Most distant black hole raises a huge cosmic question
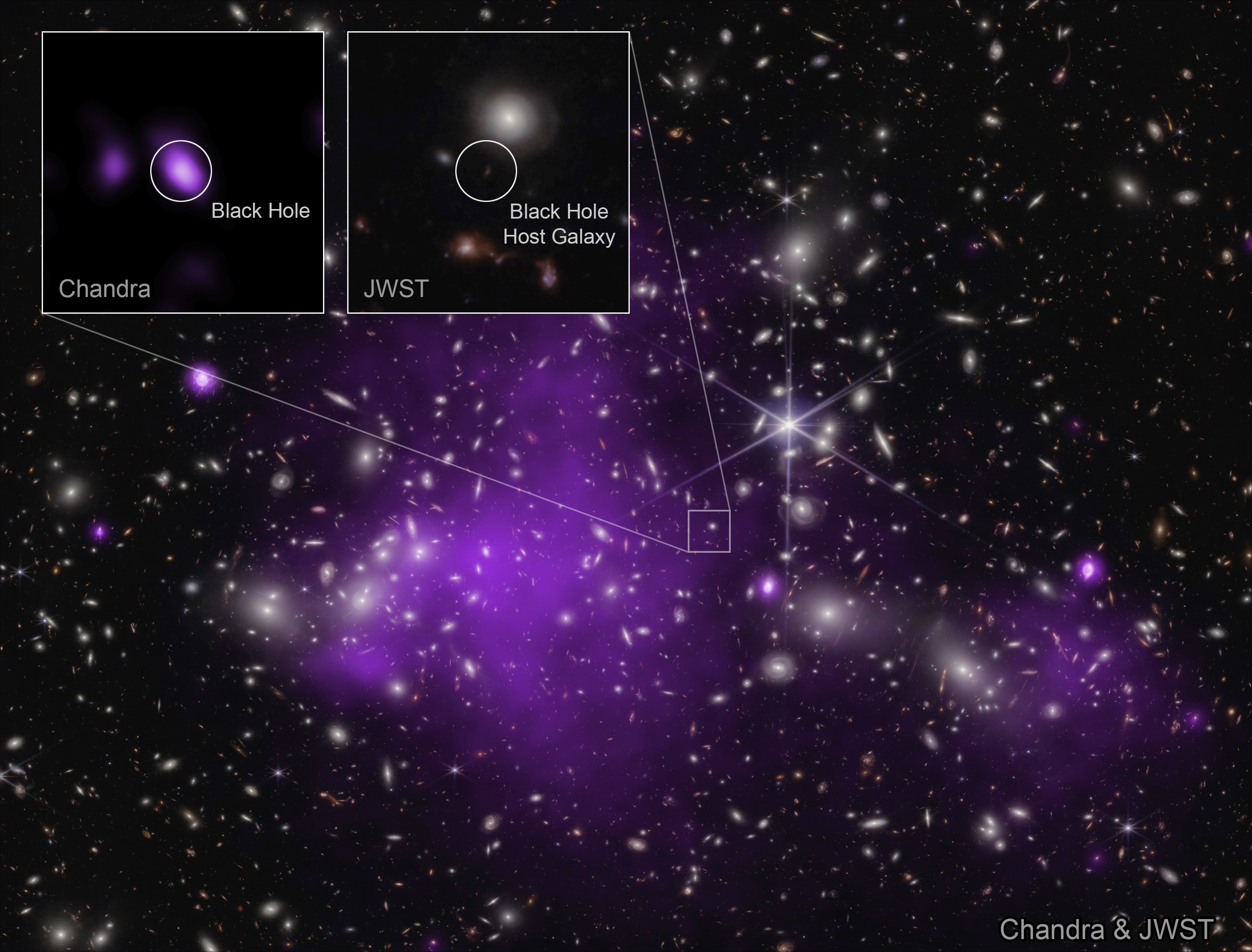
- Prior to the JWST era, which only began in mid-2022, the earliest black hole ever seen was already found 700 million years after the Big Bang: when several billion stars had already formed in the largest galaxies.
