Runaway supermassive black hole caught by Hubble
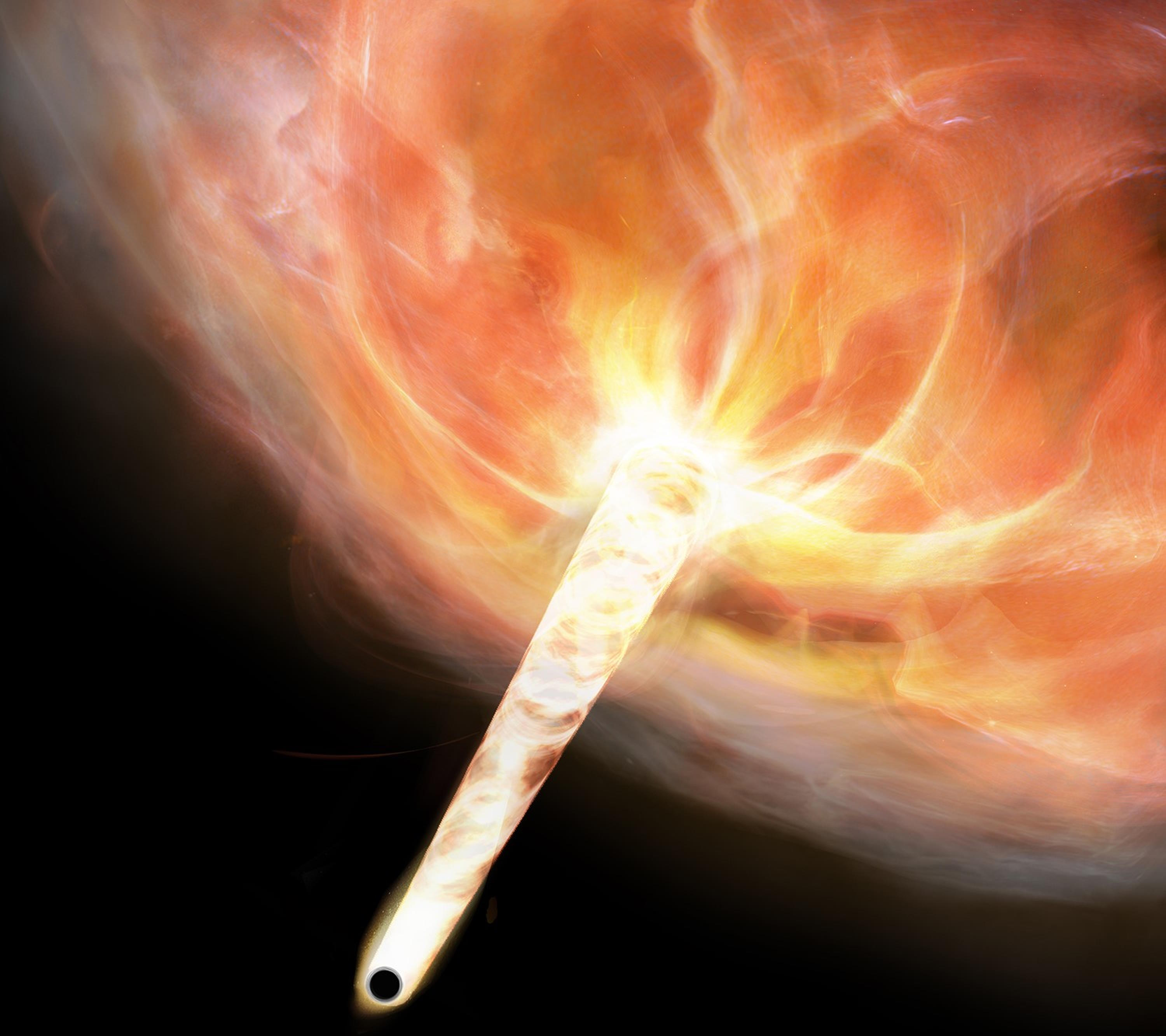
- While almost all major galaxies possess supermassive black holes at their cores, not every galaxy manages to keep and hold onto these cosmic behemoths forever.
- Whenever black holes merge, their spins and orbits can result in the post-merger black hole receiving a high-velocity kick: sometimes fast enough to eject it from their host galaxies entirely.
