Ask Ethan: Is the Universe’s expansion accelerating or not?
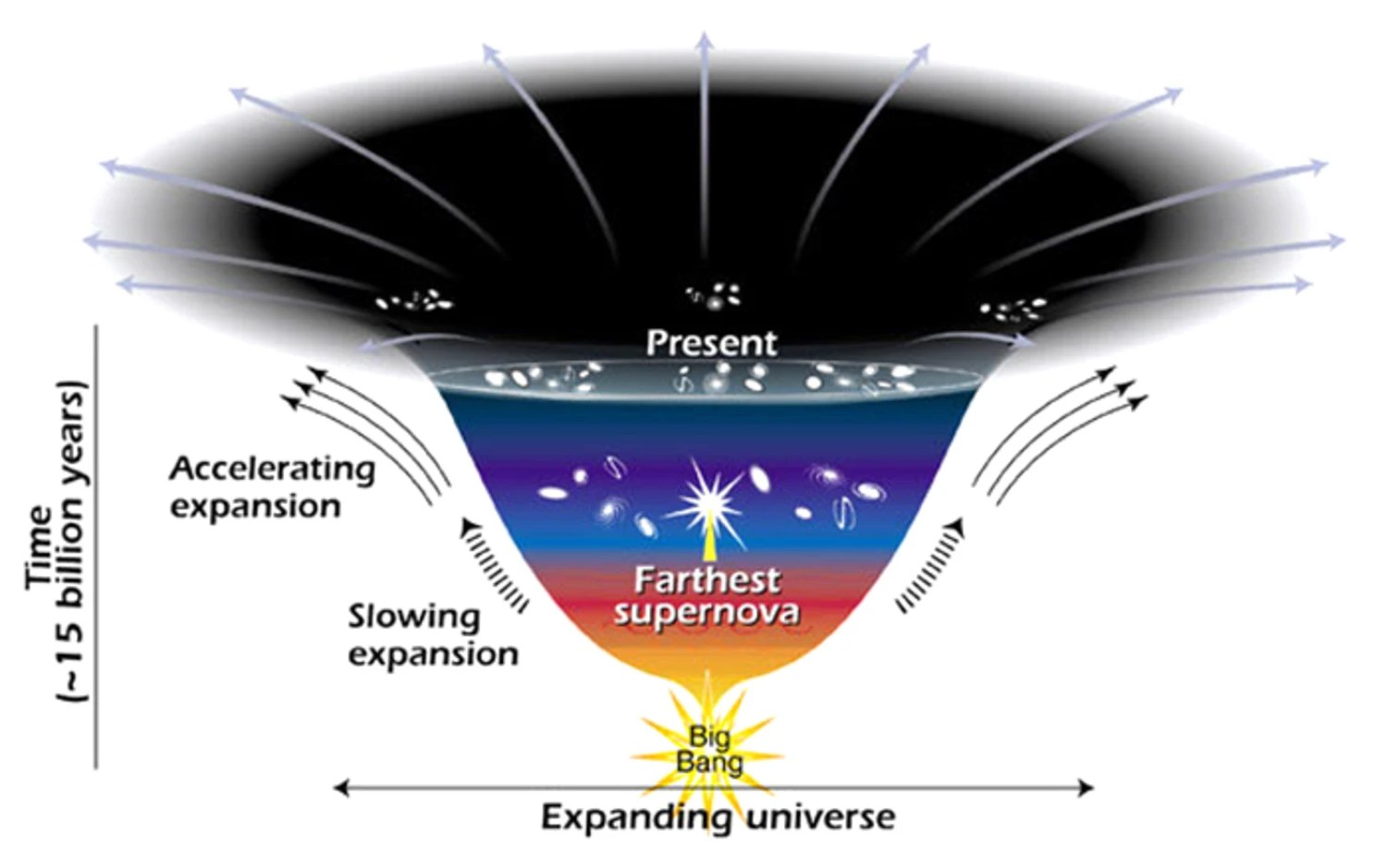
- Ever since the observational evidence for dark energy became robust and overwhelming some ~25 years ago, astronomers have talked about the accelerated expansion of the Universe.
