Ask Ethan: Did time run slower in the early Universe?
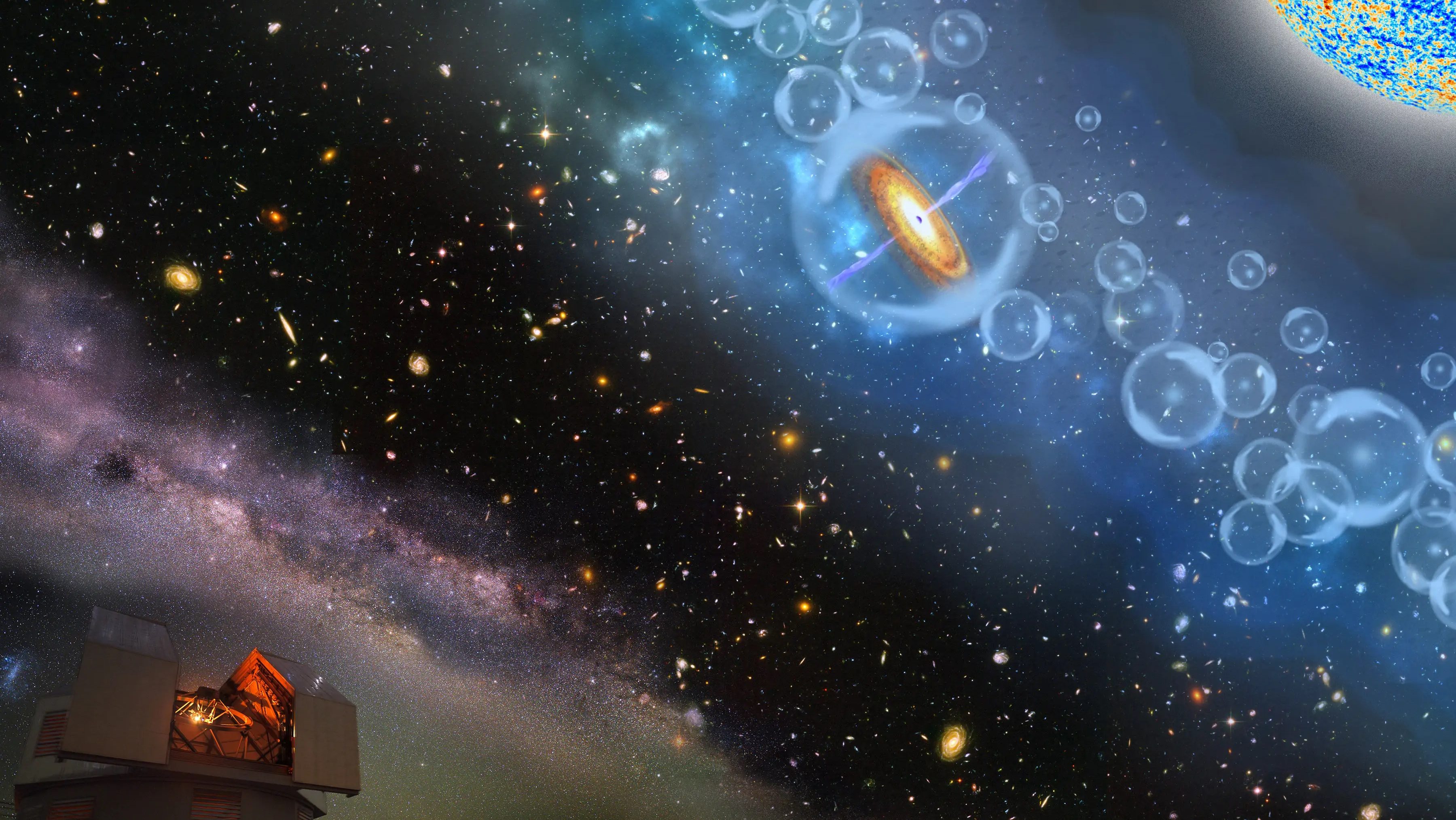
- A new study has been making waves, examining 190 quasars to show that a periodic “ticking” appears slower to us the longer ago the quasar light was emitted.
- In a sensationalistic and quite incorrect fashion, many outlets have been reporting that this means that “time ran slower in the early Universe,” which isn’t right.
