Stars alone can’t explain black holes, JWST data reveals
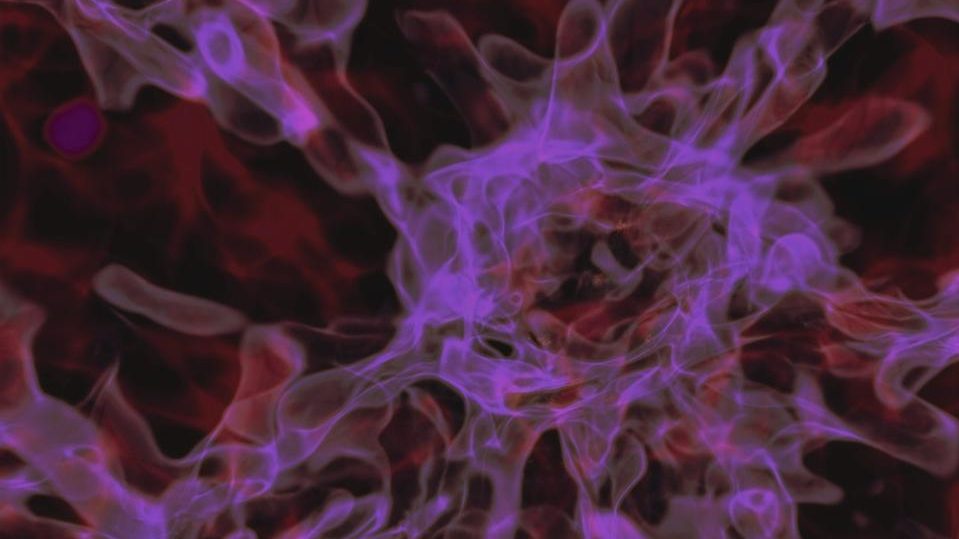
- Today, even the galaxies that house the most massive black holes have about a thousand times as much mass in the form of stars as the mass of their central, supermassive black hole.
- With the advent of JWST, however, we’re now seeing large numbers of massive, star-rich, black hole-containing galaxies early on in our cosmic history: from the first 1.5 billion years or even earlier.
- In these galaxies, their supermassive black holes are what’s known as “overmassive” for the rest of the mass of the galaxy, indicating that something other than stars must be responsible for the most massive ones. Here’s what that means.
At the center of practically every galaxy today isn’t just a collection of stars, gas, and dust, but a monster behemoth: a supermassive black hole. Ranging from millions to billions of solar masses, these cosmic monstrosities are responsible for some of the most violent, energetic events in the known Universe.
- When a star or other massive object passes too close to one, the black hole’s gravity can violently tear it apart: a tidal disruption event.
- When gas or other matter gets accreted around that black hole, the acceleration of that matter produces jets of radiation and particles: an active galactic nucleus, blazar, or quasar, depending on how we view it.
- And when another black hole merges with a supermassive one, it generates incredibly energetic gravitational waves: perhaps the most energetic events in all the cosmos.
Today, even the most massive of the known black holes represent only about 0.1% of the stellar mass of the galaxy: just one-thousandth of the amount of mass found by summing up all the stars in the galactic environment surrounding it. For a long time, astronomers have wondered just how these supermassive black holes came to be: did they form from earlier generations of stars, or was something else needed to explain them? With a large suite of new data now available owing to the advent of JWST, the answer now seems certain: stars, alone, can’t explain these black holes. Here’s the evidence that leads us to that conclusion.

The first thing we have to understand is that there are two main ways that black holes can grow:
- by the steady, gradual accretion and infall of matter, such as gas,
- and by individual events such as mergers and the swallowing of massive objects, such as stars, stellar remnants, and other black holes.
The first one, in general, is the primary method that leads to growth over time, while the second can lead to growth in leaps, jumps, and bursts, particularly when violent events such as galactic mergers occur. (And, specifically, when two supermassive black holes, one originating from each progenitor galaxy, merge together.)
