation, and any other form of energy you can imagine.
Not only did he derive what many have called the most important equation(s) in cosmology, but he showed that any spacetime that was:
- uniformly filled with matter, radiation, and/or any other species of energy,
- and that possessed the same physical properties in all directions in three-dimensional space,
could not be both static and stable; it must either expand or contract. Furthermore, the rate of expansion or contraction would be determined exclusively by the combination of the energy densities of (the sum of) all the different species of energy present within the Universe, as well as by the spatial curvature of the Universe. That profound realization, borne out over the past 101 years in the form of the Friedmann equations, has been a cornerstone of physical cosmology ever since.
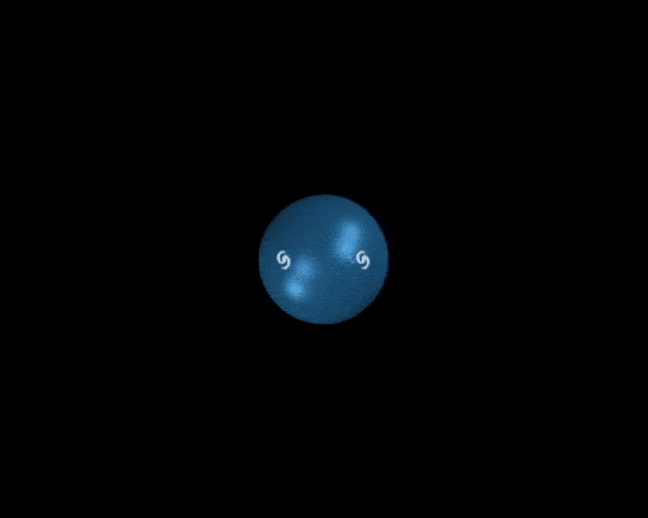
Early observations and the discovery of cosmic expansion
The evidence for cosmic expansion began with three observations:
- Henrietta Leavitt’s discovery of the period-luminosity relation for Cepheid variable stars. Just by measuring how long it takes one of these stars to go from bright-to-faint-to-bright again, you can know how intrinsically bright it is. Then, by measuring its apparent brightness, you can infer how distant it is from you, enabling you to measure cosmic distances wherever you can identify and measure these variable stars.
- Vesto Slipher’s discovery and measurement of the spectral line shifts of these spiral and elliptical “nebulae” in the skies. Whereas stars and other objects within the Milky Way appear to be moving — and hence, have their emission and absorption lines shifted dependent on their relative motion with respect to ourselves — at tens or even a couple hundred km/s relative to us, these objects moved at thousands of km/s, and were almost all “redshifted,” corresponding to motion away from us.
- And finally, Edwin Hubble (and his assistant, Milton Humason) measured Cepheids in those same spiral and elliptical nebulae, measuring their distances and confirming their extragalactic nature.
When you combine “How far away are these objects?” with “How quickly do we see these objects appearing to recede from us?” and put them on the same graph, you find precisely what Friedmann had predicted: there’s a direct relationship between the two. It could be ignored no longer: the Universe was expanding.

The big question for 20th century cosmology… and a surprising answer
But how quickly was the Universe expanding, and moreover, how was the expansion rate changing over time? Throughout the 20th century, it was often noted that cosmology was a hunt to measure two parameters:
- H0, or the Hubble parameter today, which tells us how fast the Universe is expanding right now: at present.
- q0, sometimes called “the deceleration parameter,” which is a measure of how the Hubble parameter is changing with time.
Once we came to realize that the hot Big Bang describes the early stages of our Universe, we quickly understood that the expanding Universe was a race: between the initial expansion rate, which worked to drive everything apart, and the gravitational effects of all the matter and energy within our Universe, which worked to bring everything back together. Depending on, relative to the initial expansion rate, there was more matter-and-radiation, less matter-and-radiation, or exactly the same amount of matter-and-radiation as some particular critical value would tell us something no less profound than the ultimate fate of the Universe.
- More matter-and-radiation than expansion: in this scenario, the Universe expands for a time, but gravitation not only slows that expansion down, but eventually overcomes it. Things reach a maximum size/separation, then expansion stops and reverses, and everything eventually recollapses, with our Universe ultimately ending in a Big Crunch.