- Instead, as the Universe expands, signals passing through it experience time dilation: a consequence of General Relativity. We’ve seen this effect many times before; now learn what it means.
No matter where or when you’re located in spacetime, you always experience the same laws of physics. The fundamental constants remain constant across space and time, and so do our notions of mass, distance, and duration. Rulers, or any measuring stick made of atoms, will always have the same length, and clocks, or any device made to measure time, will always show that it passes at the same universal rate for all observers: one second per second. There are never any exceptions to that, neither according to the laws of quantum theory, nor according to Einstein’s general theory of relativity.
But if you’ve been paying attention to the news, that might not be what you’ve been reading as of late. A news release from July 3, 2023 — a release that’s gotten quite a bit of traction — claims that “the Universe was five times slower soon after the Big Bang.” Many have written in to inquire about this, including Howard Vernon and Elise Stanley, asking:
“Since we’ve just discovered that time flowed more slowly in the early universe…”
“With the recent discovery [of slow, distant quasar ticking] might it be timely to do an article on time dilation…”
And I think the only option is to separate fact from fiction. Let’s unpack what’s really going on with clocks, time, and the expanding Universe.
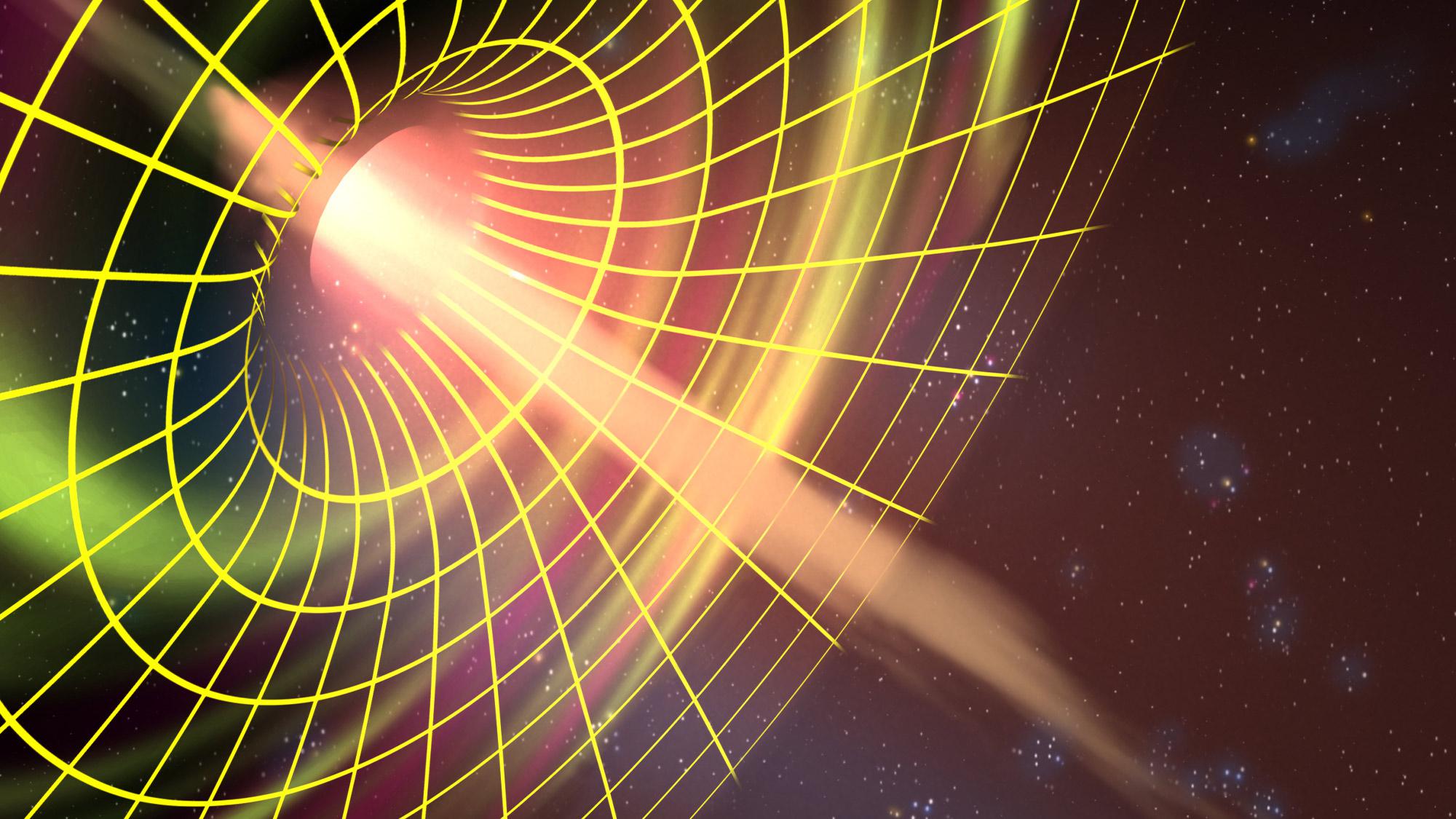
Time in the Universe
One of the greatest advances in our understanding of physics came when Einstein put forth relativity: the notion that quantities like time and space are not absolute in any sense, but rather are specific to each and every observer. Dependent on where-and-when you are, as well as how you’re moving, you may have a different