When you have an accreting black hole, such as an active galactic nucleus or a quasar, there has to be a balance between the outward force/pressure of the radiation and winds that emerge from the object and the gravitational force of matter that falls inward. If there’s too much infall of matter, then there will be a rise in the amount of radiation and winds, and that will blow the infalling matter back out: a phenomenon that occurs when black hole growth proceeds at a rate that exceeds a certain theoretical limit. Because of this relation, there’s a limit to how quickly objects like black holes can grow, and hence, if we extrapolate backward in time, there’s a limit to how large an initial “seed” for these supermassive black holes must have been.
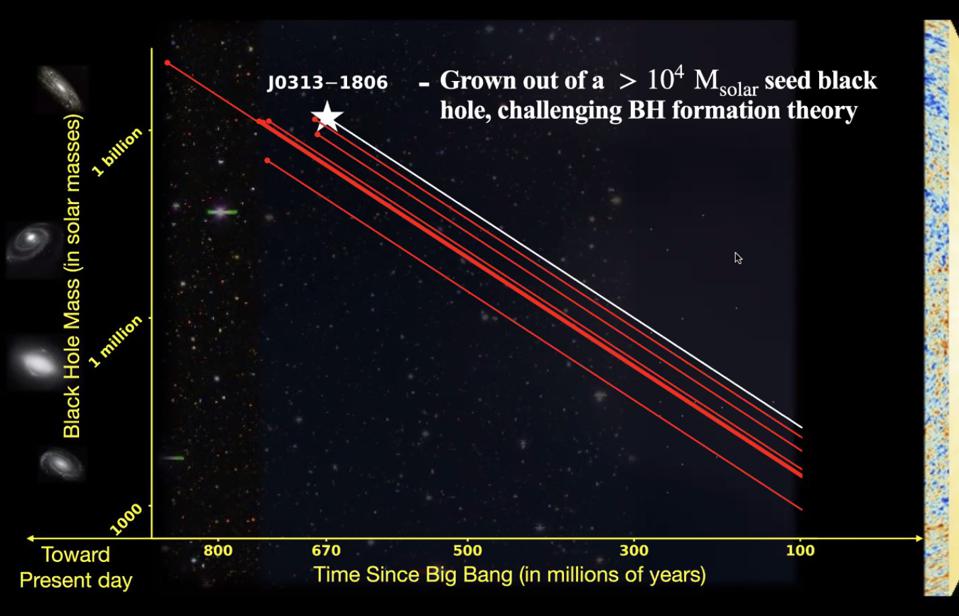
For a black hole of even tens of billions of solar masses today, that doesn’t necessarily pose any sort of problem, because today’s black holes have had 13.8 billion years of cosmic history over which they grow. But if we look back in time, we would have naively expected that the most massive black holes that we would have seen at early times would be far less massive than the enormous black holes spotted today: in the billions or even tens of billions of solar masses.
In the pre-JWST era, it was rare to find astronomical objects — things like galaxies and quasars — from the first ~1.5 billion years of the Universe’s history (beyond a redshift of z = 4, in astronomy-speak), as those objects were not only incredibly far away, but were fainter, lower in mass, and had their light severely redshifted by the expansion of the Universe. The few that we did find, if they had enough activity coming from their centers, gave enough information to infer the masses of the supermassive black holes they housed from data in the non-visible portion of the spectrum: such as at infrared or X-ray wavelengths.
Quite surprisingly, we began to find that, even before the Universe was a mere 1 billion years old, some of these black holes had masses that rivaled or even exceeded a billion solar masses.

That led to a big puzzle: how did black holes get so massive so quickly? After all, there were three major options to consider that would still be consistent with the other data we had about the Universe from observations of cosmic structure, galaxy evolution, the leftover radiation from the Big Bang, and more.
- The Universe was born without black holes, and none arose until the first stars lived-and-died, with the most massive among them leaving black hole remnants behind.
- The Universe was born without black holes, but formed them not only from stars, but from clumps of matter — such as streams of gas — that directly collapsed to form event horizons around them.
- The Universe was born with either primordial black holes or incredibly massive, overdense seeds that would swiftly collapse to create black holes, long before any stars had the opportunity to form.