- Over the history of the Universe, thousands upon thousands of supermassive black holes should’ve been ejecting, roving the cosmos as rogue SMBHs. Remarkably, Hubble just spotted one!
If a supermassive black hole could somehow be separated from the host galaxy where it formed and grew up, what would happen to each of them? As it turns out, the supermassive black hole wasn’t needed to “anchor” or “stabilize” the galaxy, even in the inner regions. With copious amounts of stars, gas, dust, and even dark matter in the galactic core, the galaxy will be fine, and will even begin regrowing a new black hole in short order. Sure, the interior orbits of stars and X-rays normally produced by the central gas will be affected, but only for a short time. After a further few billion years go by, the only hint that will remain within the galaxy is an uncharacteristically under-massive supermassive black hole: one that’s less than 0.001% of the total mass of the galaxy.
But the black hole, even though it doesn’t emit any light of its own, will be capable of generating an impressive array of signals. As it passes through the interstellar medium of the galaxy that it originated from, it will leave a mix of fast shocks in the gas plus evidence of recent star-formation in its wake. As it travels through any other interloping galaxies, it will generate a very similar signal. Its speed should indicate relative motions of ~1000 km/s or above. And if it happens to align with a background object, a lensing or even a microlensing signal might be detectable.
For the first time, a candidate for a runaway supermassive black hole has been identified by a combination of shocks and star-formation seen in the wake of its path. Here’s why this might be the first of thousands of such runaway, or rogue, supermassive black holes.
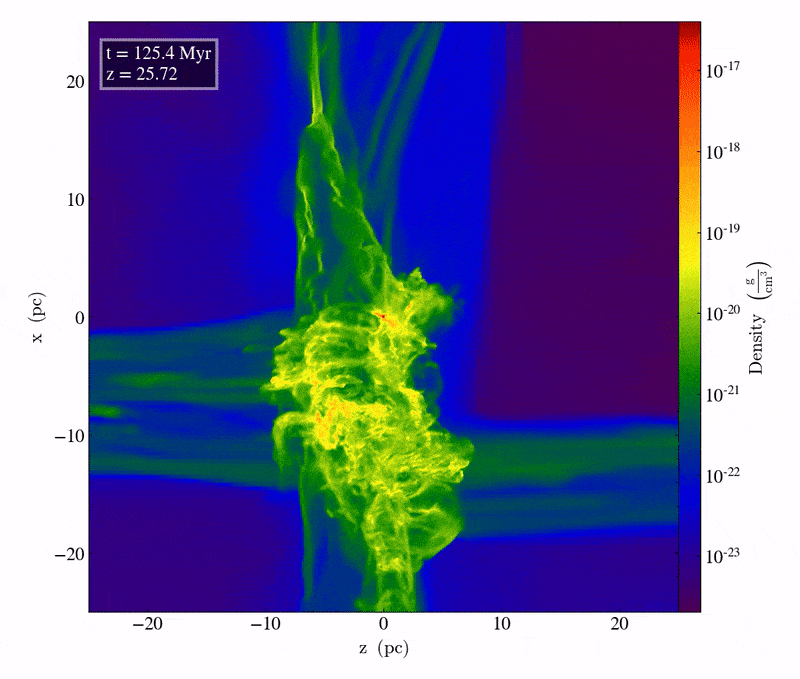
The theory of supermassive black holes starts off in the early Universe: after the formation of neutral atoms but before the formation of stars: in what we commonly call the Universe’s “dark ages.” There were small density imperfections in the Universe at that time, seeded by inflation at about the 1-part-in-30,000 level and, thanks to the complex interplay between gravitation, radiation, and both normal-and-dark matter, having grown to a maximum of about 1-part-in-5,000 by the time that neutral atoms form. The overdense regions continue to gravitationally grow, leading to the formation of large molecular gas clouds and streams of flowing, infalling gas onto and within them.
By the time the Universe is somewhere between 100 and 200 million years old, the largest of these gas clouds have grown tremendously, and now weigh in somewhere between 10 million up to nearly 1 billion solar masses. At this point, they fragment and begin to gravitationally collapse, cooling off primarily via the radiative properties of the relatively rare molecular hydrogen (H2). As simulations have shown, and hopefully as observatories like JWST and ALMA will someday verify, this creates individual collapsed “overdensities” of several tens of thousands of solar masses: the most massive of the first stars and/or the seeds of the earliest supermassive black holes.