perception of how far apart two objects are (distance) or how long it takes (time) for two different signals to arrive. Unlike the Newtonian idea where space was like a cartesian grid and time was an absolute, Einstein’s work showed us that each observer has a unique experience of what space and time are.
However, by properly understanding the laws of relativity, we can “transform” from what any observer anywhere in the Universe experiences to how any other observer will see distances and durations for them. For you, no matter where or when you are, as long as you’re in what we call an inertial frame of reference (i.e., you’re not accelerating due to thrust, an external force, or anything other than the curvature of spacetime), you will experience distances as being proper (where a meter stick made out of atoms measures 1 meter in any orientation) and time as being proper as well (where one second on your clock means that one second of experienced reality has passed).
In other words, while everyone experiences the same laws of physics for themselves, they may see lengths as “contracted” or time as “dilated” for other observers, dependent on the curvature and evolution of spacetime and the relative motions of the observer and the observed.
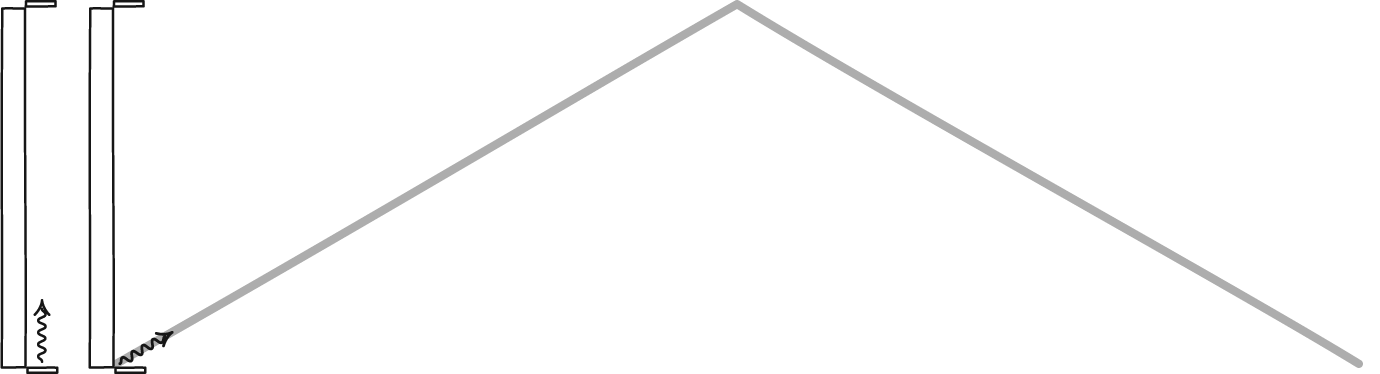
Signals in the expanding Universe
One of the most amazing discoveries of the past 100 years came in the 1920s and early 1930s: when we established that the more distant a cosmic object is located from us, the more severely its light appears to be shifted to longer and longer wavelengths. The underlying explanation is that, in the context of Einstein’s general theory of relativity, the fabric of spacetime cannot be a static structure if it’s uniformly filled with matter-and-energy, but rather must either expand or contract. Since the data indicates expansion, then expansion it is.
