All of spacetime is rippling with gravitational waves
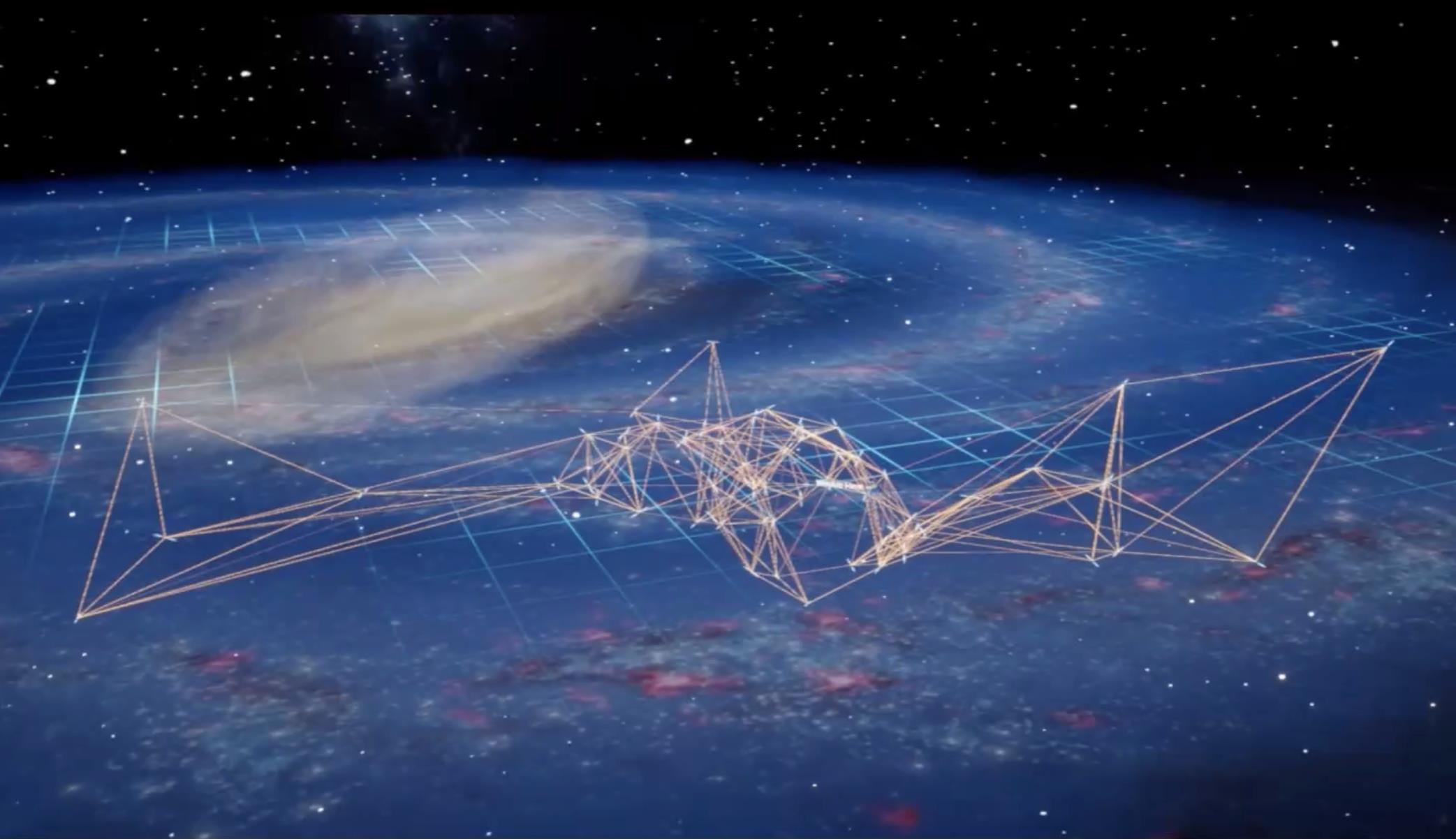
- At long last, we have a second way to directly detect gravitational waves: by leveraging the timing variations of millisecond pulsars throughout the Milky Way.
- For the first time, we’ve seen robust evidence for the background gravitational wave “hum” of the Universe.
- The NANOGrav collaboration’s data suggestively points to that background being caused by pairs of supermassive black holes in “death spirals” with one another, and future observations should reveal their nature definitively.
From all across the Universe, planets, stars, stellar remnants, and other massive objects are locked in an intricate but inherently unstable gravitational dance. Each and every mass curves the fabric of spacetime in their respective vicinity, while every other mass moves through a path determined by that curved spacetime. But that simple act — of one mass moving through the space that’s curved by another mass — is inherently an unstable one, as gravitational masses moving through a gravitational field undergo a radiation reaction, mandating that they emit gravitational radiation, or gravitational waves.
For 100 years since General Relativity was put forth, these gravitational waves went undetected, until the LIGO scientific collaboration detected them from low-mass black holes (a few hundred solar masses or below) in the final stages of their inspiral and merger. In the time since that first 2015 detection, about 100 other gravitational wave signals were detected, but all in the same end-stages of an inspiral and merger.