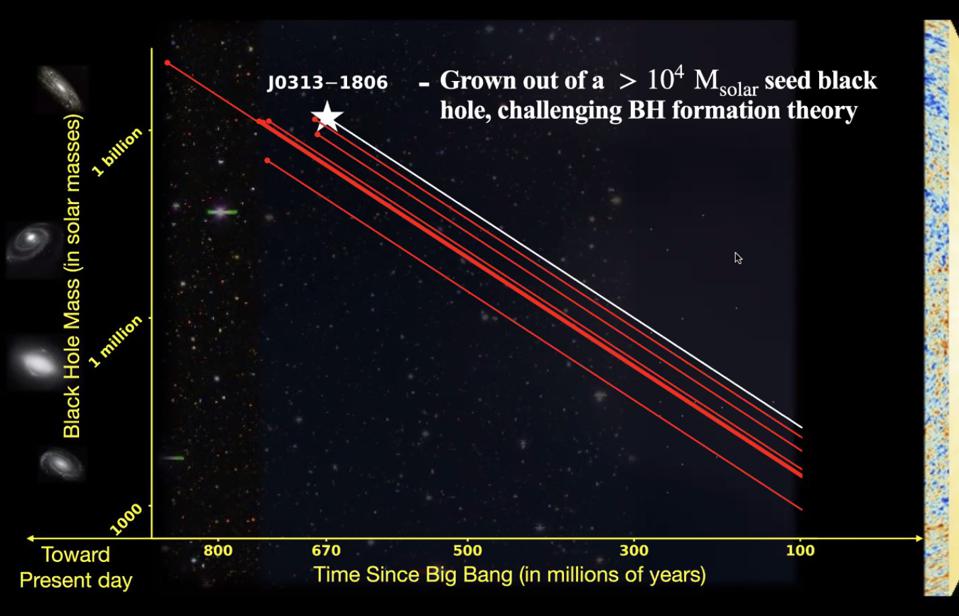
These supermassive black hole seeds then grow through three major processes:
- the coalescence and merger of stars and smallish black holes with the supermassive seed,
- the rapid infall and accretion of normal matter, with conditions occasionally allowing the mass accretion rate to exceed the theoretical Eddington limit,
- and the major mergers of proto-galaxies and full-grown galaxies, each with their own supermassive black hole inside.
The first two processes aren’t typically going to be capable of dislodging the supermassive black hole from its position at the center of the galaxy. The requirement that energy and momentum be conserved in these interactions allows the speed of the supermassive black hole, relative to the center of the galaxy, to change by only ~1 km/s or less, even as the black hole continues to grow in mass.
However, whenever two comparably sized galaxies merge together, you can also expect that each will have a supermassive black hole at their center, and that their masses will be of relatively the same size: within a factor of ~10 of one another. Each black hole will not only be spinning rapidly, at speeds close to the speed of light, but these black holes will be orbiting one another with relatively random orientations relative to each of their spin-axes.
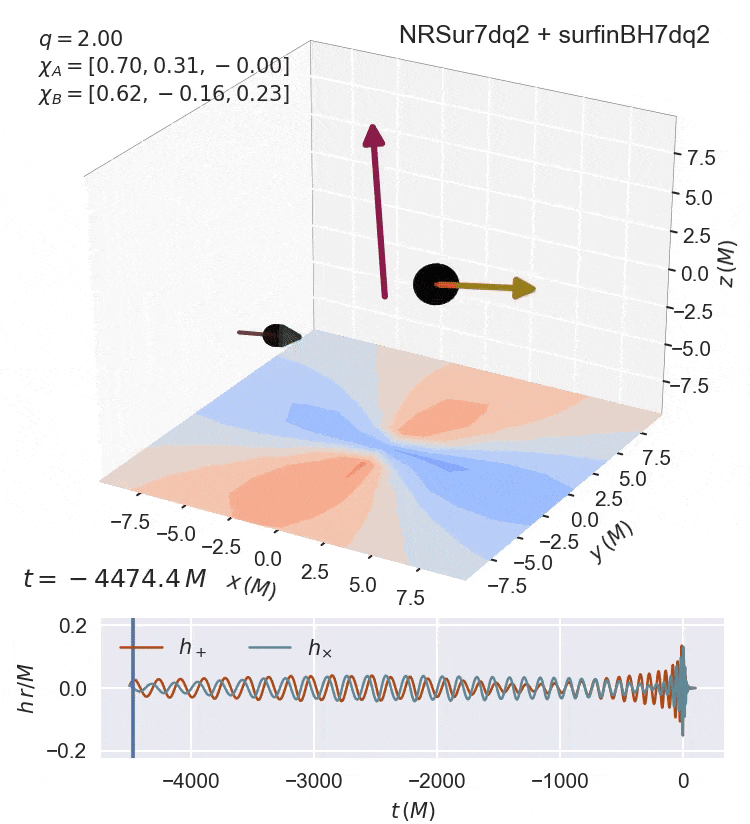
What happens next is both “the hard part” from a theoretical perspective, but also the all-important part in order to enable us to predict what ought to happen next. Combined, these two black holes will have:
- a mass ratio with one another, where the less massive one is somewhere from ~10-100% the mass of the larger one,
- spins that are both large in magnitude, but misaligned with one another by anywhere from 0° to 180°,
- and a significant amount of orbital angular momentum that, in general, will also be misaligned with the spins of both black holes.