- In June of 2023, CEERS 1019, discovered by JWST, broke the cosmic record by more than 100 million years. But in November of 2023, that record fell once again, thanks to JWST, Chandra, and gravitational lensing.
- This X-ray emitting supermassive black hole is centered within a relatively tiny galaxy, raising the tremendously important question: how did this black hole get so massive inside such a low-mass environment?
Although black holes are the most extreme objects in the Universe — with more mass confined inside a tiny volume of space than anyplace else — they’re also poorly understood in a number of important ways. While we know that when very massive stars die, their cores collapse and that can lead to the formation of black holes, we know there must be other ways to form them:
- from merging neutron stars,
- from stars that (somehow) spontaneously collapse,
- and perhaps even from larger structures, like streams of gas, that never go through a stellar phase at all, but simply directly collapse to make black holes.
It may even be possible, although there are some compelling physics reasons to think not, that the Universe was born with primordial black holes: a population of black holes that sprang into existence long before the very first stars ever formed. These scenarios are particularly important when it comes to explaining the existence of the earliest supermassive black holes ever seen, as many of them are what we call “overmassive” or “outsized,” where it’s very difficult to explain how they got so massive, so quickly, especially considering the environments they’re in.
With the newest cosmic record breaker — black hole UHZ1, the most distant ever discovered — the cosmic mystery just deepened in an unexpected way. Here’s why scientists are fascinated.

Starting in the late 1950s, we began observing energetic sources in the Universe that didn’t match any known class of object. As we moved beyond optical astronomy and started exploring the Universe in other wavelengths, new types of objects began to emerge. With the advent of radio astronomy, certain sources appeared to emit large amounts of radiation with either no visible-light counterpart or simply a single, unresolvable point of light. These quickly became known as either QSRS (for quasi-stellar radio sources) or QSOs (for quasi-stellar objects), which were both eventually revealed to be the same class of object: quasars.
When we began looking at these objects in X-ray wavelengths of light as well, we found that they weren’t just bright and energetic at those wavelengths as well, but that they often varied rapidly at X-ray wavelengths. With improved telescopes, optical counterparts began to be identified: these objects turned out to be galaxies, although often faint and ultra-distant. Then in 1971, the first anomalous X-ray source within our galaxy, Cygnus X-1, was identified. We soon came to understand that we were looking at two examples of the same type of object: a black hole. Moreover, these weren’t isolated black holes, but black holes that were actively feeding on some sort of matter, accelerating it, and causing the emission of radiation of a wide variety of frequencies and wavelengths.

We now know that black holes exist with all sorts of properties (like masses and rotation rates) and in many different locations (including within our own galaxy, as part of multi-star systems, at the centers of most galaxies, and even flying through the intergalactic medium). The ones at the centers of galaxies are particularly interesting, because they’re not of comparable masses to heavy stars, but rather rise up into mass ranges spanning millions, billions, or even tens of billions of solar masses. At the center of the Milky Way, a four million solar mass behemoth lurks, and we’ve even reached the technological point where we can observe individual stars near the galactic center orbiting a point that emits no optical light at all.
We can be extra certain that these objects are, in fact, black holes, because we’ve directly imaged their event horizons in radio wavelengths of light. The black hole at the center of nearby, active galaxy Messier 87 (above) was the first one ever imaged, which is much more massive than the one at the heart of the Milky Way: at 6.5 billion solar masses. However, we’ve observed the Milky Way’s central black hole directly as well, showing us that the objects that look like black holes and act like black holes really are black holes, with properties consistent with everything we think black holes should and shouldn’t be able to do.

Alongside these sets of observations, we also have extraordinary X-ray data that comes to us from numerous space telescopes, including NASA’s Chandra X-ray observatory: a cosmic workhorse that’s celebrating its 24th anniversary this year. Despite being a relatively small observatory in terms of its physical size, Chandra has incredible angular resolution owing to the extremely small wavelengths of X-ray light. It’s sensitive to many high-energy phenomena, including:
- hot gas,
- energetic plasmas,
- pulsing neutron stars,
- and the matter accelerated by black holes.