The first option is a certainty: as soon as our Universe made stars, many of those first stars would die in a catastrophic supernova explosion, their cores would collapse, and many of those stars would leave a black hole behind as a remnant. Those black holes, however, would only have masses around 100 times that of the Sun. The second option is an intriguing possibility, bolstered by recent theoretical simulations by Muhammad Latif and Daniel Whalen, that could lead to more massive seeds: up to 10,000 or even perhaps 100,000 solar masses each. The third option, while exotic, would perhaps require invocation if still more massive seeds were needed.
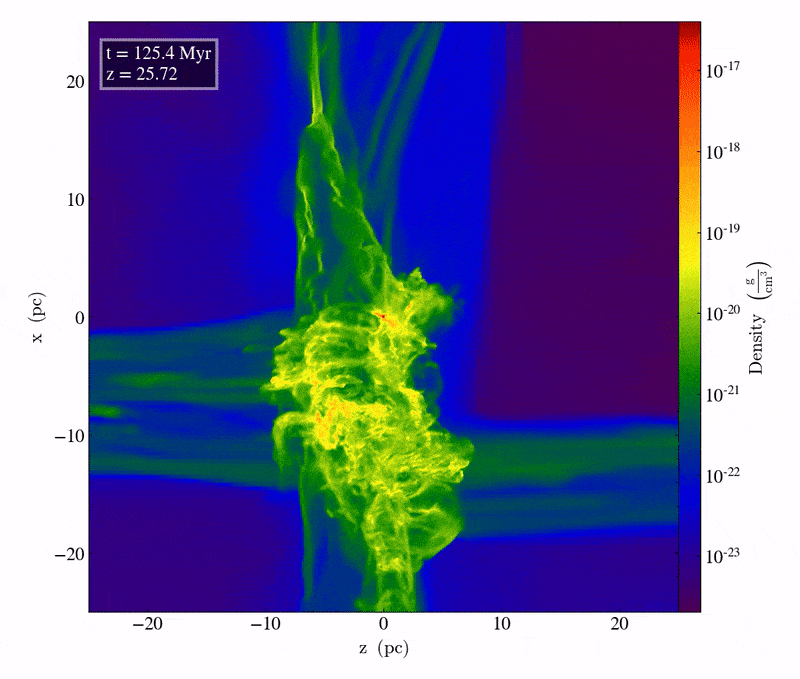
The key to uncovering the past history of the Universe, and to understanding the origin of the heaviest supermassive black holes, is not rooted in some theoretical calculations; it’s driven by observations. If we want to know how these black holes arose, and how they grew so big so quickly, there are two key classes of observations we need to make.
One is to look farther back in space and time to the earliest times in the Universe — back to galaxies from the first 1.0-1.5 billion years of cosmic history, the first 0.8-1.0 billion years, the first 0.6-0.8 billion years, and even before the first 0.6 billion years — and to measure the masses of supermassive black holes found at these early stages of cosmic history.
And the second is to take those galaxies where a black hole’s mass can be extracted and to measure the mass present in stars in those galaxies, and to see whether the relationship between the mass of the central, supermassive black hole and the mass present in stars is the same as it is at late times — in that same ration of about 1:1000 — or whether that mass ratio evolves toward more equal values.
With both of those pieces of information, we should be able to tell whether the black hole-stellar mass ratio remains constant, which would point to a “stellar seed” origin for these black holes, or whether there are an abundance of what we call overmassive black holes: where the black holes that are observed would need to have grown from something more massive than the black hole left behind by a star.