For the first time, a new class of gravitational wave signals has been seen in an entirely different way: by scientists monitoring the timing of the most accurate natural clocks in the Universe, millisecond pulsars. In a tour-de-force series of papers, the NANOGrav collaboration presents strong, compelling evidence for a detectable gravitational wave background on timescales ~10 billion times longer than LIGO is capable of seeing. It marks the first direct detection of this cosmic gravitational wave background, and the next steps will be even more exciting.

First off, it cannot be overstated what a huge success it is to see these gravitational waves. One of the remarkable predictions of General Relativity was that, unlike in Newton’s gravity, gravitationally bound systems aren’t stable forever. Under Newton’s laws, if you put any two masses in the Universe in orbit around one another, they would each make the shape of a closed ellipse, returning to the same point over and over again with every orbit, with that orbit never decaying, but remaining eternally stable.
Not so in General Relativity. Under Einstein’s theory of gravity, any two masses orbiting one another cannot do so forever, as the way that spacetime curves absolutely forbids it. Over time, these masses will radiate energy away in the form of gravitational waves, gradually causing them to inspiral in toward one another as their orbits decay. Eventually, if you wait for a sufficiently long time, enough energy will be lost that these masses will:
- move closer together,
- into tighter orbits,
- where they move even more quickly,
- emitting gravitational waves of a higher frequency (shorter period) and greater amplitude,
- and so on and so on,
- until they eventually merge together.
In Einstein’s Universe, which as far as we’ve ever been able to measure, is the best description of our Universe, every system is unstable in this fashion. Even if the Sun and Earth were to live forever exactly as they are now, the Earth would inspiral and merge into the Sun after ~1026 years went by.
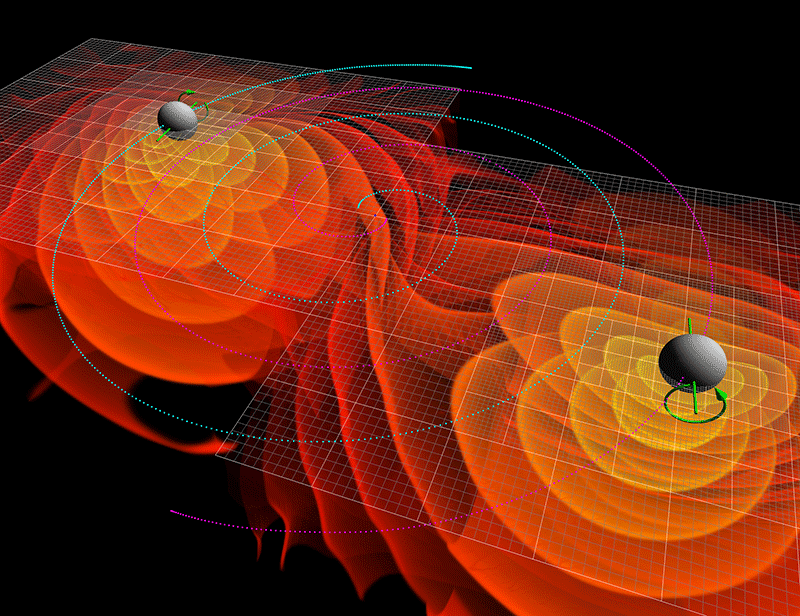
There were hints that this type of orbital decay, along with the necessarily associated gravitational wave emission, occurred even before we measured the first gravitational waves directly. That hint came from a type of object known as millisecond pulsars: the Universe’s most precise natural clocks. A pulsar is a neutron star with an incredibly strong magnetic field: billions to quadrillions of times as powerful at the surface of the neutron star as the magnetic field is here on the surface of our own planet. Pulsars have both a rotational axis and an offset magnetic axis, and so each time they spin around, they “beam” a brief blip of light at every object that happens to coincide with where its magnetic axis points.
Not every neutron star is a pulsar, but we don’t yet know if that’s because not every neutron star pulses or merely because most neutron stars don’t have their magnetic axis “point at us” as they rotate. Of the observed pulsars, most are young and/or rotate only slowly. But as they age, they’re known to spin up, so there is a population of very old pulsars that spin with a period of 1-10 milliseconds, pulsing 100 or more times every second. These millisecond pulsars are the most accurate natural clocks in the Universe, and can keep time to within about ~1 microsecond over a time period of decades.
In the second half of the 20th century, we discovered our first binary pulsar system: where a pulsar orbits another stellar-mass object. Lo and behold, its orbit, based on its pulse timing, was observed to decay, exactly in line with General Relativity’s predictions.
Since (gravitational potential) energy was being lost as the orbit decayed, something must have been carrying that energy away, and gravitational waves were really the only option. That was one of the primary motivations for building terrestrial gravitational wave detectors, like LIGO and Virgo, to detect the final stages of these inspirals and mergers directly. From 2015 — when the first bona fide detection took place — until the present, that was the only method ever used to successfully, directly observe these gravitational waves.