- It’s true, at least in a sense: if you put your finger down on a galaxy that isn’t bound to our own, it will recede away from us at faster and faster speeds as time marches forward.
- But the expansion rate itself, also known as the Hubble constant/Hubble parameter, isn’t accelerating or increasing at all; it’s dropping. Here’s how to clean up the biggest misconception about dark energy.
One of the biggest surprises in all of science history came at the very end of the 20th century. For the prior ~70 years, astronomers strived to measure the expansion rate of the Universe, hoping to discover what made up our Universe and to determine its ultimate fate. Quite unexpectedly, they discovered that the Universe wasn’t made up solely of matter and radiation, but was actually dominated by a novel, unexpected, and still poorly-understood form of energy: dark energy. Making up around 70% of the total energy density of the Universe today, it quickly became synonymous with a somewhat different phrase: the accelerated expansion of the Universe.
But it turns out that the Universe’s expansion rate, which we measure as the Hubble constant (or, more accurately, as the Hubble parameter), isn’t accelerating or even increasing at all; it’s actually dropping. What’s the deal? That’s what Frank Kaszubowski wants to know, writing in to ask:
“In this lecture, you pointed out that there is a misconception between the terms “expansion” and “acceleration”. Did I understand it correctly that the acceleration is only an apparent one?”
The expanding Universe is one of the most challenging concepts to wrap your head around, even for many experts in physics, astrophysics, and General Relativity. Here’s what is and isn’t accelerating, and what’s truly going on with the expansion rate.

The first thing we need to understand is what, exactly, we’re capable of measuring when it comes to the expanding Universe. We can’t actually measure this intrinsic property of space; all we can measure is what effects the expanding Universe has on the light that we receive from distant objects. The light we observe has a specific intensity over a specific set of wavelengths, and our observatories and instruments can be optimized to perform spectroscopy: recording even slight differences in the amount of light we receive as a function of the wavelength we observe. We measure the light we receive, and it’s up to us to do so as precisely and accurately as possible.