This realization eventually led to the modern picture of what we call the Big Bang origin of our Universe: that things started out hot, dense, and uniform, and evolved from there. As time marches forward, the following things occur:
- the Universe expands,
- masses gravitate,
- the distance between (unbound) objects grows,
- radiation has its wavelength redshifted toward longer wavelengths,
- which causes the Universe to cool,
and eventually, over time, this leads to the complex cosmic web of structure we observe today.
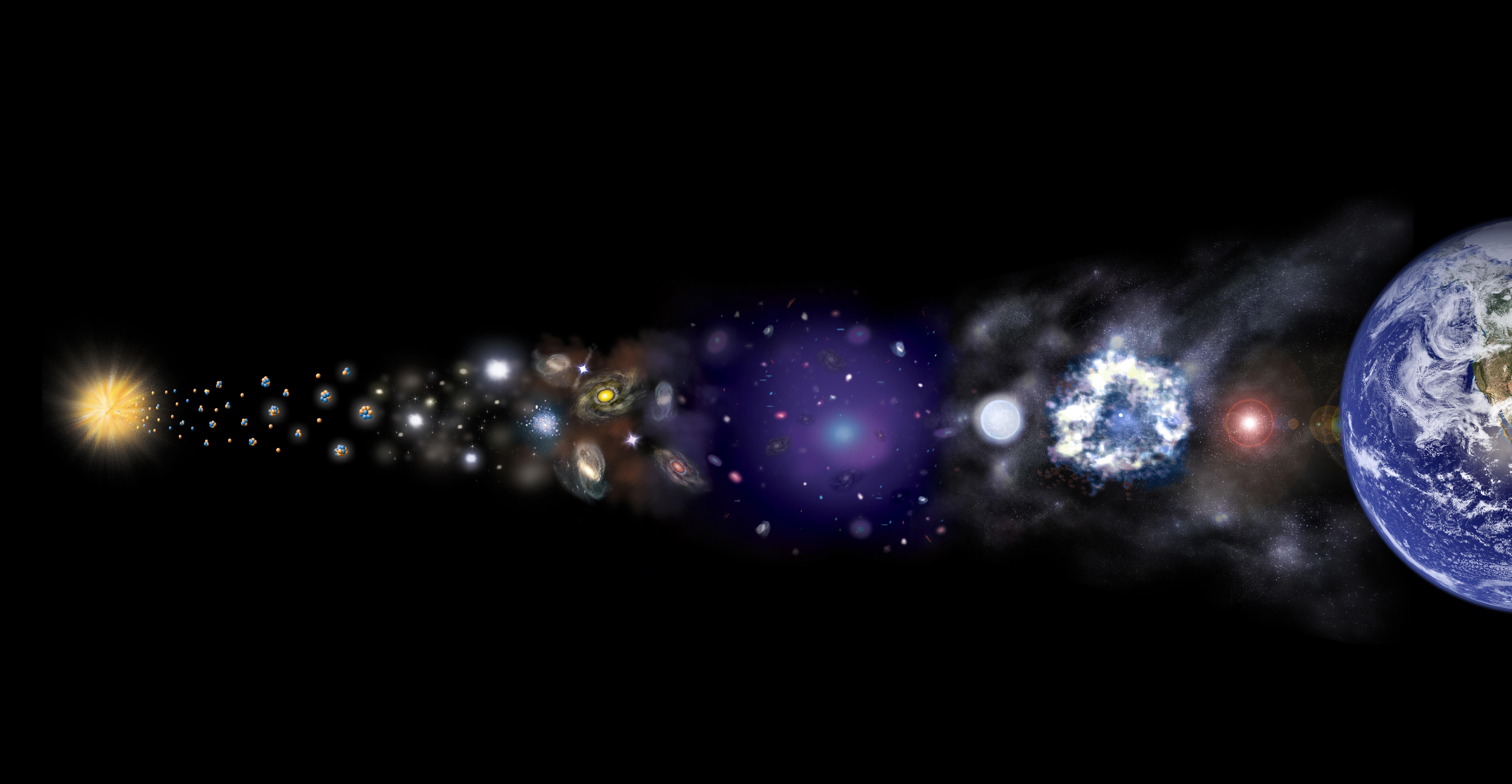
As we look to greater and greater distances, however, we have to keep in mind that we are seeing the Universe as it was long ago: closer in time toward the first moments of the hot Big Bang. Back at those earlier epochs, the fundamental constants still had the same values, the forces and interactions still had the same strengths, elementary and composite particles still had their same properties, and atoms bound into a 1-meter-long configuration still amounted to a size of one meter. Additionally, time still passed at the same rate that it always had: at one second per second.