- Less matter-and-radiation than expansion: in this scenario, the Universe expands and gravitation works to slow it down, but never stops it entirely. The Universe keeps expanding, forever and ever, with only isolated, gravitationally bound clumps persisting within it. This Universe eventually ends in a “Big Freeze” fate.
- Exactly enough matter-and-radiation to balance the expansion: in this final, balanced on a knife’s edge scenario, there’s exactly enough matter-and-radiation to slow the initial expansion down and cause it to approach, but never quite reach zero. If there were one more atom in this Universe, it would recollapse, but instead it just coasts forever.

It took many decades to finally reveal what the Universe was actually doing, and to practically everyone’s surprise, the answer was none of these scenarios matched the data. Instead, when we measured the Universe’s expansion history as a function of time, we found that the “deceleration parameter,” q0, was actually NEGATIVE, meaning that the Universe wasn’t decelerating right now, but was accelerating instead!
In all three of the above scenarios, if you were to start off at one galaxy and measure how fast a distant galaxy receded from you over time, you would find that its recession speed started off fast, and then slowed down over time. The rate at which it slowed down would tell you which scenario described your Universe, and would allow you to infer your Universe’s fate and, ideally, its composition as well.
But what the observations showed, instead, was that if you were to measure a distant galaxy’s recession over time, it would have started off fast, then slowed down for a time, and then, about ~6 billion years ago, stopped slowing down and started speeding up again. The deceleration parameter, q0, was positive for those first ~7.8 billion years of cosmic history, but then switched signs, passing through 0, and has been negative ever since.
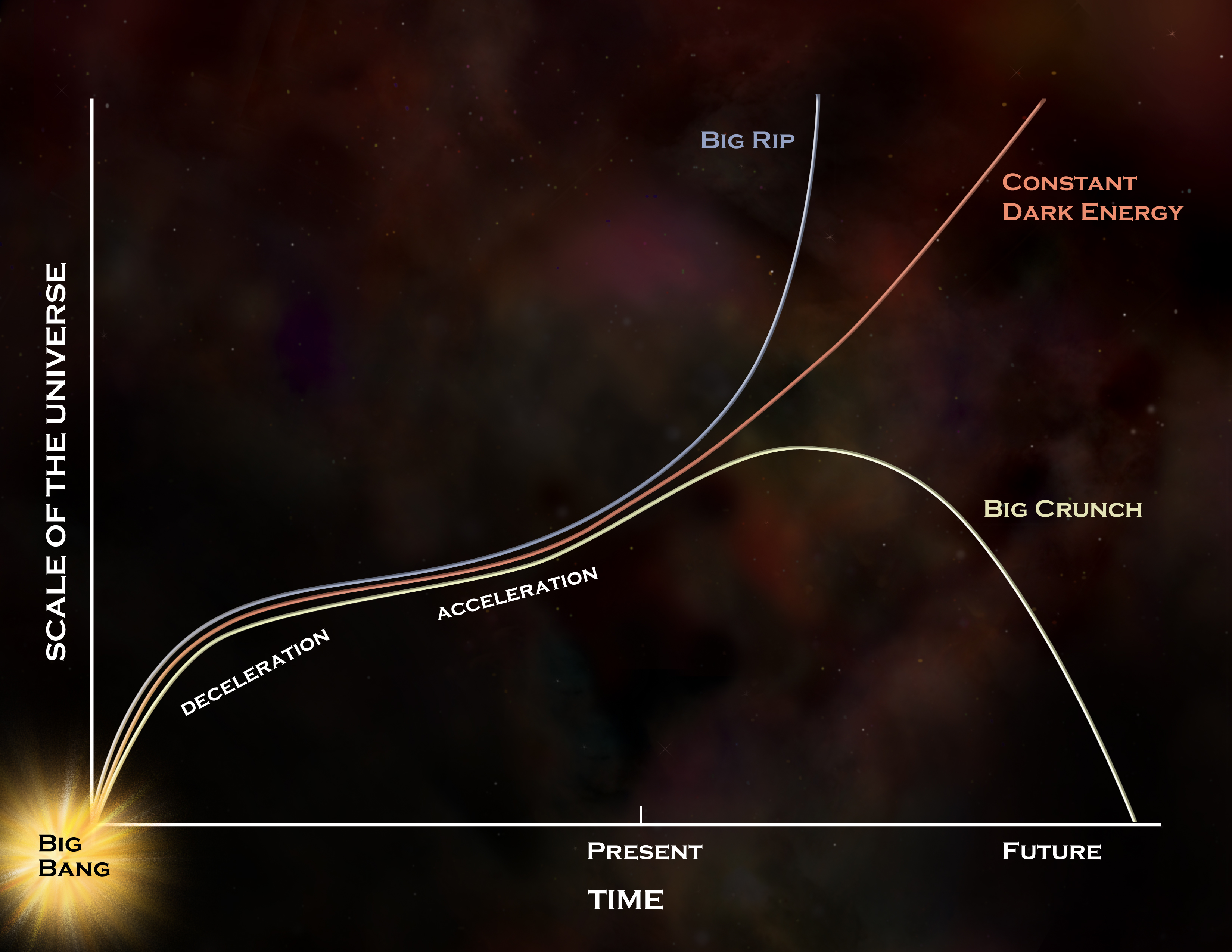
Our consensus model of what’s happening today
How did we get it so wrong, for nearly all of the 20th century? It was because of our erroneous underlying assumptions.