Because we know the properties of the atoms and ions that make up light-emitting (and, for that matter, light-absorbing) objects, including the specific quantum transitions that occur within those bound states, we can determine how severely that observed light is “shifted” from the rest-frame in which it was emitted. When an electron in a hydrogen atom, for example, drops from the first excited state down to the ground state, it emits an ultraviolet photon of precisely 121.5 nanometers. But for almost every object that we observe that contains hydrogen in an excited state, we don’t see an emission (or absorption) line at 121.5 nanometers at all.
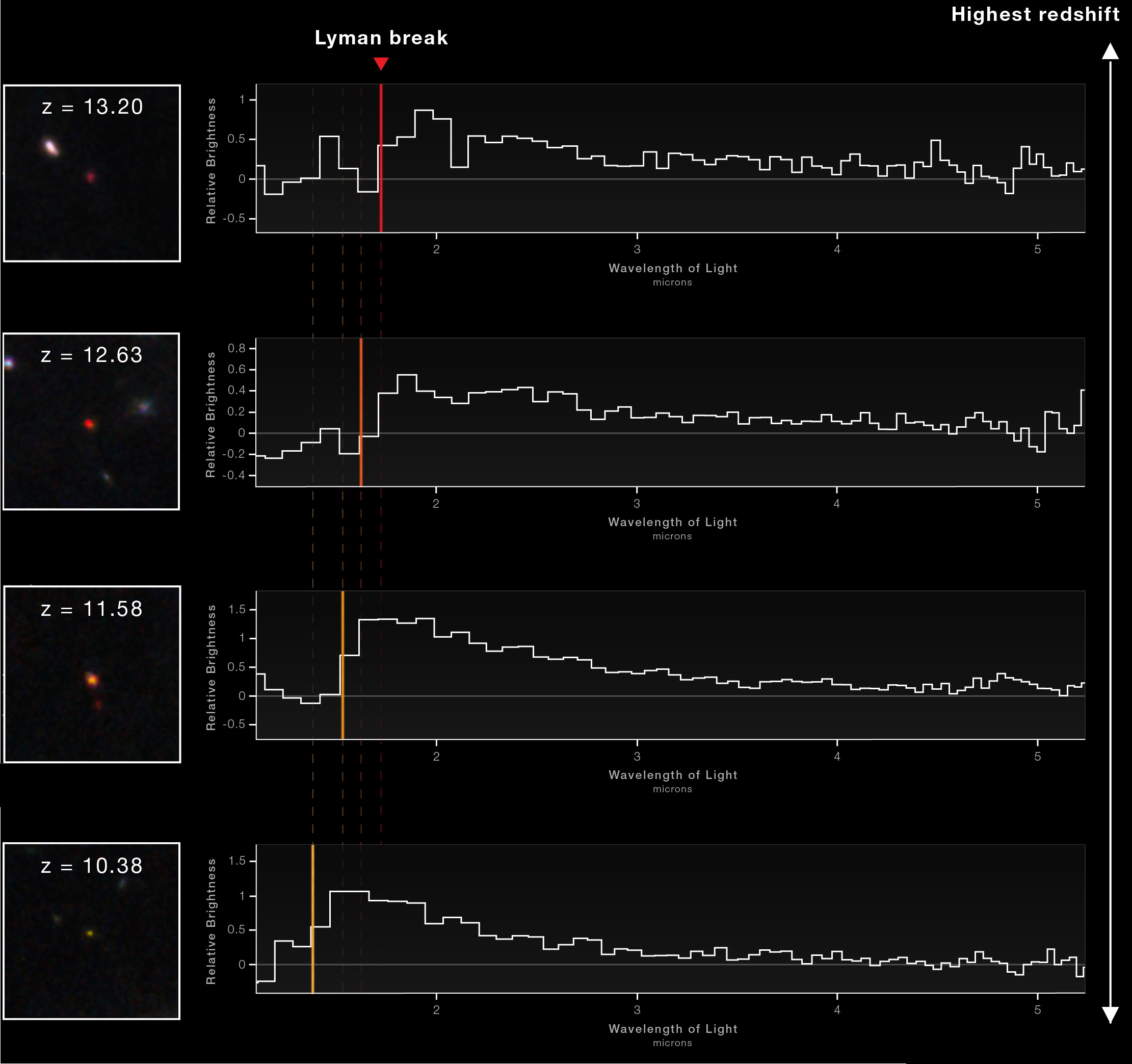
The feature exists, and in the rest-frame of those hydrogen atoms themselves, the light is emitted at precisely 121.5 nanometers, as the laws of physics do not change from place-to-place or moment-to-moment. However, there are a number of effects that can alter the properties of the light that we observe from the atoms that initially emitted that light. They include:
- Thermal effects, as atoms at a finite temperature will be randomly moving omnidirectionally, causing the emission (or absorption) line to broaden, based on the temperature of the atoms that make them up.
- Kinetic effects, such as the rotation of the host galaxy from which the light originates, which also causes the light-emitting (or light-absorbing) material to move, but from a distinct physical mechanism from thermal effects.
- Gravitational effects, such as blueshifting to shorter wavelengths when you fall into a gravitational potential well (i.e., when light enters our Local Group, galaxy, and Solar System) and redshifting to longer wavelengths when you climb out of one.
- Peculiar velocity effects, which encode the motion of individual objects relative to the local standard of rest, and which must be factored in for both the emitting and observing locations, as they cause a Doppler shift that affects the light’s observed wavelength.
- And the expansion of the Universe, which stretches all wavelengths of light to be greater and greater for the entire time that light travels from its point of origin to its ultimate destination.