But the light that we see from those objects, by the time it arrives at our eyes, has been journeying for a very long time through the expanding Universe. The light, as we see it, is no longer identical to the light that was emitted by the object so long ago. As the Universe expands, not only does the fabric of space itself “stretch” in some sense, but the signals that pass through it get stretched out as well. This should include the signals from every quantum of energy that traverses that space, including light, gravitational waves, and even massive particles.
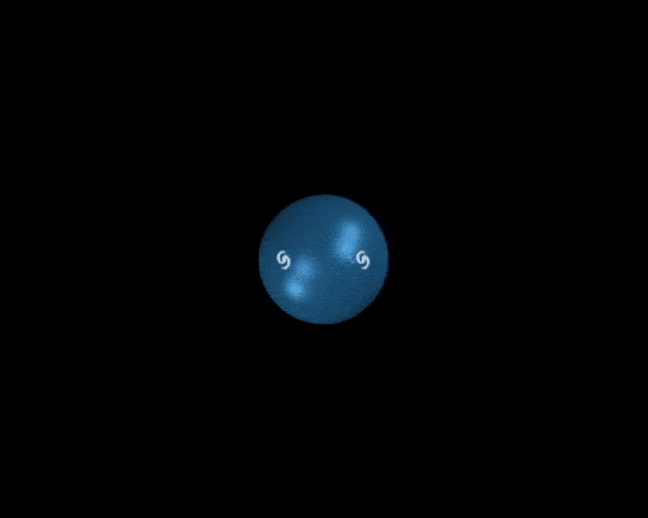
What gets “stretched” by the expanding Universe?
The signal we see, in a great many ways, is no longer the same as the signal that was emitted so long ago in the distant Universe. There are a number of effects that the expanding Universe has on what an observer eventually sees.
In analogy with the Doppler shift, which can be seen in all types of waves where the emitting source and the observer are in motion with respect to one another, we also see a cosmological redshift owing to the expansion of the Universe. Light, when it’s emitted, has a specific wavelength inherent to it. But as it travels through the Universe:
- It could either sink more deeply into a gravitational potential well, becoming more energetic and blueshifted, or could climb out of a gravitational potential well, becoming less energetic and redshifted.
- It could be observed by someone moving toward the emitting source, which would lead to that light appearing more energetic and blueshifted, or it could be observed by someone moving away from the source, which leads to that light appearing less energetic and redshifted.
- And it could be observed by someone far away across the great cosmic distances, where that light would be blueshifted by a contracting Universe, or where it would be redshifted by an expanding Universe.