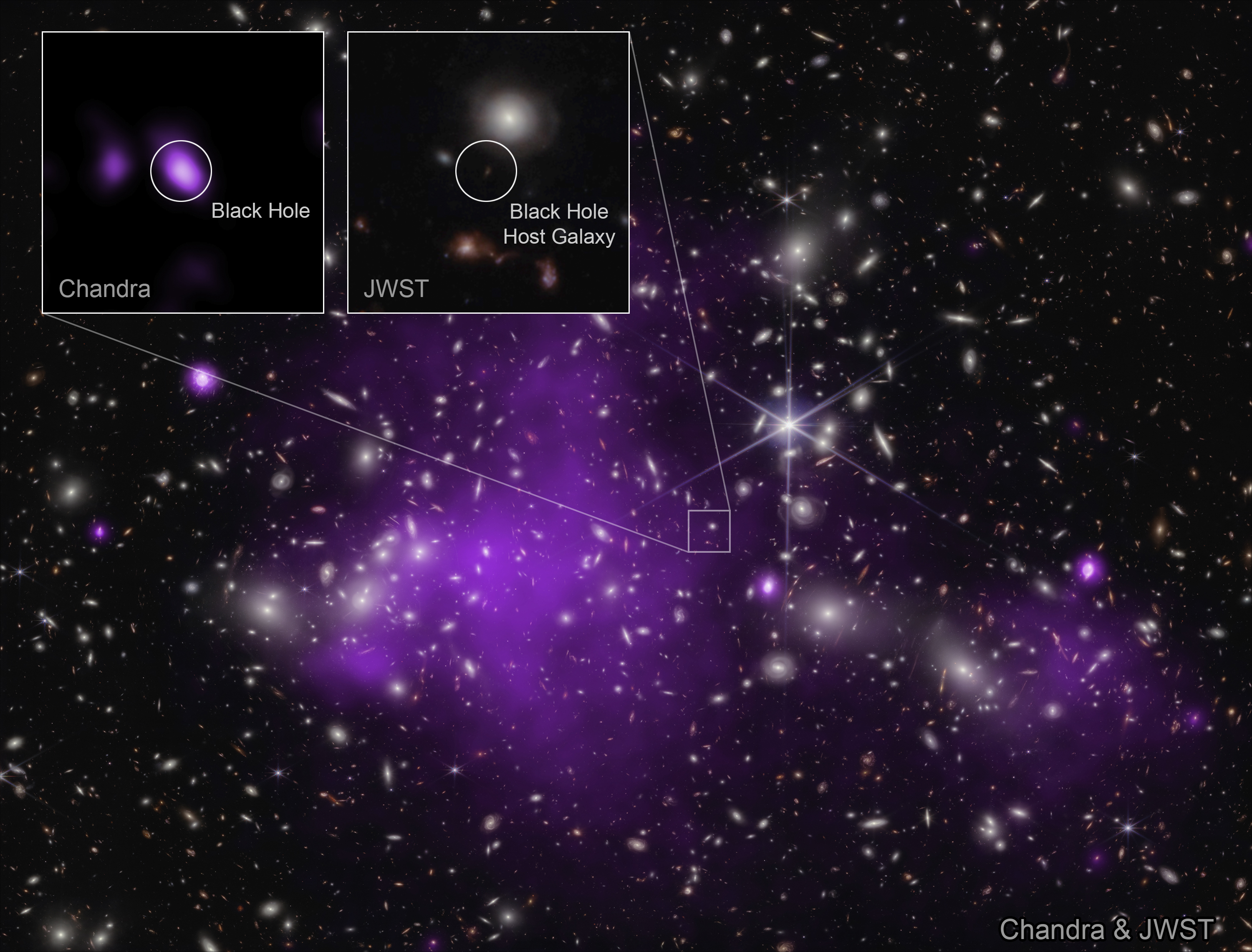
Now that we’re in the JWST era, we’ve discovered our first black holes from when the Universe was less than 600 million (0.6 billion) years of age: from when the Universe was just 4.3% of its current age or younger. In fact, we now have three detected black holes from that era.
- The supermassive black hole in CEERS 1019, which has a mass of around 9 million solar masses: about 1% of the total stellar mass at that time, which comes from around 570 million years after the Big Bang.
- The supermassive black hole UHZ1, which comes from just 470 million years after the Big Bang: although its mass is uncertain, between 10-100 million solar masses, the stellar mass within that galaxy is comparable: also between 10-100 million solar masses, implying the black hole-stellar mass ratio is at least 10%, and could be even 100% or more.
- And the most distant black hole yet detected, in the galaxy GN-z11, comes in at right around 2-3 million solar masses, but has an impressive ~1 billion solar masses worth of stars inside of it. The black hole-stellar mass ratio is only 0.2-0.3%, and comes from 440 million years after the Big Bang.