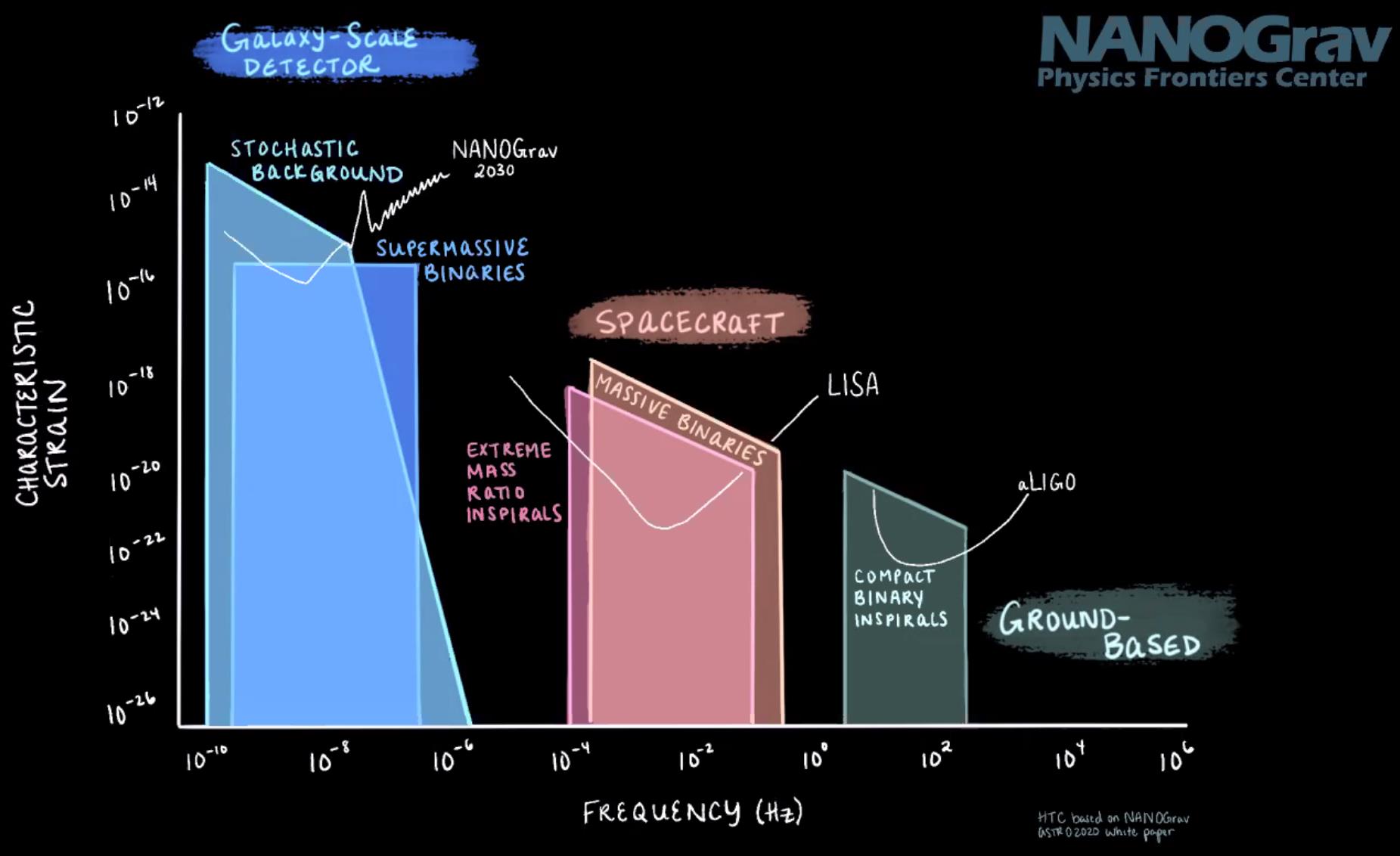
Today, June 28, 2023 (or June 29 in some parts of the world), is the day that all changes.
Gravitational waves are emitted by all orbiting objects all throughout the Universe, with tight orbits producing high-frequency (short period) gravitational waves and wider orbits producing lower-frequency (long period) gravitational waves. Whereas LIGO uses laser arms that are a few kilometers long and is sensitive to gravitational waves with periods that are a fraction-of-a-second long, other teams of gravitational wave hunters use the known millisecond pulsars from across the Milky Way, separated by thousands of light-years. By observing them all together and looking at the timing differences between pairs of pulsars, they can measure gravitational waves with periods of years or even a decade. After a herculean 15-year effort, the NANOGrav collaboration has finally collected enough data from enough millisecond pulsars to conclude that, at last, yes: spacetime itself is full of the ripples from these gravitational waves, and we’re confidently seeing them for the first time.