We had assumed that the Universe started off expanding at a certain rate, and then everything within the Universe would work to gravitationally bring it all back together again. That’s because we had assumed that everything within the Universe was (or behaved as) matter and radiation, including:
- normal, atom-based matter,
- black holes,
- photons and all forms of light,
- gravitational waves,
- neutrinos,
- and dark matter, whatever it may be ultimately composed of.
What we most often didn’t consider — at least, not until the 1990s came around — is that there might be some exotic form of energy that didn’t get less dense as the Universe expanded. But such a thing is clearly possible: there could be energy inherent to the fabric of space itself. Two possible and compelling theoretical origins for this include:
- Einstein’s cosmological constant, which can be written down in any spacetime,
- and the zero-point energy of the quantum vacuum, which may not be zero, but which might instead have a positive, non-zero value everywhere.
Both of these explanations are still 100% valid and consistent with all of the data, and the phenomenon of the accelerated expansion of the Universe is most typically described as being driven by some form of dark energy, which is the generic term for any species of energy that would lead to the types of cosmic accelerations we observe.

What’s accelerating, what isn’t, and what does it mean?
This is where the biggest points of confusion often arise: when it comes to the question of what it is, exactly, that’s accelerating.
In cosmology, we normally talk about the expansion rate as being described by the Hubble parameter: H, or the Hubble parameter today, H0. This is normally expressed and measured in units of km/s/Mpc, which is to say that a distant object recedes as though it has a recession speed of a certain amount (a certain value of km/s) for every megaparsec (Mpc, or about 3.26 million light-years) of distance it has from us today.
This value — the expansion rate — does not accelerate (or increase), not even with dark energy. Without dark energy, it always drops to approach zero (and reverses in the “Big Crunch” scenarios), but with dark energy, it only drops to and approaches some finite, positive, non-zero value. According to our best measurements, the current expansion rate is around 70 km/s/Mpc, but will someday drop to around 45 km/s/Mpc, but no lower, in the Universe we inhabit. The expansion itself is accelerating, but that doesn’t mean the expansion rate is accelerating. Ever since the hot Big Bang, it’s been decreasing, and it’s still decreasing today; the fact that the Universe is accelerating just tells us that the final, ultimate value that it approaches won’t be zero, but a positive, greater-than-zero value.