However, these are only a few data points, and the less data you have, the less reliable your results are going to be, especially when drawing conclusions about the entire Universe.
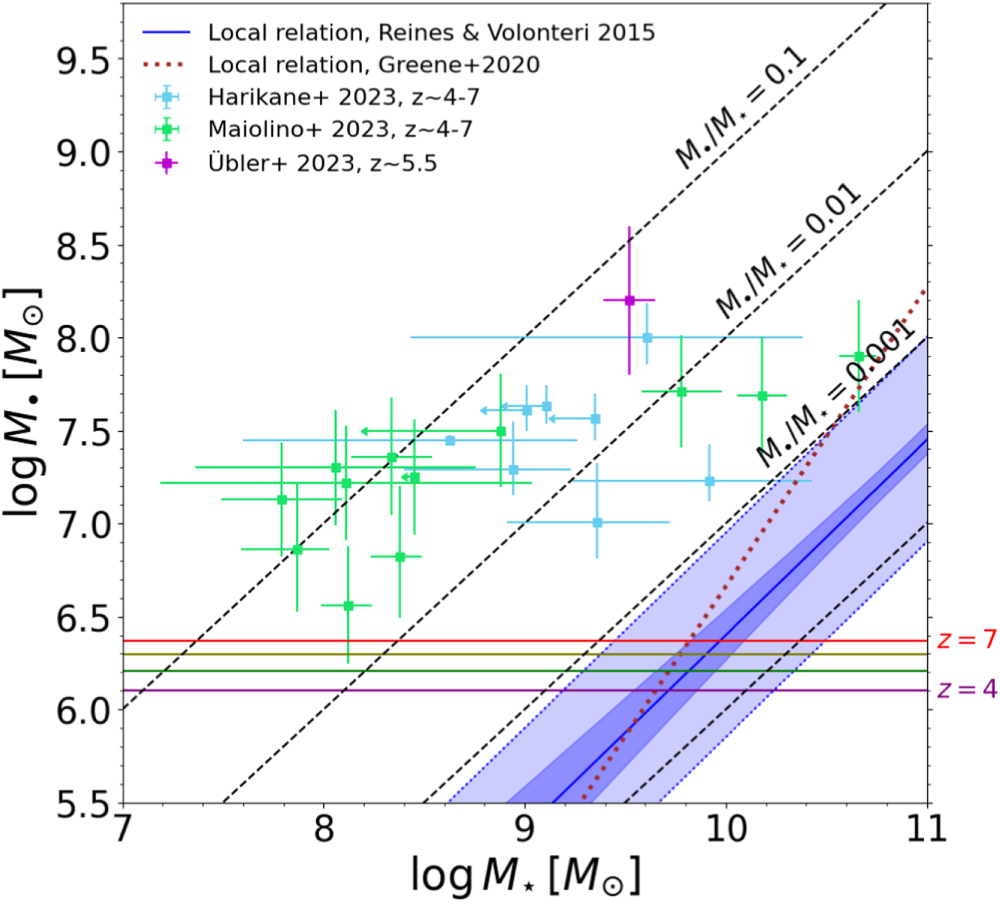
Thankfully, in a new paper published in the Astrophysical Journal Letters, lead author Fabio Pacucci was able to identify an enormous number of galaxies — primarily from JWST’s CEERS and JADES surveys — that allow us to extract estimates for both the stellar masses of the host galaxies and also reliable estimates (with quantified uncertainties) for the masses of the central, supermassive black holes housed inside of them.
Today, there are big, massive galaxies like Messier 87: the central galaxy of the impressive Virgo Cluster of galaxies. There are over a trillion stars inside of it, containing a total stellar mass of between 2-and-3 trillion solar masses. It also has the largest supermassive black hole known in our vicinity: of about 6.5 billion solar masses. The black hole-stellar mass ratio of 0.2% is fairly typical of galaxies with the biggest black holes in them: around that 1-to-1000 ratio.