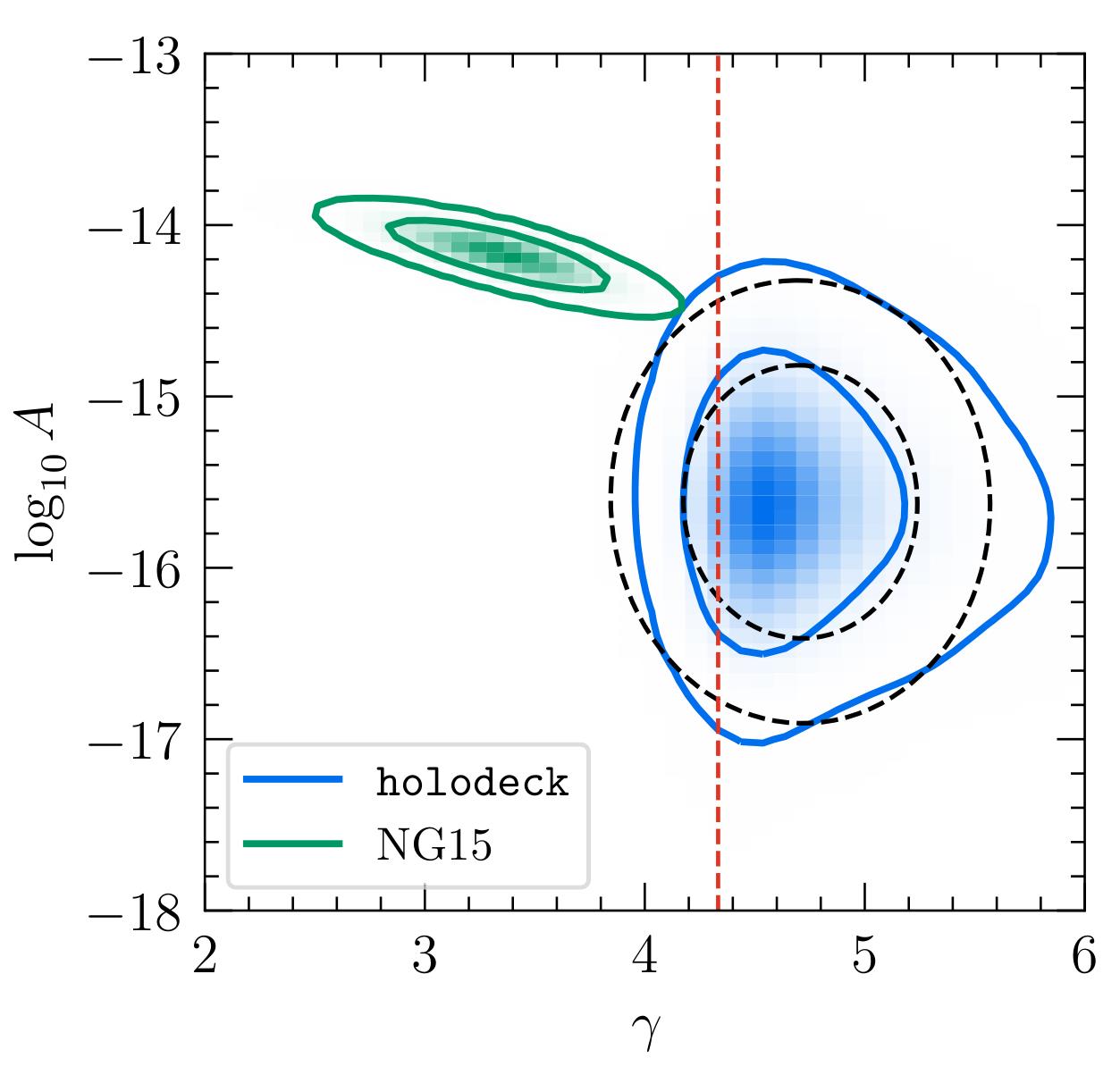
Most of us, when we picture space, likely do so the way Newton did: as some type of three-dimensional grid. When Einstein’s General Relativity came onto the scene, his theory showed three flaws with the Newtonian picture, although only the first two were commonly realized at first.
- Viewing space as a three-dimensional system with a set of coordinates placed on top of it was fine, but the choice-of-coordinates is arbitrary, and will be viewed differently by every observer at a unique location within our four-dimensional spacetime and with a unique motion through that space. There are no “absolute” coordinates that are better-or-worse than any other set of coordinates; they’re all relative to each specific observer, including where they are and how they move.
- The structure of space itself is not flat, grid-like, and cartesian, which is how Newton conceived of space. Instead, that space is curved, and can flow “into” or “out of” regions of the Universe depending on whether that portion of the Universe is either expanding or contracting. As one of the 20th century’s greatest minds in General Relativity, John Wheeler, once put it, “Spacetime tells matter [and energy] how to move, and matter [and energy], in turn, tells spacetime how to curve.”
- And that superimposed atop that curved spacetime with a unique structure relative to each and every observer is the full suite of all the gravitational waves propagating through spacetime at the speed of light: from all directions. Being at a point in spacetime is like being atop an unsteady ocean, as you feel the cumulative effects of all the waves generated by all of the ocean sources all at once. Except, in spacetime, it’s the cosmic ocean that generates these waves, and all the forms of matter and energy within our visible Universe.

At all frequencies, there’s a “hum” to our Universe generated by all of the gravitational waves put together. Occasionally, at the final stages of an inspiral or merger, one particular gravitational wave voice — from one binary system made of two masses — stands out above the background chorus, shouting with a rising pitch that culminates in a cacophonous “chirp,” which is precisely what Earth-based gravitational wave observatories like LIGO are measuring for stellar mass black holes and neutron stars, and what the space-based LISA (Laser Interferometer Space Antenna) will observe for supermassive black holes that devour other masses that are substantial enough.