It’s the physics of General Relativity that determines what happens next. In all cases, about ~10% of the mass of the secondary (less massive) black hole will be radiated away by the system in the form of gravitational waves during the inspiral-and-merger phase. The remnant black hole will have a mass that’s the sum of ~100% of the primary (more massive) black hole and ~90% of the secondary black hole, with the remaining energy (via Einstein’s E = mc²) emitted in the form of gravitational waves.
However, depending on the specifics of these orbital conditions, more gravitational waves will be emitted in one direction than the opposite direction, causing the remnant black hole to be “kicked” in the momentum-conserving opposite direction.
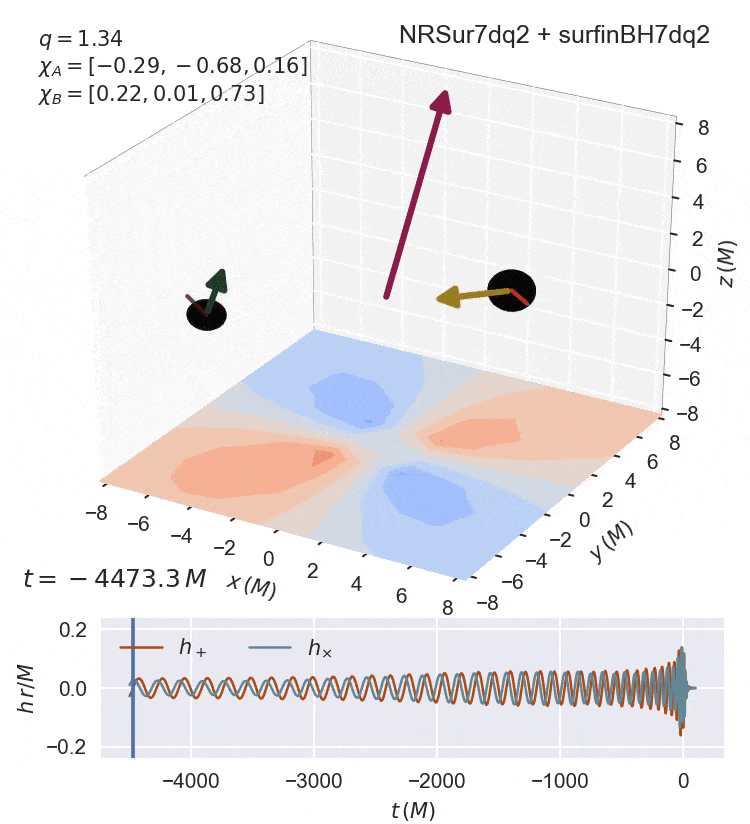
Typically, the remnant black hole receives a “kick” from this merger. Because the masses can be relatively close to one another, kicks of tens of kilometers-per-second, or roughly the speed of Earth around the Sun, are fairly normal. But you have to recognize that the “normal” case is only going to happen most of the time. In the most extreme cases, which occur somewhere in ~0.1% to about ~1% of configurations, the spins of the two black holes, just prior to the moment of the merger, will line up in a single plane.