On the other hand, there are also galaxies with more modest supermassive black holes. Andromeda, the largest galaxy in the Local Group, has more like a 1-to-10,000 ratio. Our own galaxy, the Milky Way, has more like a 1-to-100,000 ratio, with a black hole of just ~4.3 million solar masses despite having around ~400 billion solar masses worth of stars. Finding this fainter, lower-mass population will be difficult in the early Universe, so we might expect to find only the biggest, brightest examples at early times: consistent with that 1-to-1000 ratio.
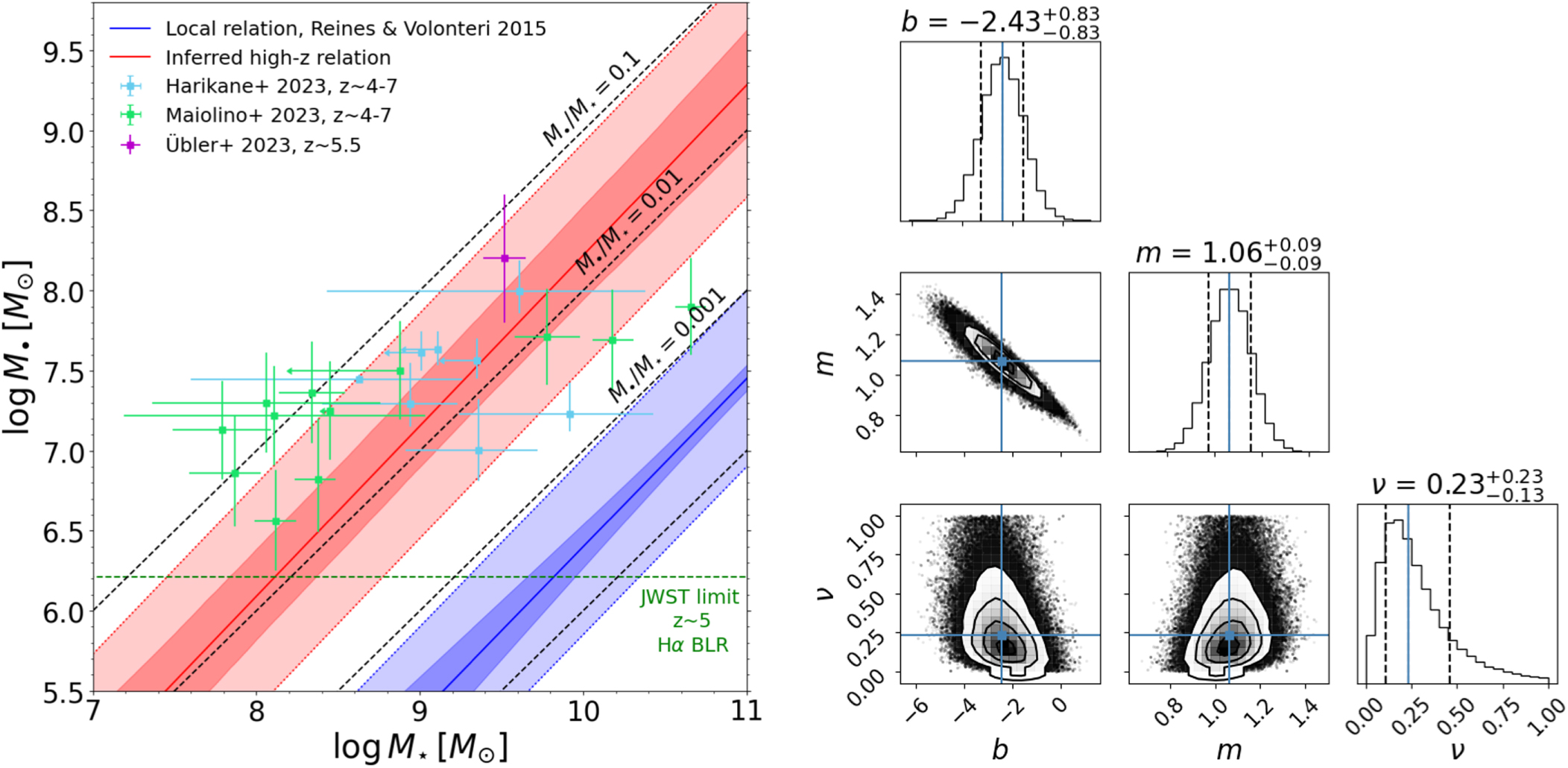
But that isn’t what Pacucci’s study, using the most comprehensive JWST data available at the start of 2024, found at all. Instead of a 1-to-1000 ratio, they found that, overall, there was more like a 1-to-10 or 1-to-100 ratio: where black holes were ten-to-a-hundred times as massive, compared to their galaxy’s stellar masses, as the modern-day counterparts that we find today. Even if we restrict ourselves to the most extreme supermassive black holes known today, we are seeing that early on, black holes were much more overmassive back then as compared to now.