It has witnessed activity from our own Milky Way’s black hole, and helped us find stellar mass black holes that would be otherwise invisible to our arrays of observatories on Earth and in space. In particular, it’s even taken deep-field images of certain regions of the sky that don’t have a lot of (light-absorbing) hydrogen gas in them, revealing enormous numbers of what can only be very distant, supermassive black holes, all at the centers of presently active galaxies. In one long-exposure region of sky, shown below, Chandra discovered more than 300 supermassive black holes in an area spanning barely a tenth-of-a-square-degree in the sky.
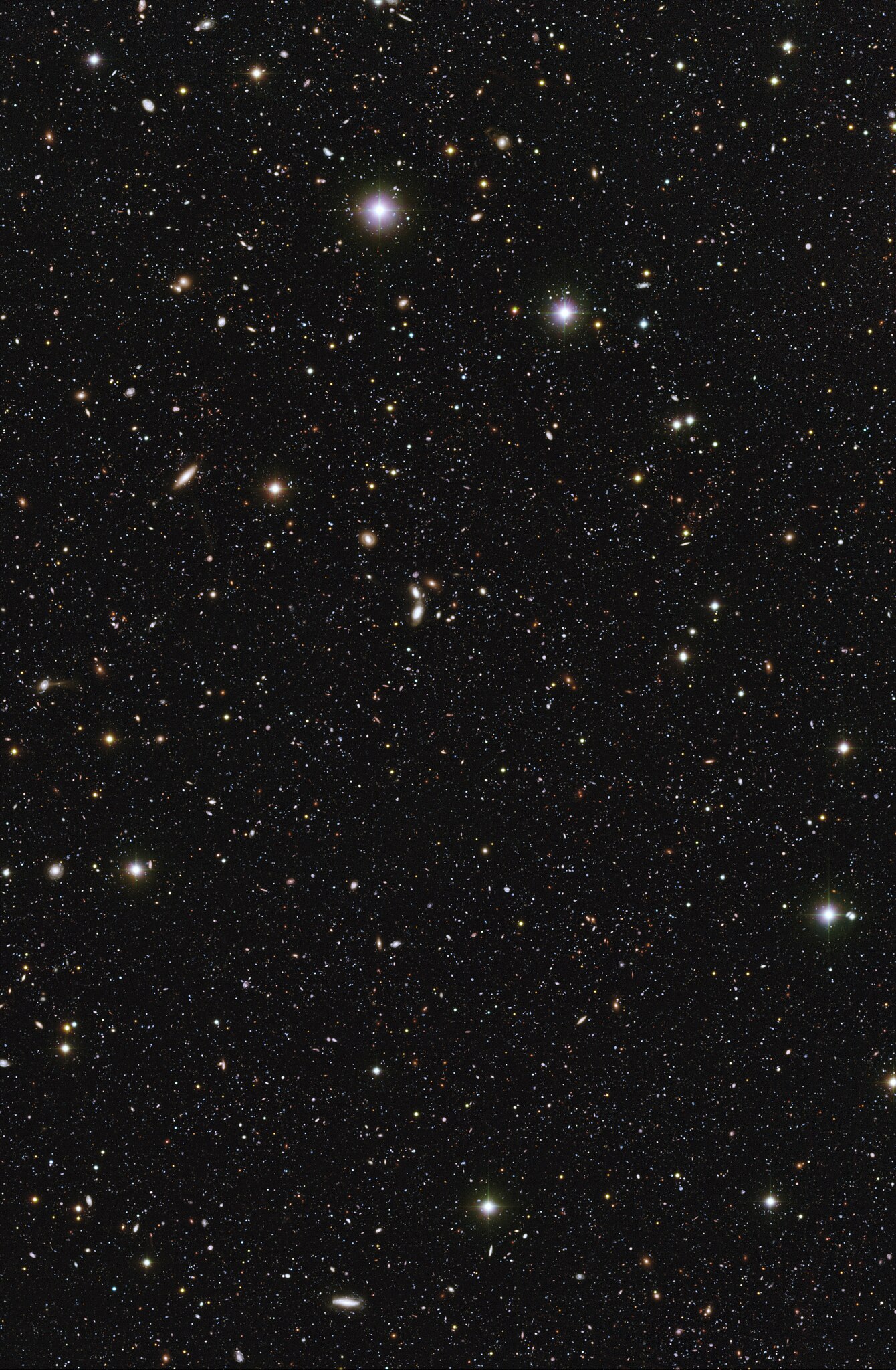
Meanwhile, in infrared wavelengths of sky — also excellent for identifying black holes — NASA’s Spitzer Space Telescope similarly discovered enormous numbers of supermassive black holes. Now that Spitzer has been superseded by arguably our most ambitious space telescope of all time, the JWST, the hunt for the earliest and most massive black holes has gotten an additional boost.