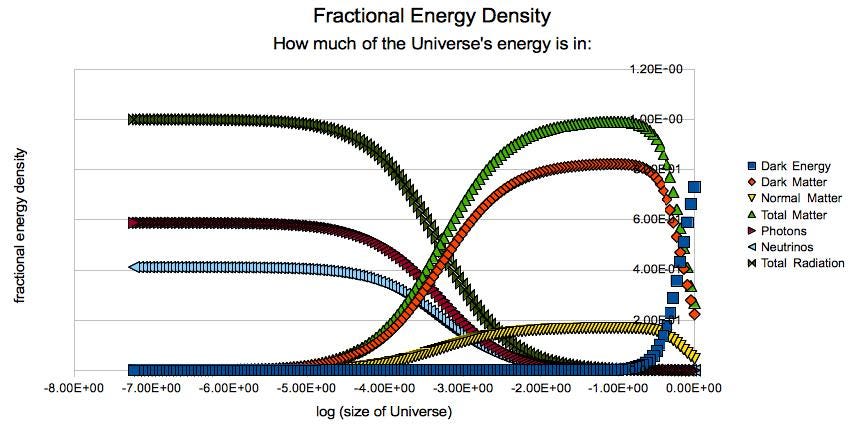
What is accelerating, though, is the recession speed that you measure for any individual object within the expanding Universe. If a distant galaxy, today, is located about 1 billion light-years (around 300 Mpc) away, then it’s receding at around 21,000 km/s. At some point in the future, it will be twice as far away: 2 billion light-years (around 600 Mpc), and when it is, even though the expansion rate will have dropped by a little (maybe to 60 km/s/Mpc), it will be receding at a faster rate of around 36,000 km/s. In the even more distant future, it will reach a distance of around 21.7 billion light-years (6,667 Mpc), and even though the expansion rate will now be at its minimal value of ~45 km/s/Mpc, this object will now be receding at 300,000 km/s: greater than the speed of light.
This implies that there’s only a limited amount of time we have — or that anyone, anywhere has — to reach a distant galaxy that’s not bound to the same galaxy group-or-cluster that they are. As objects get pushed to greater and greater distances, their recession speed will appear to progressively increase with no upper bound, surpassing even the speed of light at some point. Once that occurs, no signal, spaceship, or message that is sent off can ever reach that destination, implying that there’s a “reachability” limit as well as a “visibility” limit for every object in the Universe beyond one’s own Local Group.

And finally, what’s ultimately driving the expansion of the Universe?
So what, then, is the ultimate cause of the Universe’s expansion? It turns out that there are two things responsible, that a large number of things that we previously thought could be responsible aren’t, and that the two things that are responsible are only possibly related to one another: the initial expansion and the onset of dark energy. We have to consider both independently, and then, and only then, the possibility that they might be related.
Where did the initial expansion rate, the rate at which the Universe began expanding at the start of the hot Big Bang, come from?
This arises from the end of cosmic inflation: the period that preceded and set up the hot Big Bang. During inflation, the Universe was expanding relentlessly — at a constant rate — as though there were a large amount of energy inherent to space itself during this epoch. With every ~10-35 seconds or so that elapsed, the Universe would double in size in all three dimensions: in length, in width, and in depth. The energy density of space would remain constant, even as this expansion constantly created new space. When inflation came to an end, practically all of this energy was transformed into matter-and-radiation, with the matter-and-energy density at that time determining the expansion rate. That’s why the Universe, our Universe, began expanding so rapidly right at the start of the hot Big Bang, and also why the expansion rate and the matter-and-energy densities balanced out so perfectly.

For billions of years, as the matter and radiation densities dropped, the expansion rate dropped along with it: in direct proportion to the square root of the overall energy density, just as Friedmann’s equations predicted. And then, those densities dropped by a great enough amount so that a new form of energy began to influence the expansion rate: dark energy, which behaves indistinguishably from either
- a cosmological constant,
- the zero-point energy of space,
- or to energy inherent to the fabric of space itself.
The value of this energy density is incredibly small: a factor of ~1025 smaller than it was during inflation, but its presence at all makes it inevitable that it will eventually come to dominate the expansion of the Universe. It only took several billions of years, and now here we are: living in a Universe dominated by dark energy, where it’s responsible for driving the expansion rate.
Many other things could have driven the expansion of the Universe: spatial curvature, topological defects, exotic forms of energy, etc. Yet it appears that, other than a period where the expansion rate and the matter-and-radiation densities were balanced, it’s always been some form of energy that behaves like it’s inherent to space that drives our cosmic expansion. It brings up a speculative but tantalizing possibility: that the early period of inflation and the current period of dark energy-domination are related. It’s possible, but no one knows how — or even whether — there’s a relationship at all. We know these things exist and observe their effects, but an underlying explanation for “how” or “why” still eludes us at present. Perhaps some young, creative, ambitious person reading this right now will be the one to discover the answers!
Send in your Ask Ethan questions to startswithabang at gmail dot com!