But that “background hum” is there at all frequencies, and, importantly, is produced by all masses orbiting one another in the Universe. This is true for:
- planets orbiting stars,
- stars that are members of multi-star systems,
- stellar remnants and their systems,
- stars and stellar remnants moving within galaxies,
- galaxies that merge together,
- and supermassive black holes along with everything that orbits them.
Based on our best modern understanding of our Universe, we can model-and-calculate the expected magnitude of the gravitational wave background at all frequencies. If we ever get to the appropriate sensitivity levels, at any such frequency, we’ll be able to detect the existence of this background. And if we can get even more sensitive than that, we should be able to tease out the nature of the signals contributing to this background, determining what’s actually creating these gravitational waves permeating our cosmos.
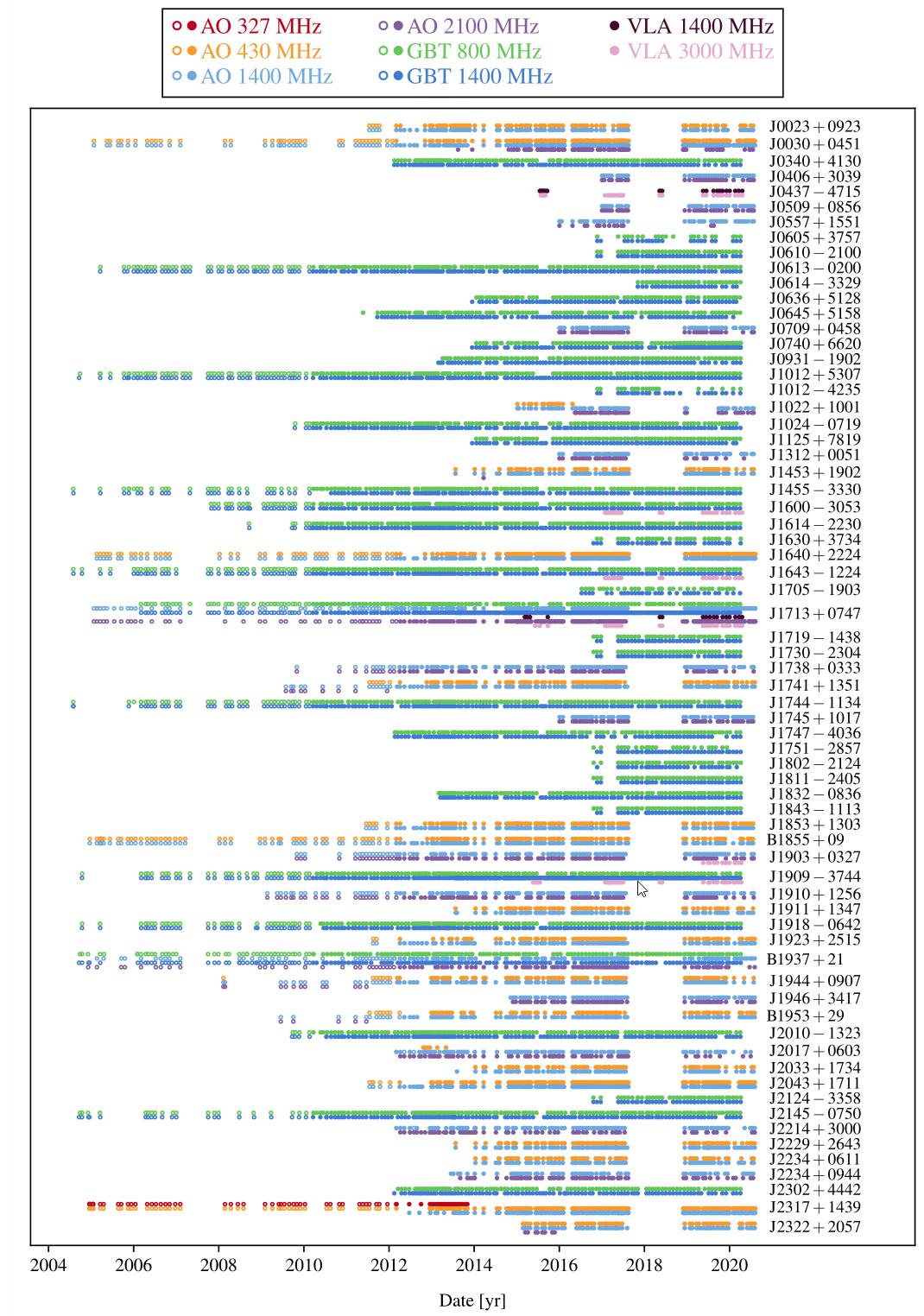
That’s the big news announced by the NANOGrav collaboration, which synthesizes pulsar timing data from scores of millisecond pulsars observed across all of North America. (There are other pulsar timing arrays as well, including Europe’s EPTA, India’s InPTA, China’s CPTA, Australia’s Parkes Pulsar Timing Array, and the international effort seeking to synthesize them all: the IPTA.) Over the past 15 years, NANOGrav has:
- increased the number of pulsars they’ve observed, from an initial 14 up to 68 today and with more than 80 looking ahead,
- increased the number of telescopes and telescope arrays observing these pulsars (with the notable exception of the recently collapsed Arecibo observatory),
- increased the types of frequency bands over which each individual pulsar can be observed (ranging from a low of 327 MHz to a high of 3.0 GHz),
- increased the baseline time for which these pulsars have been observed (just publishing their 15-year data set),
- and, as a result of all of these, increasing the signal-to-noise ratio of their data in an effort to uncover this background hum.