This has a lot of implications on a lot of fronts, but the biggest one is this: seeing black holes that are more massive early on, as compared to the stellar masses we find in the galaxies that house them at those early times, is strong evidence that — at least in these galaxies — the black holes that we are seeing did not arise from the stars themselves, but rather from more massive seeds than stars, alone, can provide. The fact that these black holes are “only” a factor of 10, 100, or even 1000 times larger than they are at present suggests that they didn’t form from stars, but rather from the direct collapse process of clouds of normal matter, further suggesting that invoking something exotic, like primordial black holes, is unnecessary.
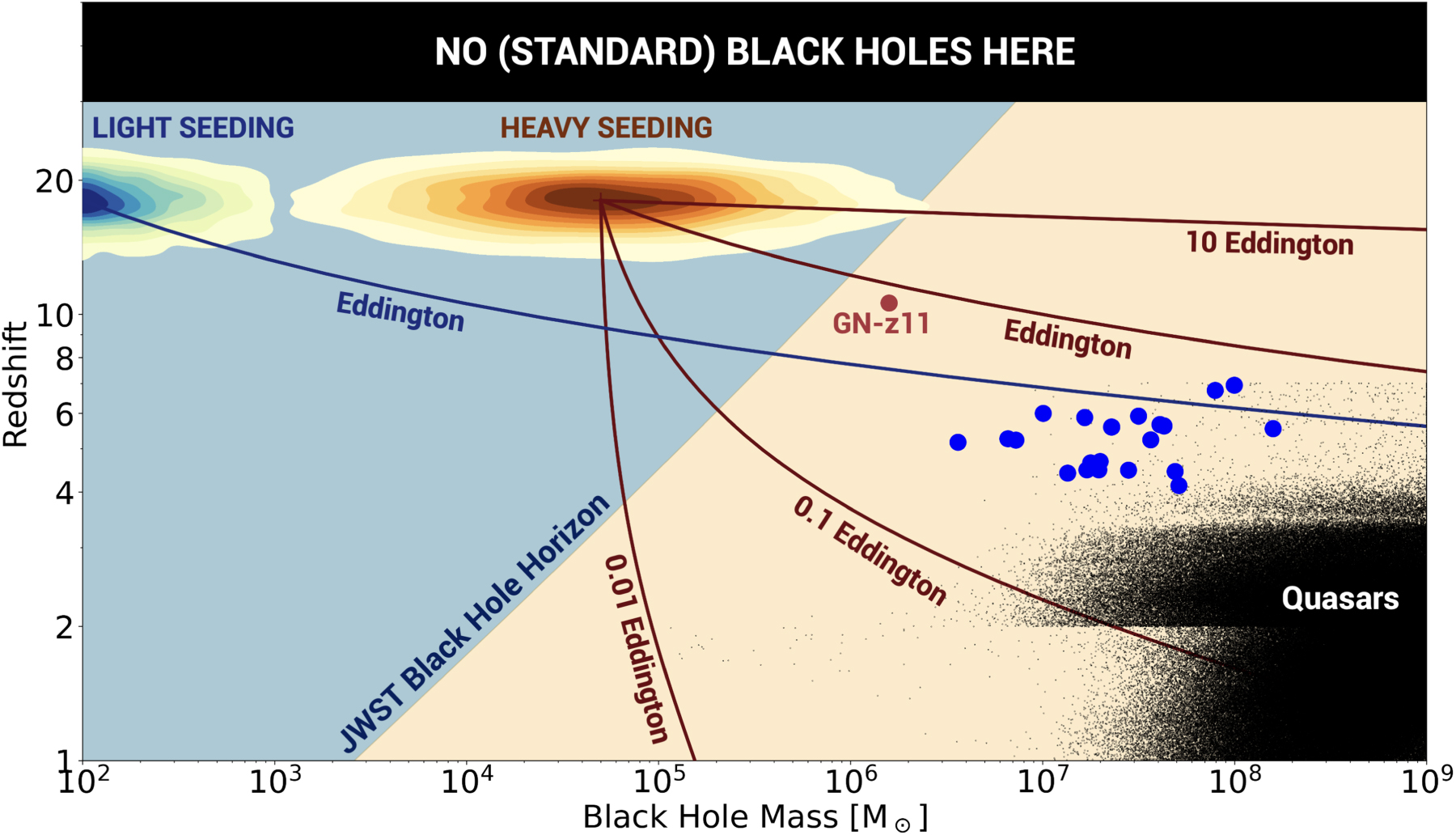
This new study, synthesizing large amounts of JWST data from the first 1.5 billion years of cosmic history, reveals a large population of overmassive black holes. Whereas the identification of UHZ1, the first severely overmassive black hole, provided evidence for heavy black hole seeds that were formed by direct collapse, the current study both strengthens the evidence for that scenario and also shows that this scenario may be the norm for giving rise to the most massive black holes of all in the modern-day Universe.
This scenario was theoretically predicted back in the 2010s, and to see the evidence coming in for it today is vindication for the astronomers and astrophysicists who pushed for this once-controversial scenario back in the days when data was