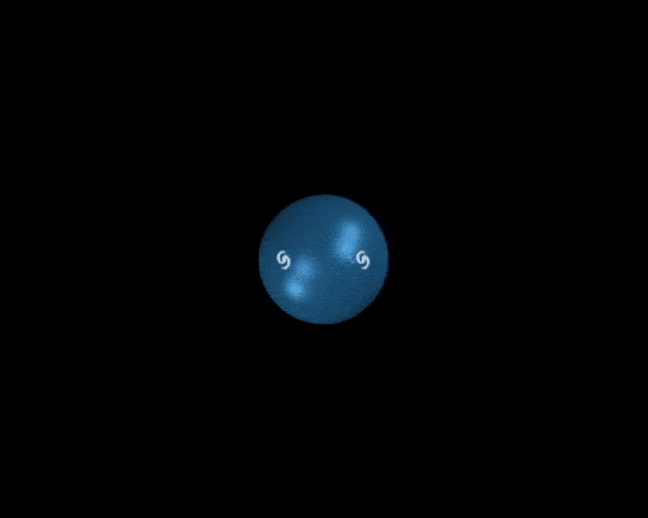
For two objects that are near one another, the first four effects can be large relative to the fifth. However, for objects that are sufficiently well-separated, the expansion of the Universe becomes by far the dominant effect; when we measure the light from a very distant object, the observed redshift (and it’s always a redshift and never a blueshift beyond a certain distance) is almost 100% due to the effects of the expansion of the Universe.
That’s what we measure: the brightness of a distant object as a function of wavelength, we identify the wavelength at which certain atomic, molecular, and ionic transitions occur, and we use that to infer a redshift for a distant object. For objects that are more distant than a few hundred million light-years, we can justifiably chalk ~100% of that redshift up to the effects of the expanding Universe.
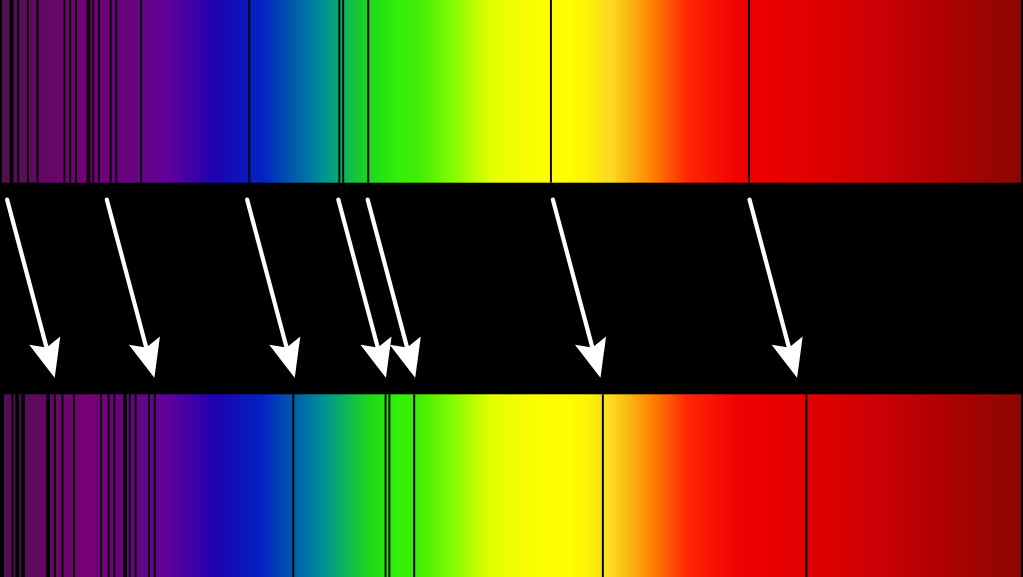
Now, one way to look at the expanding Universe is to consider that space itself expands, and the light that travels through it stretches in wavelength due to that expansion during the entirety of its journey. (And hence, more distant objects travel for longer periods of time and have their light stretched by greater amounts.) But another, equivalent way to conceive of it is as though the distant object is receding away from us at a certain speed. This is why sometimes you’ll see astronomers talk about a distant galaxy’s redshift, and other times you’ll see them talk about a distant galaxy’s recession velocity. The measurements are the same either way; it’s just a matter of how you interpret the result.
Either way, this is where the connection between what you measure (light of specific wavelengths, that reveals how much it’s redshifted by relative to its emitted rest-frame) and an inferred recession speed arises. If that same distant object you initially observed begins receding faster-and-faster over time, we’d say this object is accelerating away from us; if its redshift drops and it recedes more slowly over time, we’d say the object’s recession is decelerating. For most of the 20th century, one of the major goals of the science of cosmology was to measure the rate at which objects accelerate or decelerate over time.