At last, for the first time, they’ve gotten there. They have enough high-quality data to see good evidence for the existence of this background hum, which (according to theory) is predicted to arise, at these frequencies, primarily from pairs of supermassive black holes found at the centers of post-merger galaxies.
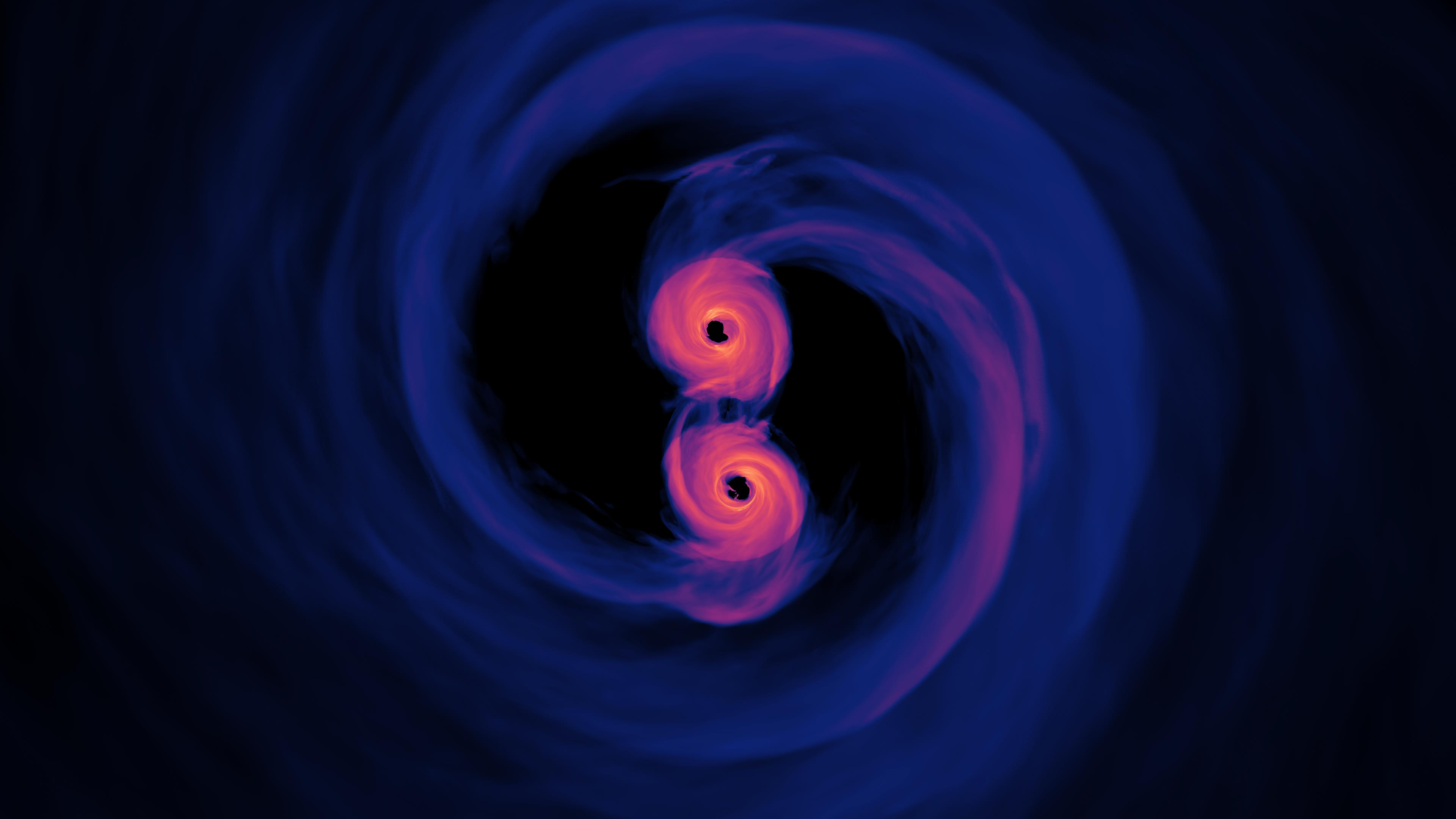
The way they did this was not to look at the absolute timing measurements of any of these pulsars individually, but rather to correlate the timing data from all pairs of pulsars (i.e., to look at all possible combinations of the timing variations seen between any two pulsars, together) and to see how their signals were varying: in phase or out-of-phase, with a positive or negative correlation, in a frequency-dependent or frequency-independent way, etc.
Different signals should generate different types of correlations, and so the NANOGrav collaboration tested what they were seeing, which definitively looks like it “isn’t just noise” according to the data, against different sets of predictions.