In this case, the gravitational waves are maximally emitted in one direction, and the recoil of the post-merger black hole will be as large as is physically allowed, and will occur in the opposite direction. Instead of “tens of kilometers-per-second,” we’re now looking at post-merger speeds for the black hole of up to several thousands of kilometers-per-second, or somewhere around 1-2% the speed of light itself.
It only takes a speed of a few hundred kilometers-per-second to escape from the gravity of the modern Milky Way at the location of the Sun, so any merging black holes that achieve these so-called “super-kick” conditions will be excellent candidates for being kicked out of their home galaxies entirely.
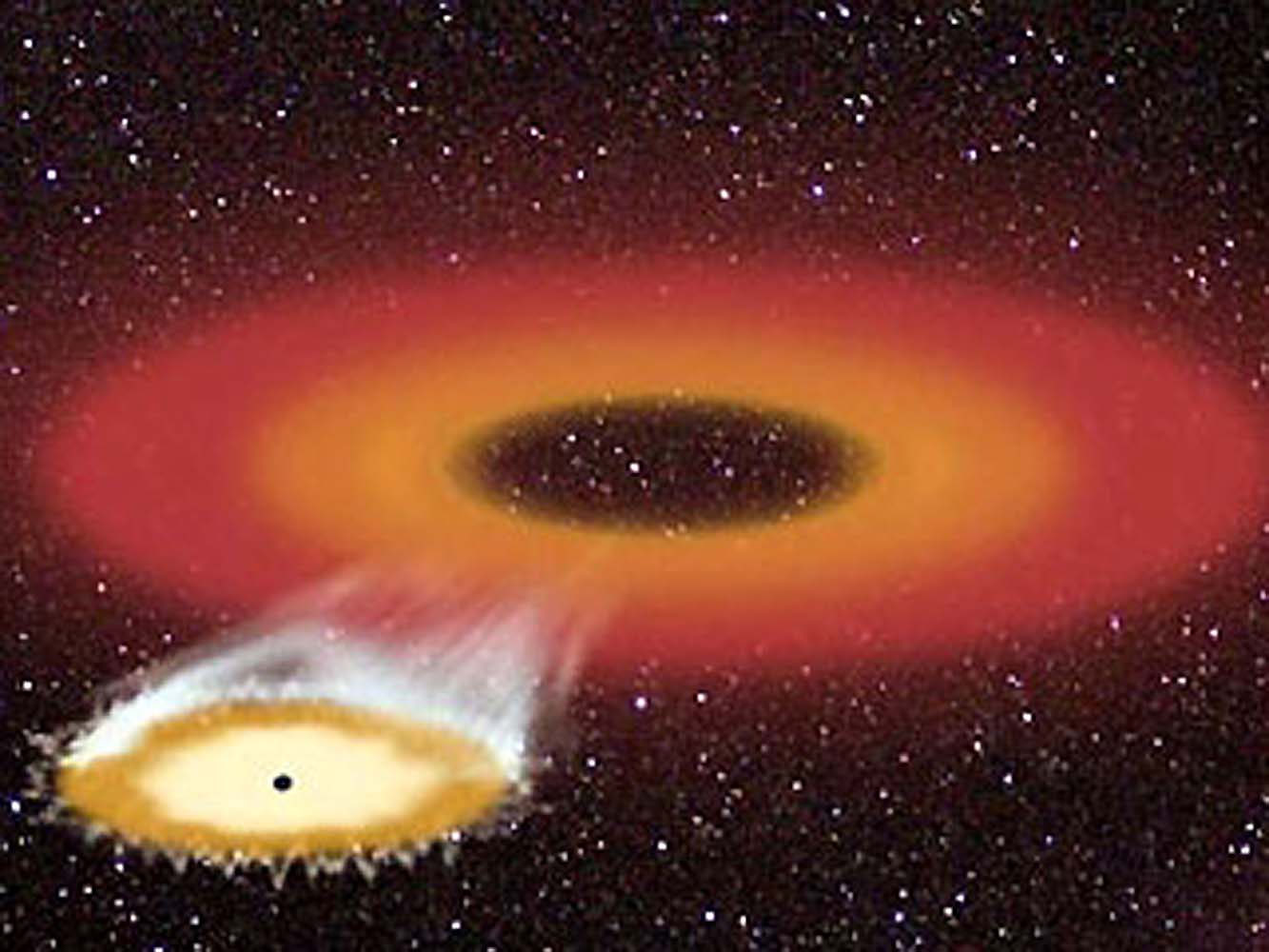
Considering that:
- there are somewhere between ~100 million to ~1 billion very large, Milky Way-sized (or larger) galaxies in the modern Universe,
- that a typical large galaxy undergoes somewhere around ~5-10 of these substantial mergers over their histories,
- and that from ~0.1% to ~1% of all major mergers have the potential to eject their supermassive black holes,
then even if we take the more conservative numbers — 100 million galaxies, 5 major mergers per galaxy, and 0.1% of such mergers are capable of ejecting a supermassive black hole — that implies there are at least 500,000 events within our observable Universe that, at one point, successfully ejected a supermassive black hole from their host galaxy.