sparse. The fact is that we can now confirm that stars, alone, are unable to explain the origins of all of the supermassive black holes that we find in the Universe, and that some other mechanism — a mechanism that is consistent with what’s predicted by direct collapse scenarios — is required to give rise to the ones we find early on.
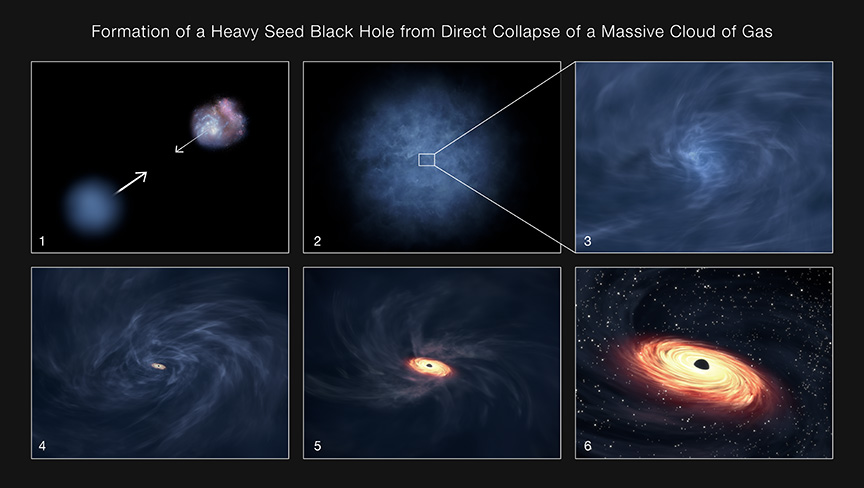
We still don’t know whether there are other, smaller black holes out there that have yet to be revealed, particularly among the faintest, lowest-mass galaxies that strain the limits of what JWST can detect. There could yet be multiple populations of galaxies with black holes: some of which were formed by direct collapse, and others that formed from the remnants of the first stars, as we are only beginning to take a census of these objects.
However, the era of claiming that nothing more than stars — living and dying as normal, with some of the most massive ones having their cores collapse down to black holes — are required to give rise to the supermassive black holes we find at later times is now over. Something more is required: perhaps it’s direct collapse, which is the leading suspect, or perhaps it’s something even more exotic. Perhaps stars can still explain many of the supermassive black holes that arise in the Universe, or perhaps stars are only a sub-dominant effect, and that direct collapse is responsible for the majority of supermassive black holes in the Universe. With the JWST era now in full swing, the good news is, we’re likely to soon find out.