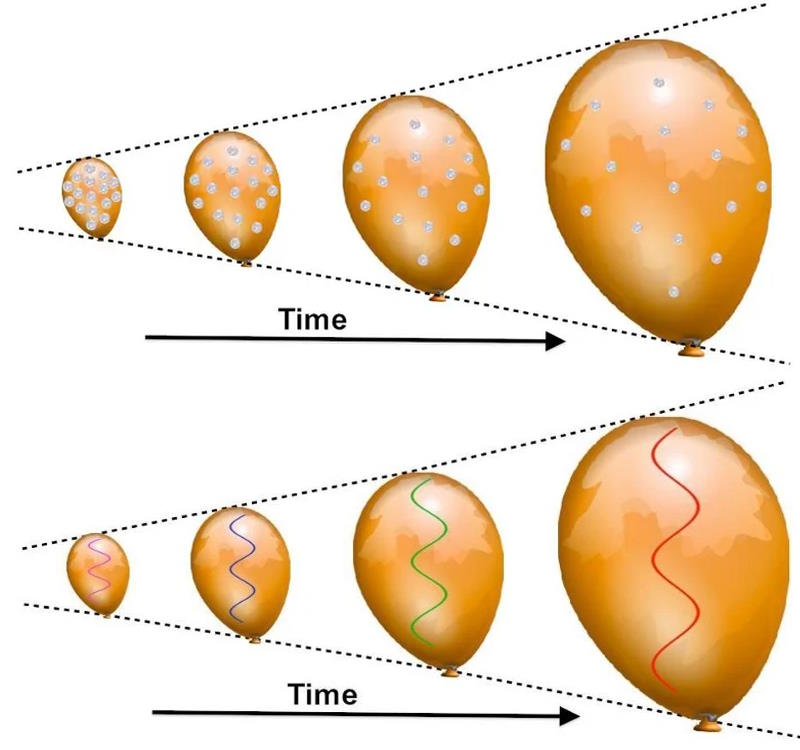
Since we’ve confirmed that our Universe is expanding, that means that light gets redshifted, or shifted to longer wavelengths and lower energies, as the Universe expands. Furthermore, the greater the amount the Universe has cumulatively expanded over the interval where that light has been propagating through the Universe from the emitter to the observer, the greater the magnitude of the redshift observed.
This doesn’t merely apply to light, either. A gravitational wave that’s emitted by any source, from merging black holes to planets orbiting stars to any masses that move in the vicinity of space that’s curved by another mass, will also be redshifted and stretched to longer wavelengths as the Universe expands.
Massive particles, as well, whether charged or neutral, will lose kinetic energy as the Universe expands. You can recover identical predictions for how much energy they use either by treating the expansion as affecting the particle’s relative velocity or by considering the dual wave/particle nature of the particle in motion and noting that its wavelength, too, gets redshifted by the expanding Universe.
Regardless of how you look at it, the wavelength of any wave that propagates through the expanding Universe gets stretched as the fabric of space also stretches, and the more the Universe expands while these waves propagate, the greater the magnitude of this effect.

But think about something for a moment: if these signals are getting redshifted, what’s happening to them?
Physically, it’s like they’re getting “stretched out.” Every quantum of light has a specific wavelength when it’s emitted, and in each second that passes, a certain number of complete waves of that wavelength get emitted.
By the time the Universe has expanded by a factor of two, the distance between each successive “crest” or “trough” of these waves will have doubled. That corresponds to what we observe as objects at a “redshift of z=1,” where the wavelength of every quantum of light we observe has been stretched by an amount equal to its original wavelength.
Whereas the source that emitted that light would have seen, say, 600,000,000,000,000 (six hundred trillion) wavelengths of that light pass them by with each second that passed (for light of a wavelength of 500 nanometers), the person observing that light will now only see half that number (three hundred trillion) wavelengths pass them by with each second that passes. Yes, the light now has a longer wavelength (of 1000 nanometers), but it also takes two seconds for the same information that was emitted over the timespan of one second to arrive at the observer.

In other words, the expanding Universe doesn’t just cause a cosmological redshift and a “stretching” of the emitted signal in terms of wavelengths, but it also causes a cosmological time dilation: a “stretching” of the emitted signal in time. This means, when we’re looking at objects that are very far away, we’re not observing them in “real-time” according to how they experienced it, but rather in slow-motion owing to this cosmological time dilation. The formula is very simple: the same “factor” that your signals get redshifted by is the “factor” by which your signals appear slowed-down when you view them.
It’s not that clocks were running slower in the early Universe; that’s not true at all. What’s true, instead, is that the expanding Universe makes the signal that we observe appear “stretched out” in time, and that applies to all of the signals we see from the distant Universe.
- We see this for distant supernovae, as measured by their light curves: the time it takes from the initial detonation until it rises to its peak brightness, and then to fall off again and fade away.
- We also see it for gravitational waves, as the gravitational waves that arrive from more distant black hole mergers have their inspiral times “stretched out” by the expansion of the Universe.
- And we even see in the temperature fluctuations imprinted in the cosmic microwave background, as these fluctuations must vary over time, but that variability is “stretched out” in time by more than a factor of 1000, explaining why we have yet to observe the “hot spots” and “cold spots” changing over the ~30 year timespan we’ve been watching them.