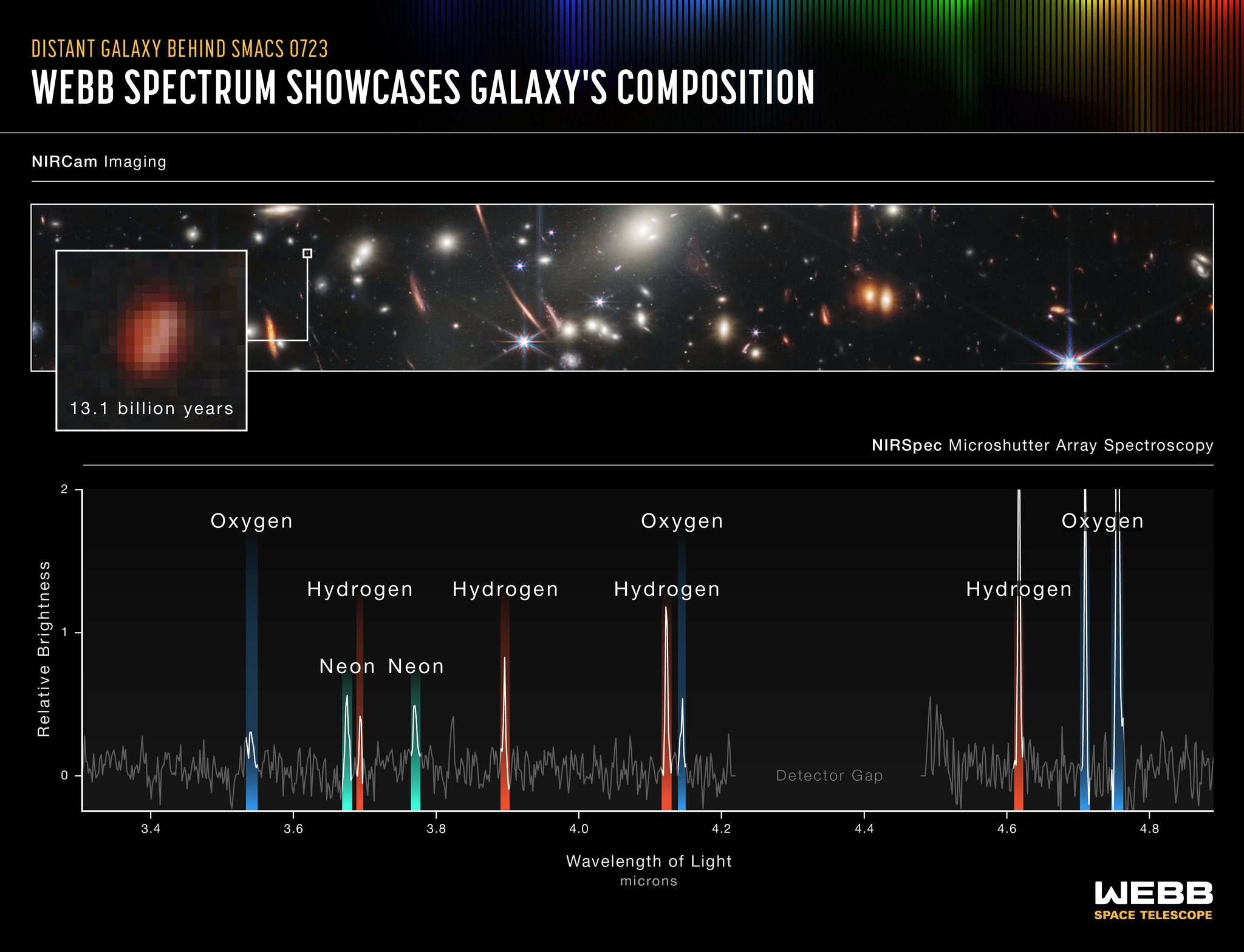
From a practical perspective, this measurement is pretty much impossible. Humans have only been around for a short period of time on a cosmic scale, and it’s really only been a little more than a century where we’ve had the capability to measure things like redshift with any sort of accuracy or precision. In order to measure how an object’s redshift (or recession speed) changes with time, you realistically need to measure it at multiple points in time, separated by hundreds of millions of years or more. Given the longevity of our species, that simply isn’t possible.