- They see no evidence that these gravitational waves were generated from inflation at the start of the early Universe, which is good, because if the signals from those gravitational waves were so large that they appeared at these sensitivities, it would challenge what we think we know about the origin of the Universe.
- They see no evidence for exotic physics: bizarre phase transitions, primordial black holes, or cosmological defects among them.
- They also see no evidence for chirping, which would arise if we had ultramassive (perhaps even too massive for conventional physics to explain) black hole binaries merging together.
But even though there isn’t a sufficient signal, yet, to determine what these gravitational waves are, we’re seeing something, and it looks like that thing is most consistent with the signal theorists expected: binary supermassive black holes.
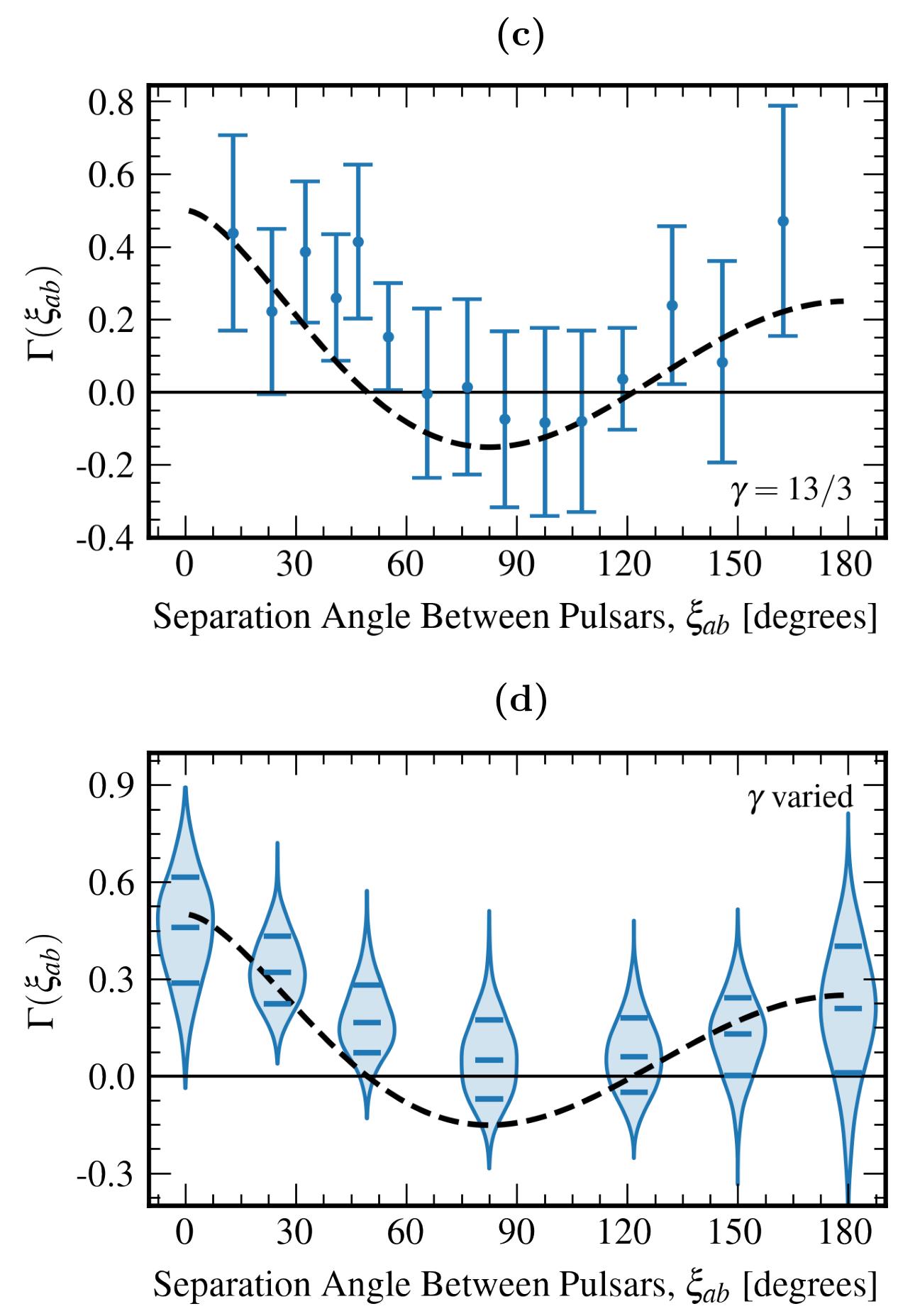
The reason the data points to the supermassive black hole binaries as the most likely explanation is simple: because of how galaxies are clustered, we expect that we’ll see different signals coming from different directions. So if there’s a relationship between correlations between any two pulsars and the angles, relative to our position, that those two pulsars are in the sky, that would be suggestive evidence for the supermassive black hole interpretation of the data. That evidence exists, but not at a high enough significance to claim “discovery” just yet.