Prior to the JWST era, a large number of quasars and active galaxies had been discovered: not just in the late-time, well-evolved Universe, but also at very great distances, which correspond to very early times in the young Universe. Many black holes that had already grown to several hundred million or even a billion or more solar masses — black holes that are hundreds of times the mass of the Milky Way’s central and most massive black hole — have been found from time periods that correspond to the Universe being less than a billion years of age since the hot Big Bang.
These early, but still very massive, black holes have posed an interesting puzzle for astronomers: if the only black holes that we can form come from stars, and stars top out at a few hundred solar masses, then how did these supermassive black holes, in such a short period of time, grow from such small seeds to be so massive so quickly?
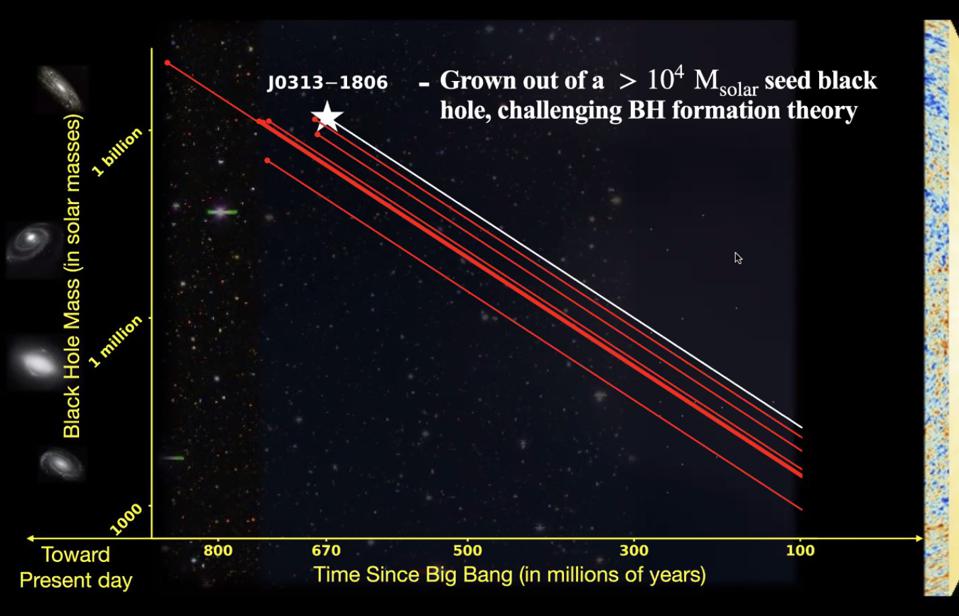
This is where we had hoped that JWST would come in and help us resolve the puzzle. After all, it’s pretty clear that:
- if you require stars to make black holes,
- and the black holes that you make from stars top out at a couple of hundred solar masses,
- and black holes have a maximum rate (the Eddington rate) at which they can grow by accreting matter,
then our standard calculations for how big black holes should get, based on known physics, fails to give us predictions that align with what we’ve already observed. Something, somewhere, must be wrong about our assumptions, models, or calculations.
Numerous possibilities abound. It could be that the Universe was born with black holes before any stars formed at all: a population of primordial black holes. However, there are many theoretical and observational reasons to disfavor these scenarios; primordial black holes would necessitate new physics. It could be that the first stars were more massive than current stars, as they were less efficient at cooling-and-collapsing, meaning that they require more initial mass to collapse from. However, models indicate that even with initial seed black hole masses of ~1000 solar masses or so, which might be reasonable estimates for the first stars, these black holes simply grow up to be too big, too fast for this, alone, to solve the puzzle.