But there’s a very clever way around this. There are a few things that we know with a very strong degree of confidence.
- We know that General Relativity works extremely well as the gravitational rules our Universe plays by.
- We know that the Universe, on the largest of cosmic scales, is the same at every location and in all directions.
- We know that the Universe is expanding.
- And we know that light always travels at the same speed — the speed of light in a vacuum — from the moment it’s emitted until the moment it’s received and absorbed.
Armed with only those pieces of knowledge, we can “make up” for the fact that we can only see a single snapshot of our cosmic history.

Instead of measuring how a single object’s redshift (or recession velocity) evolves over time, and using those measurements to determine whether those objects are accelerating or decelerating in their motion away from us, there’s a trick we can leverage. If we can gather enough objects at a variety of distances in the expanding Universe, we can use the fact that all of the light is arriving right now, but light from each individual object has been traveling through the expanding Universe for different amounts of time. With enough objects at enough different distances, we can reconstruct both what the Universe is made out of and — because we know the physics of how energy density relates to the expansion rate (the expansion rate is always proportional to the square root of the total energy density) — how it’s expanded over the entirety of its cosmic history.
We’ve done that quite exquisitely, and determined that the Universe today is made of:
- about 0.01% radiation, which dilutes as the fourth power of the size/scale of the visible Universe,
- about 4.99% normal (atomic + neutrino-based) matter, which dilutes as the third power of the size/scale of the Universe,
- about 27% dark matter, which also dilutes as the third power of the size/scale of the Universe,
- and about 68% dark energy, which doesn’t dilute, but rather maintains a constant energy density.

Over time, the Universe expands: a region of space that takes up a certain volume today will, tomorrow, expand to take up a larger amount of volume. The matter and radiation within it has a constant number of particles, but as the volume increases, the density drops. Dark energy is different though; it has a constant energy density, so even as the volume increases and the Universe expands, its density doesn’t drop.
Because the expansion rate is always proportional to the square root of the total energy density (from all the different components, combined), a Universe made solely of radiation, normal matter, and dark matter will eventually see its expansion rate drop to zero, and that corresponds to a distant galaxy, over time, receding from us slower and slower, and we’d also see its redshift decreasing with time.
But in a Universe that also has dark energy — our Universe — even as the radiation, normal matter, and dark matter densities drop to zero, the dark energy density will always maintain that same constant value. Because the square root of a constant is still a constant, that means the expansion rate won’t drop to zero, but rather will only drop to some finite, positive, greater-than-zero value.

Today, we measure the expansion rate to be in the ballpark of 70 km/s/Mpc, which means that for every megaparsec (Mpc, or about 3.26 million light-years) of distance, an object at that distance recedes at an additional 70 km/s. In a Universe with no dark energy, that expansion rate will someday drop all the way to 0 km/s/Mpc, and if you were to measure any individual object over time, its recession speed would appear to slow. But in our Universe with dark energy, the expansion rate will only drop to a minimum of somewhere between 45 and 50 km/s/Mpc.
In other words, the expansion rate of the Universe, even in a Universe with dark energy, still always decreases with time. The expansion rate is not accelerating; it’s actually shrinking. What’s different is that it doesn’t shrink and approach zero; it shrinks and approaches a finite, positive, non-zero minimum value.
Imagine what happens in a Universe where only dark energy remains, and the expansion rate is 50 km/s/Mpc. An object that starts out 10 Mpc away will begin receding at 500 km/s, which pushes it out to greater distances. When it’s 20 Mpc away, it will then recede at 1,000 km/s; when it’s 100 Mpc away, it recedes at 5,000 km/s; when it’s 6,000 Mpc away, it recedes at 300,000 km/s (about the speed of light); when it’s 1,000,000 Mpc away, it recedes at 50,000,000 km/s.