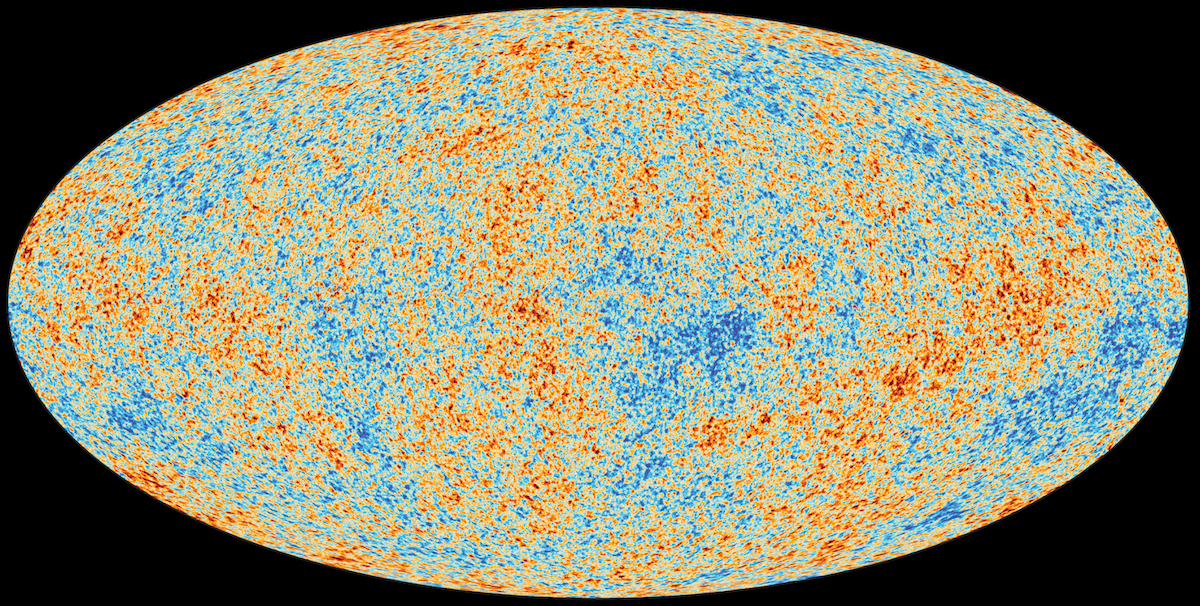
What does the new “quasar ticking” discovery actually teach us?
On July 3, 2023, scientists Geraint Lewis and Brendon Brewer published a paper in Nature Astronomy that claimed to detect this redshift-dependent time dilation in the “ticking” of quasars. Although they’re not particularly good cosmic clocks the way that millisecond pulsars are, they’re good enough clocks that, with a large enough sample of quasars, we should be able to detect a redshift-dependence to the signals that they emit.
Unlike prior studies that claimed to see no such signal and claimed to cast doubt on the interpretation of quasars as cosmic objects within the expanding Universe, this study has put those earlier claims to bed, showing that quasars do indeed exhibit this cosmic time dilation. In other words, one of the things that this study teaches us is that quasars really are cosmic objects, and they exhibit cosmic time dilation, just like everything else.
But since we can observe quasars out beyond the maximum distance we’ve ever observed an individual supernova, this also establishes a new cosmic distance record for the observed cosmological time dilation for any individual object!