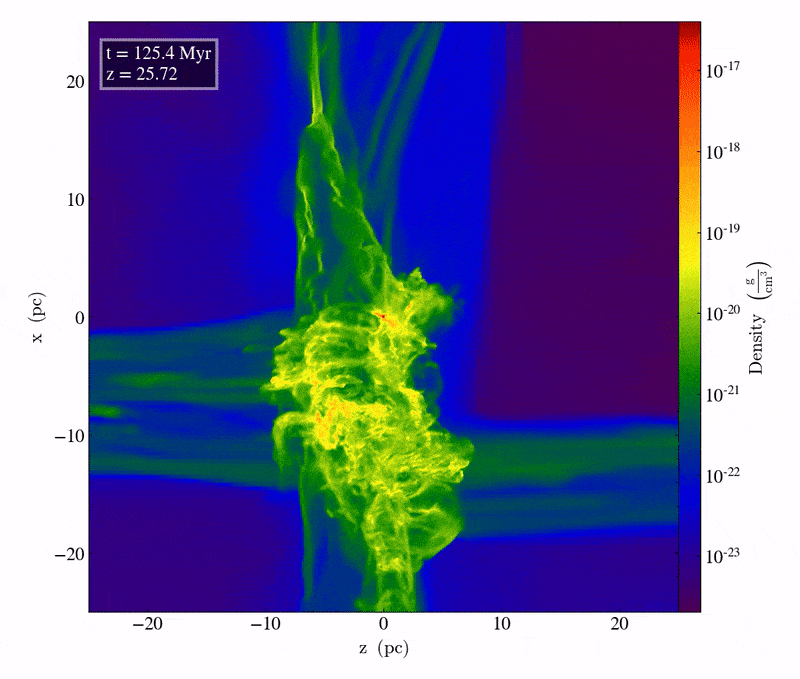
One fascinating possibility, put forth only in 2022, suggested that overdense clumps of matter — clumps that might be as massive as several tens-of-thousands of solar masses — might directly collapse to form black holes, just as some stars seem to wink out of existence with no cataclysm, presumably forming black holes spontaneously. These strong, cold accretion flows of matter can trigger the rapid collapse of dense clouds of matter, perhaps creating “seed” black holes up to the 30,000-40,000 solar mass range: massive enough that, if these seeds were formed when the Universe was just 100-200 million years old, we could finally put this puzzle to rest.
That’s where the JWST comes in. Whenever you look at the Universe with new, superior tools, the only thing you should be certain of is that you’ll find something, and maybe multiple things, that surprise you. In July of 2023, JWST researchers from the CEERS collaboration reported the discovery of CEERS 1019: a black hole of 9 million solar masses coming from when the Universe was just 570 million years old, breaking the record for most distant black hole. So long as this black hole represented no more than ~1% of the total mass of the galaxy (which it did), the Universe should have no problem forming black holes this massive this early.