These black holes will initially be “runaway” black holes, meaning they’ll be moving anywhere from several hundred up to a maximum of around ~5000 km/s relative to their galaxy, which is fast enough to escape even the strongest galaxy’s gravitational pull. After escaping from their home galaxy as well as whatever group/cluster of galaxies the original galaxy belonged to, they’ll then wander the Universe as isolated masses in intergalactic space: orphan, or rogue, supermassive black holes.
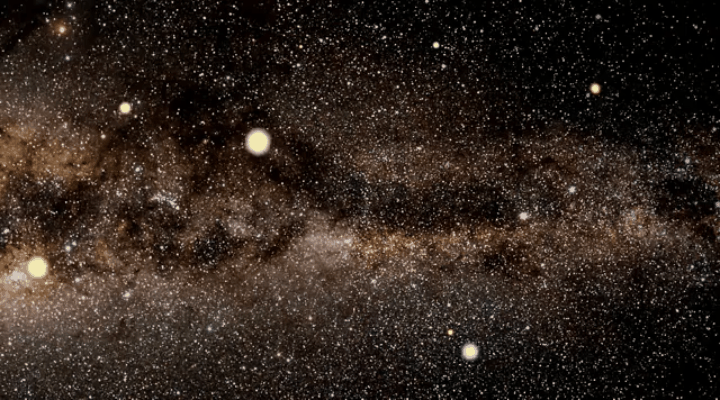
Theoretically, that’s the expectation. Based on how large a big galaxy typically is — something like 100,000 light-years for its galactic disk and several hundred-thousand light-years more for its gaseous halo — it should take between tens and hundreds of millions of years for a runaway supermassive black hole to completely exit its host galaxy. This means that, if we can catch a runaway black hole within this time interval, something we can expect for at least ~0.3% of runaway black holes within the observable Universe at any particular moment, we might find the first example of a phenomenon that should appear at least thousands of times for those of us looking “now,” 13.8 billion years after the hot Big Bang.
The place you’d want to look is near a rapidly star-forming galaxy: something you’d expect to see in the aftermath of a significant merger event. The galaxy might appear irregular, should be rife with hot, young stars, and should have large quantities of significantly ionized gas. But the twofold signature you’d need to find within those environments are:
- a narrow, linear feature that consists of hot, shocked, ionized gas in the circumgalactic medium of the host galaxy that points “away from” the galactic center,
- plus evidence of star-formation occurring along that narrow line, likely in bursts,
with the possibility of a very hot, energetic “knot” that represents the leading edge: where the supermassive black hole is right now.