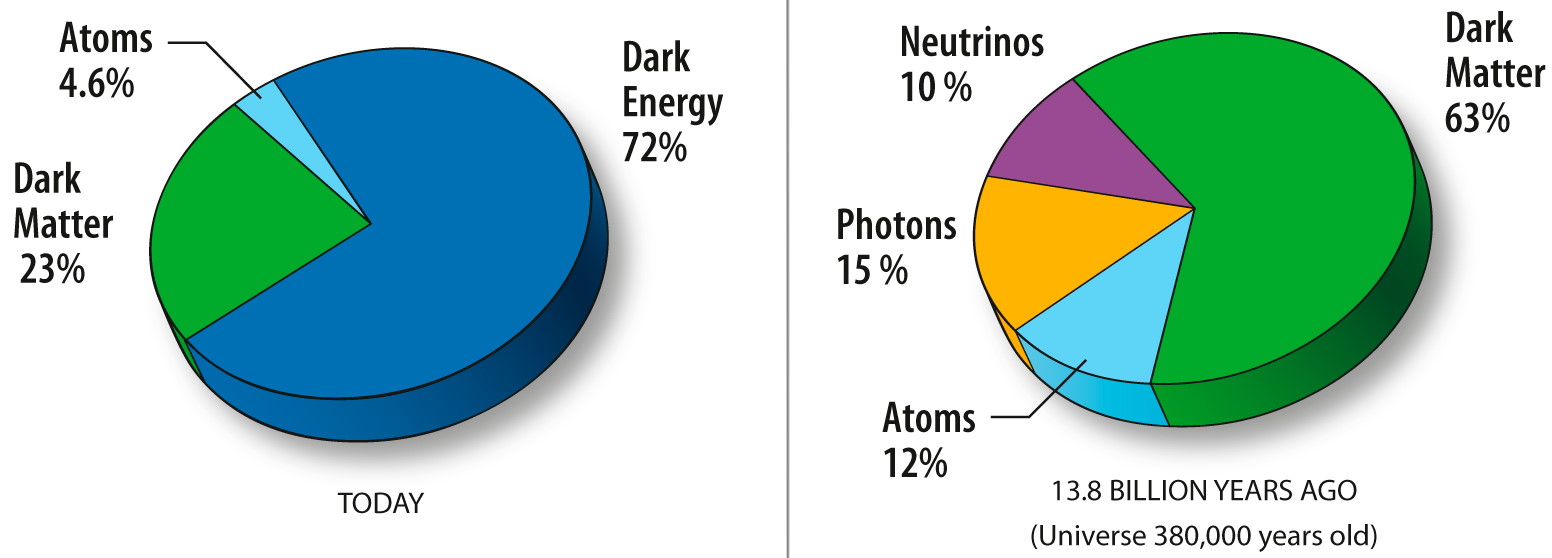
Long ago, when all the matter and radiation were packed into a much smaller volume of space, the dark energy density was extremely small compared to the matter and radiation densities. As a result, for the first few billion years of cosmic history, distant objects slowed down in their recession from us (and their redshift lessened) as time progressed. But when the matter and radiation densities dropped below a certain threshold, and the dark energy density became a significant enough fraction of the total energy density, those same objects once again sped up in their recession from us, and their redshift increased.
Even though the expansion rate — also known as the Hubble constant/parameter — still decreases, for the past ~6 billion years it’s been decreasing at a slow enough rate that as the volume of the Universe grows, these same distant objects now appear to recede away from us faster and faster; they’re now moving away from us in an accelerated fashion.
The Universe is expanding, the expansion rate is dropping, but it’s not dropping to zero; it’s in the process of asymptoting to a final value that’s only about 30% lower than its current value today. However, each individual object that’s receding from us will recede at faster and faster speeds as time goes on. Importantly, this implies that the recessional velocity of each galaxy is accelerating, but the expansion rate itself is not; it’s decreasing. It’s a challenging misconception to overcome, but hopefully now — armed with an in-depth explanation in plain English — you’ll understand that the objects within the Universe are accelerating, but the expansion rate of the Universe is not!
Send in your Ask Ethan questions to startswithabang at gmail dot com!