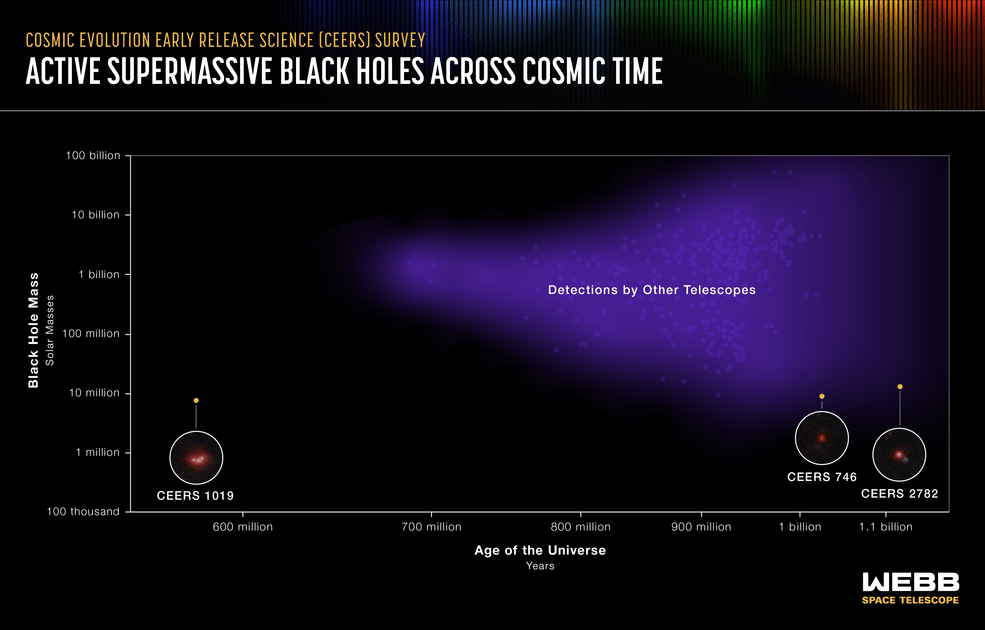
However, if we were to discover what’s known as an “overmassive” or “outsized” black hole, where the supermassive black hole is actually comparable to the total mass of the galaxy, then that would present a challenge. After all, how could a black hole, which requires a continuous source of mass to grow at the maximum rate possible, grow to be so massive that it’s comparable to the mass of the galaxy that houses it?
And yet, that’s precisely what makes this latest discovery — a new record-breaker for most distant black hole, known as UHZ1 — so compelling. When JWST looked at Pandora’s cluster, also known as Abell 2744, it discovered a great many galaxies involved in a cosmic smash-up, as well as many gravitationally lensed background galaxies, whose light was distorted and magnified by the cumulative mass of the foreground galaxy cluster. With Chandra X-ray data overlaid atop it, one object simply jumped out at astronomers. Known as UHZ1, it:
- comes to us from when the Universe was just 470 million years old, or 3% of its present age,
- contains a black hole that’s somewhere between 10 million and 100 million solar masses (from the brightness of the X-rays, as seen by Chandra),
- but where the total stellar mass of the galaxy (from JWST observations) is also only between 10-100 million solar masses.
This one discovery, in concert with everything else we’ve learned to date, teaches us a gigantic lesson.
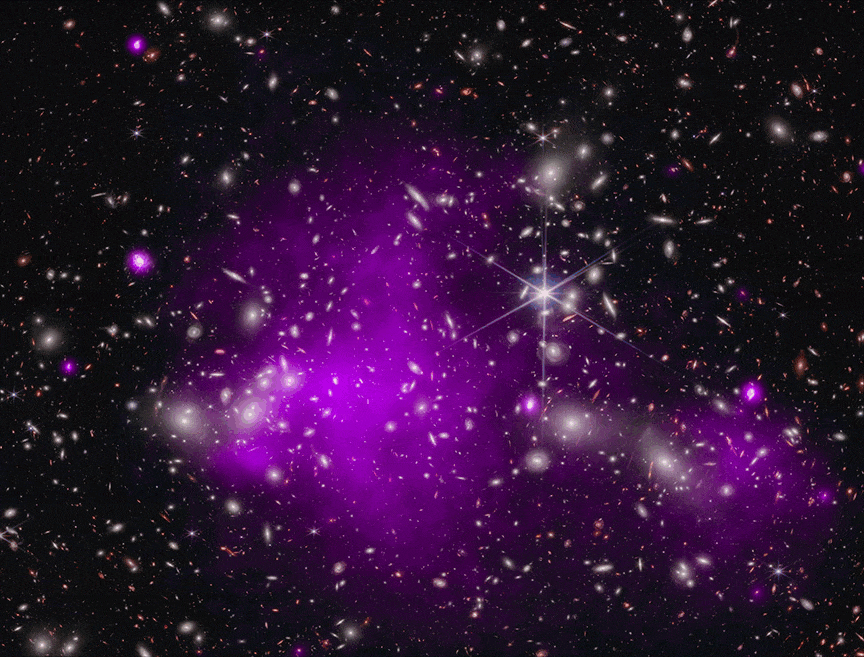
For one, it tells us that the “small seed” scenario, where supermassive black holes grow from stellar-mass seeds, cannot account for UHZ1. There’s an upper limit to how quickly black holes can grow, and that’s in part determined by their environment. With so little mass available within its host galaxy, UHZ1 couldn’t have reached 10-100 million solar masses in just a few hundred million years if it began from such a small seed. As Andy Goulding, lead author of the study that reports the galaxy’s distance and mass, put it:
“There are physical limits on how quickly black holes can grow once they’ve formed, but ones that are born more massive have a head start. It’s like planting a sapling, which takes less time to grow into a full-size tree than if you started with only a seed.”