That means we must consider the unpleasant: it’s still possible that this signal will turn out to be a fluke. It hasn’t yet reached the “gold standard” for discovery in physics and astrophysics: the 5-sigma significance threshold; it’s only about 4-sigma. There’s about a 1-in-10,000 chance that NANOGrav’s signal is a statistical anomaly, and that there is some other non-gravitational-wave-generating artifact that’s causing this to appear. But NANOGrav isn’t the only collaboration to have seen something suggestive.
- The Chinese Pulsar Timing Array, CPTA, has announced the detection of this gravitational wave background at a 4.6-sigma significance, although their major limitation is that they only have 3 years worth of data.
- The Indian Pulsar Timing Array, InPTA, has seen something consistent with a gravitational wave background “hum” to the Universe, but only at a 3-sigma significance.
- Australia’s Parkes Pulsar Timing Array can neither confirm or refute the existence of such a signal, as they only see weak (2-sigma) evidence for its presence.
But the International Pulsar Timing Array, over the next 1-2 years, hopes to synthesize together all the observations from all of these different collaborations. When they do, we just might hit that vaunted 5-sigma discovery threshold with the existing data that we have.
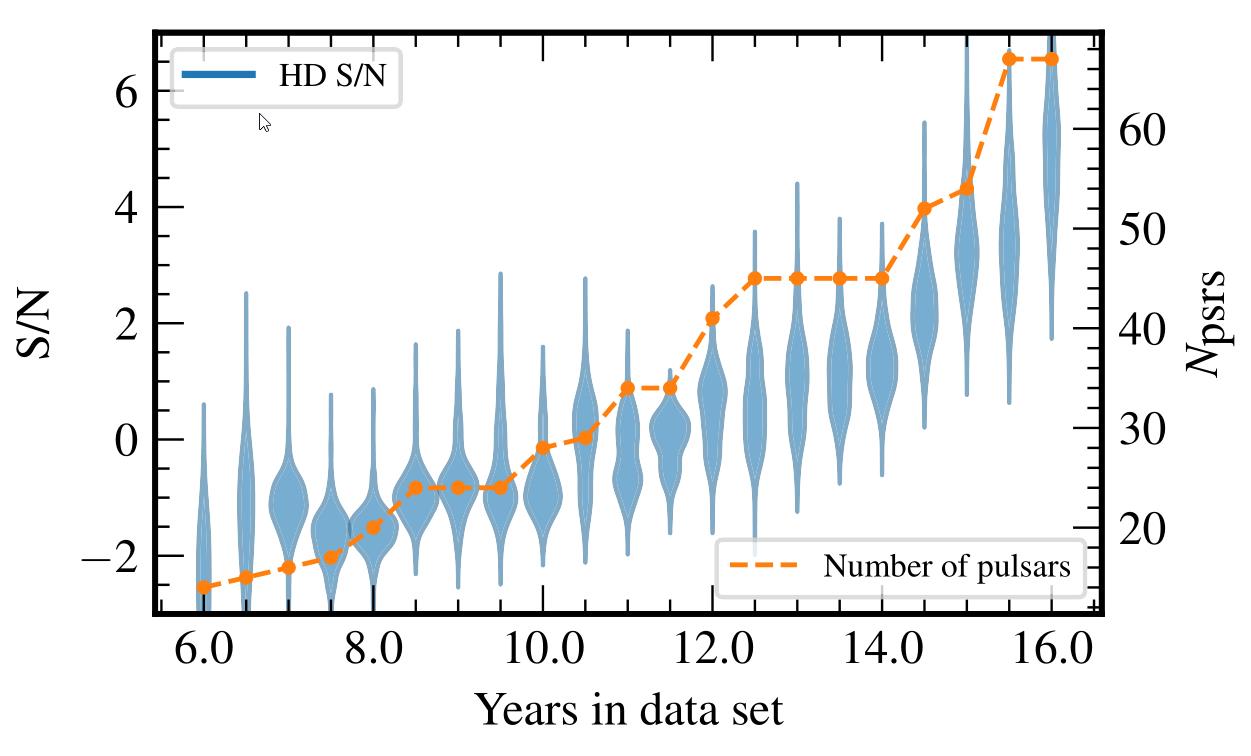
Don’t let any of that, however, stop you from appreciating just how significant this moment is for the history of science.
- We have detected the existence of the gravitational wave background of the Universe! Even though we have yet to characterize its nature, simply seeing that “it’s there” is a breathtaking achievement.
- We are on a path toward characterizing it, and when we can, we’ll have the second-ever method, after LIGO/Virgo’s terrestrial laser interferometer method, of directly detecting gravitational waves.
- And simply better-measuring pulsars, in terms of having greater numbers of pulsar-monitoring dishes and global coverage of those pulsars, will allow us to reach those goals.
But this achievement also makes a very strong science case for doing more: building larger and more sensitive radio telescopes themselves. With the collapse of Arecibo and the age of the Very Large Array, the science case has become overwhelming for building the ngVLA: the next-generation Very Large Array. It was named the top priority for radio astronomy by the National Academies in their 2020 decadal survey, and building it as designed would open up a new era of discovery for gravitational wave physics.
All of spacetime truly is rippling with the combined effects of all the gravitational waves in existence. For the first time, not only can we be confident that we’ve seen it, but we’re on the verge of actually understanding precisely where it comes from.