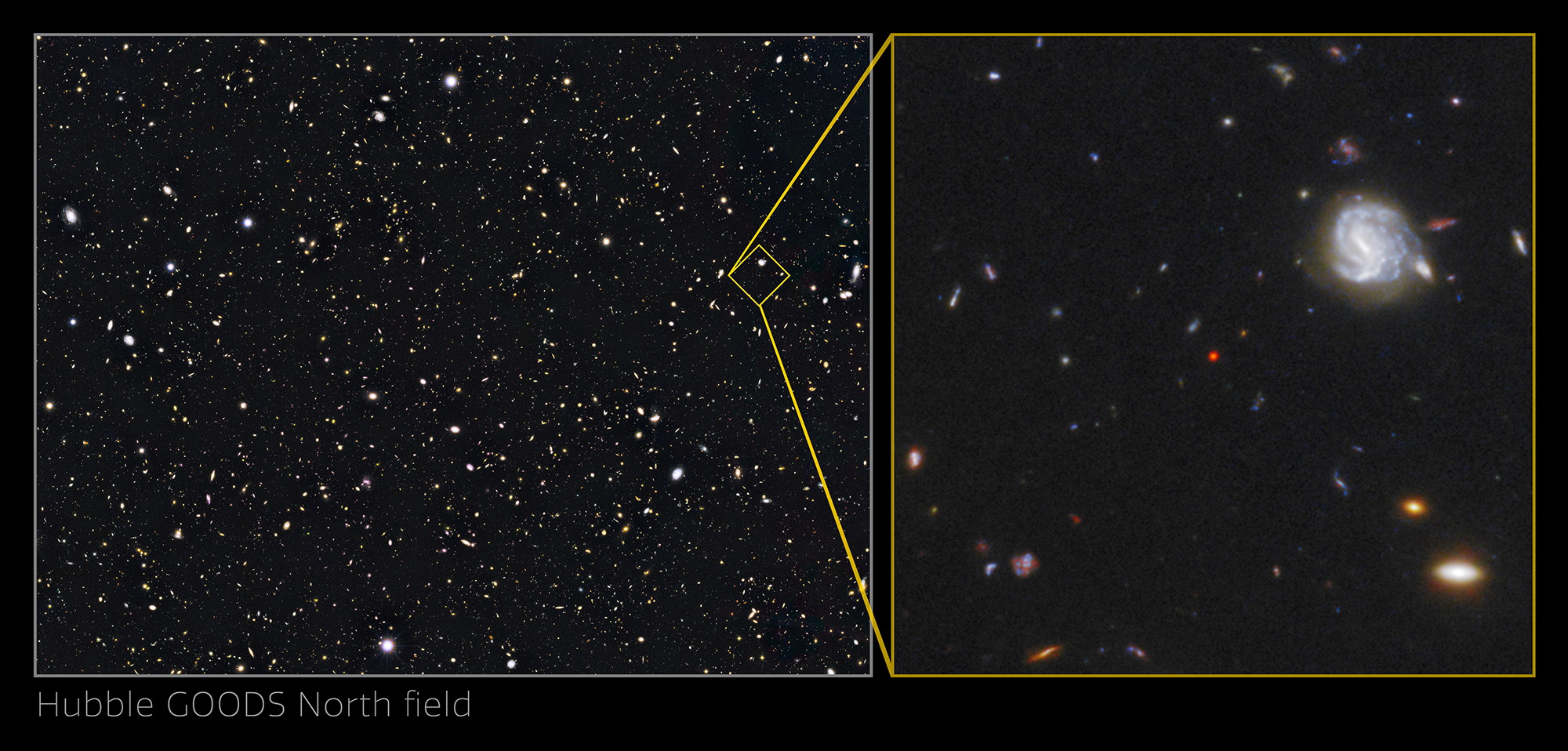
Unfortunately, a lot of people reading the stories written about this study have taken away entirely the wrong message: they now (erroneously) believe that time ran slower than it does today in the early Universe. No such thing is true! What happens is that time runs (and ran) at the same rate at all epochs throughout the Universe’s history, but that as the Universe expands, any signal that gets created gets “stretched out.” That “stretching out” occurs not only in terms of wavelength and (kinetic) energy, but also in time as well.
Time dilation has now been shown to apply in three separate instances.
- When two objects pass each other at high speeds, each one sees the other as having their clocks dilated, and time appears to pass more slowly for the other, even though each experiences time as normal.
- When two objects are in different gravitational fields, the one that’s deeper in a gravitational field experiences time passing more slowly than the one in a shallower field, and as a result, your head ages faster than your feet when you stand on Earth.
- And cosmologically, when a local observer sees a signal emitted from an object across the distant Universe, the expansion of the Universe will both stretch the wavelength of that signal and also stretch it out, in time, when we observe it.
That’s it; it’s time dilation that’s stretching out the signals from distant quasars, nothing more. But time itself always passes at the same rate for an observer anywhere in the Universe: then, now, and forever more.
Send in your Ask Ethan questions to startswithabang at gmail dot com!