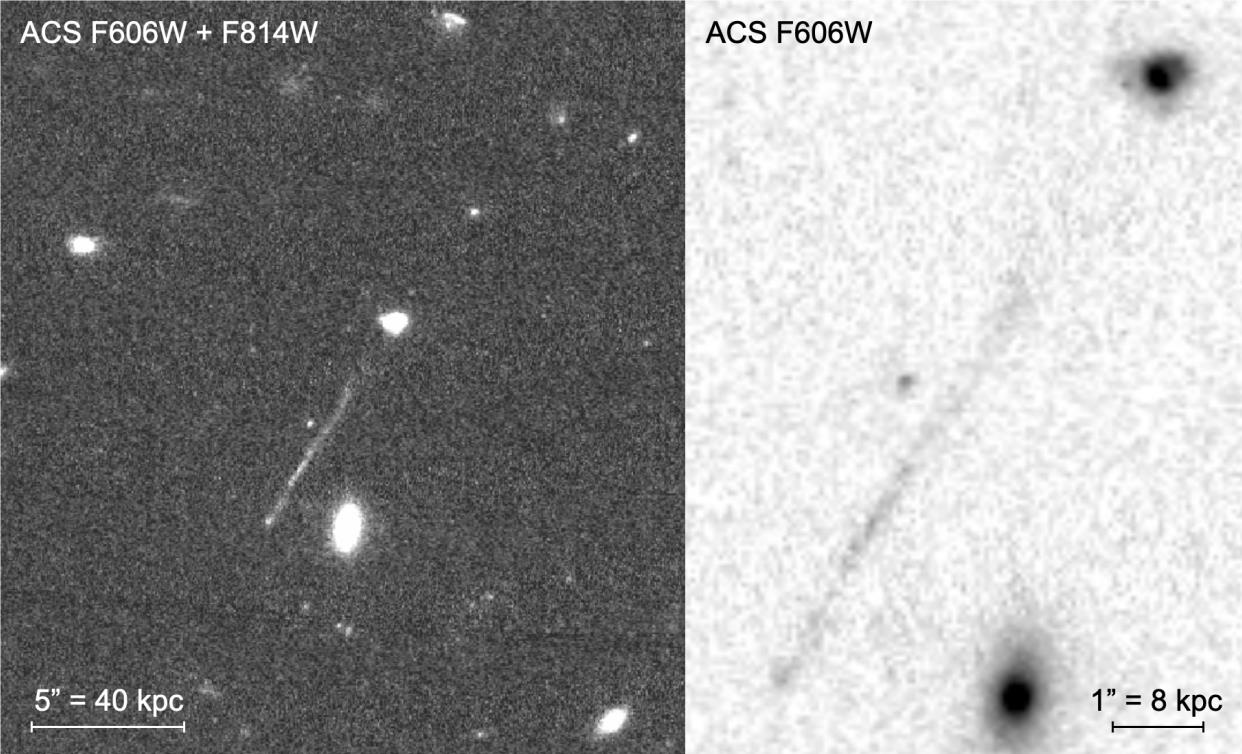
What you see, above, is a fantastic and serendipitous image of what just might be the first such runaway supermassive black hole ever spotted: the first of what must be at least thousands of them out there to observe. While observing a compact, star-forming galaxy a long way from home — about 10.6 billion light-years away, at present — Hubble also captured the surrounding field using its ACS (Advanced Camera for Surveys) instrument. A feature that appears very similar to what one might expect, linear in nature and “pointing” away from the galaxy itself, can be seen streaming away from the galaxy in question.
Follow-up observations were then taken using the spectroscopic capabilities of the upgraded LRIS (Low Resolution Imaging Spectrometer) instrument aboard the 10-meter Keck Telescope, and they found the dual signature of doubly-ionized oxygen alongside the more standard optical emission lines of hydrogen, indicating gas of varying temperatures and densities, peppered with new stars, where the temperature exceeds ~50,000 K in realms where the doubly-ionized oxygen signature is strongest.
And perhaps most remarkably of all, according to the study authors, “The feature terminates in a bright [doubly-ionized oxygen] knot with a luminosity of 1.9×1041 ergs/s.” This is exactly the type of feature you’d expect if it were caused by a runaway supermassive black hole.