But a large black hole “seed,” between 10,000 and 100,000 solar masses, could have formed from directly collapsing gas, and then grown by consuming the matter that falls onto it. As matter falls onto the black hole, it will form stars and also grow the black hole, with theoretical predictions indicating that over a few hundred million years:
- the black hole “seed” will start off outmassing the stars around it,
- then both the mass of the black hole and the mass of the surrounding stars will rise,
- but with the mass in stars rising more quickly,
- causing the stellar mass to eventually catch up to, and then surpass, the mass of the more slowly-growing central black hole.
Up until UHZ1, we had never found a black hole more massive or as massive as the cumulative amount of stars that surround it. But we’d also never found a black hole as early in cosmic history as UHZ1, either; this is a critical piece of evidence in revealing our cosmic history!
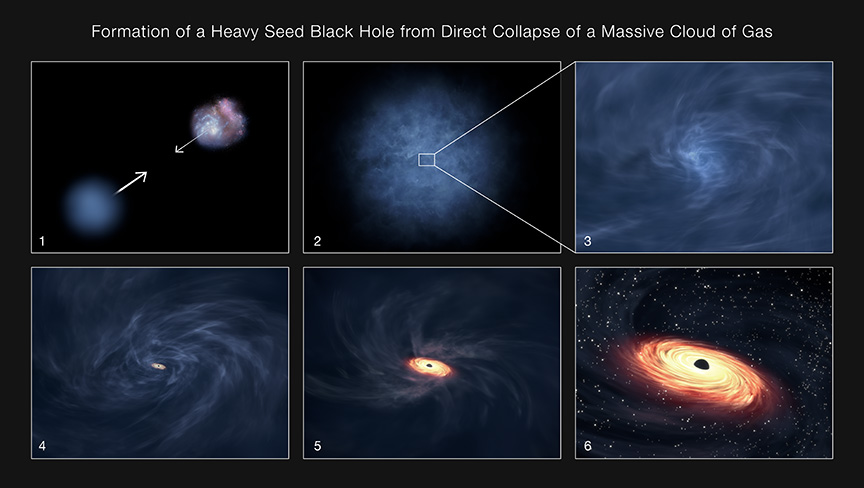
If we put all the pieces together, we arrive at a scenario where sure, the first stars will definitely give rise to large numbers of black holes, up to hundreds or perhaps even 1000 solar masses each. But the seeds of supermassive black holes were more likely formed from the direct collapse of gas, leading to black holes of tens of thousands or maybe even 100,000 solar masses, appearing just as the very first stars are only igniting. Then, as gas accretes and collapses, those black holes grow but the stellar mass in galaxies rises more quickly, with stars “catching up” to the black hole in mass after a few hundred million years, and then surpassing it just a few hundred million years later.
It doesn’t require us to assume any novel or exotic physics (like primordial black holes), but rather to accept that black holes can indeed form from the direct collapse of matter: creating large “seed” black holes of at least tens-of-thousands of solar masses, independent of any stars that form, live, die, and create black holes themselves. This observation also teaches us that with JWST, Chandra, and the power of gravitational lensing enhancing all types of light from distant, background objects, we can fully expect that large numbers of these early black holes — even ones in young galaxies with relatively small numbers of stars — will eventually be found, allowing us to test the details of this emerging picture of how galaxies and black holes are connected. Perhaps this longstanding cosmic question, of how black holes got so massive so quickly, is finally nearing a satisfying resolution.