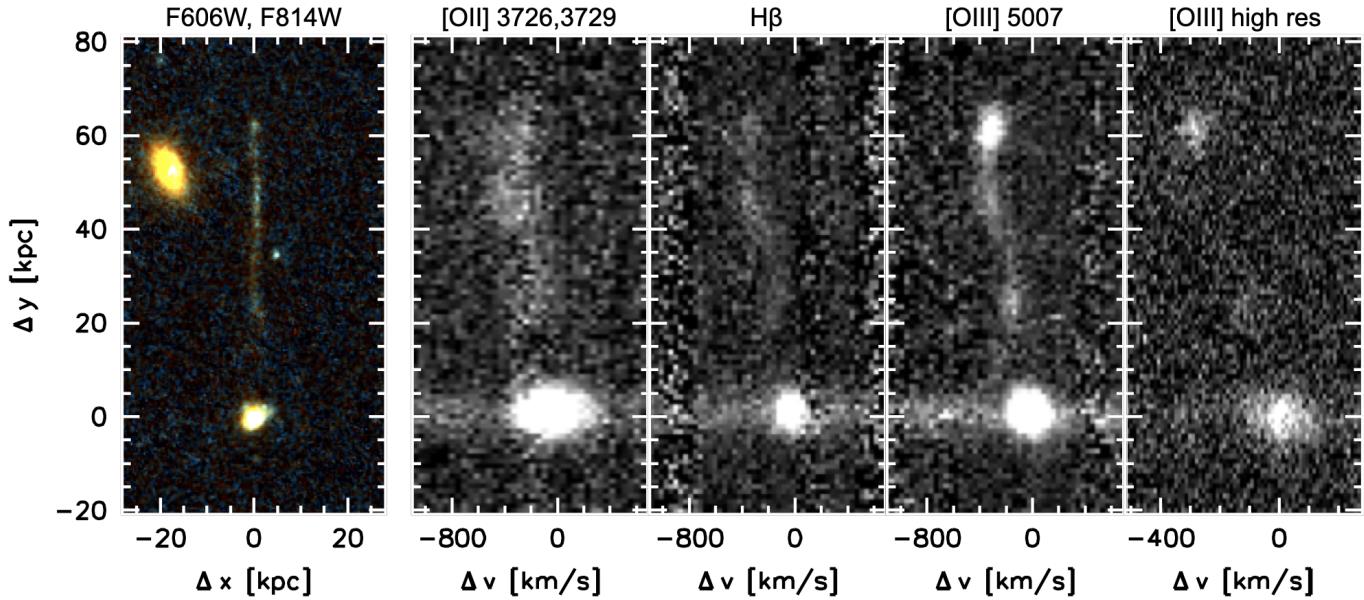
You might be skeptical that this is truly a runaway supermassive black hole, and you’d have good reason to be. There are a couple of features that don’t quite line up with what one might expect to see. For one, the feature doesn’t make a perfectly straight line, but rather a wiggly, irregular one that spreads out toward the “tail” end. And for another, although the evidence is weak, there’s some indication of a small “counter-line,” like something else has recoiled in the opposite direction from the candidate runaway supermassive black hole.
But when you look at the degree of “how non-linear is the observed feature,” you find that it’s 100% consistent with the normal motions of gas clouds within a galactic halo, i.e., the circumgalactic medium. When you look at the degree that the tail has spread out relative to the rest of the runaway black hole’s wake, you find that it’s perfectly consistent with a population of stars and gas that’s expanded after being heated and subject to a star-formation episode. With an estimated age of ~39 million years and an estimated ejection speed of ~1600 km/s, the wiggly, irregular feature is precisely in line with what we’d expect.
As for the “counter-line” feature, the authors present the (rather weak-sauce, IMO) evidence for it, and then launch into an unnecessarily complex explanation for it: the suggestion of three merging galaxies and a set of “recoiling” black holes.

Although that’s a plausible scenario, it should be far less common than the much more straightforward scenario of two similarly massed black holes merging and with the post-merger black hole receiving a super-kick from the emitted gravitational waves. While follow-up observations, particularly if one leverages the power of either JWST or ALMA, should be able to further probe these features, it’s eminently plausible that any “counter-line” is wholly unrelated to the conjectured black hole activity, and that no massive recoil is necessary to explain what’s already been observed. A simple merger of two supermassive black holes amidst a galactic smash-up may be enough.
While this is no doubt going to be followed-up aggressively by the astronomy community, what’s important to take away is the following.
- Runaway supermassive black holes should exist in significant numbers.
- The newest ones should be found around galaxies that have recently undergone major mergers.
- They will produce linear features from interacting with gas in the circumgalactic halo, including shocks, new stars, and “knots” at their head.
- And they should exhibit speeds of ~1000 km/s or even greater, relative to the galaxy they were ejected from.
Given that we live in a galaxy of ~1 trillion solar masses but possess a supermassive black hole of only ~4 million solar masses, it may be further supporting evidence for the idea that we, too, once had a large supermassive black hole and lost it at some point in our cosmic past. Perhaps if microlensing studies improve to the point where we can find and trace rogue supermassive black holes as they travel through the Universe, we’ll someday be able to better reconstruct our own cosmic history.