Ask Ethan: Could a “copy” of me exist in the Multiverse?
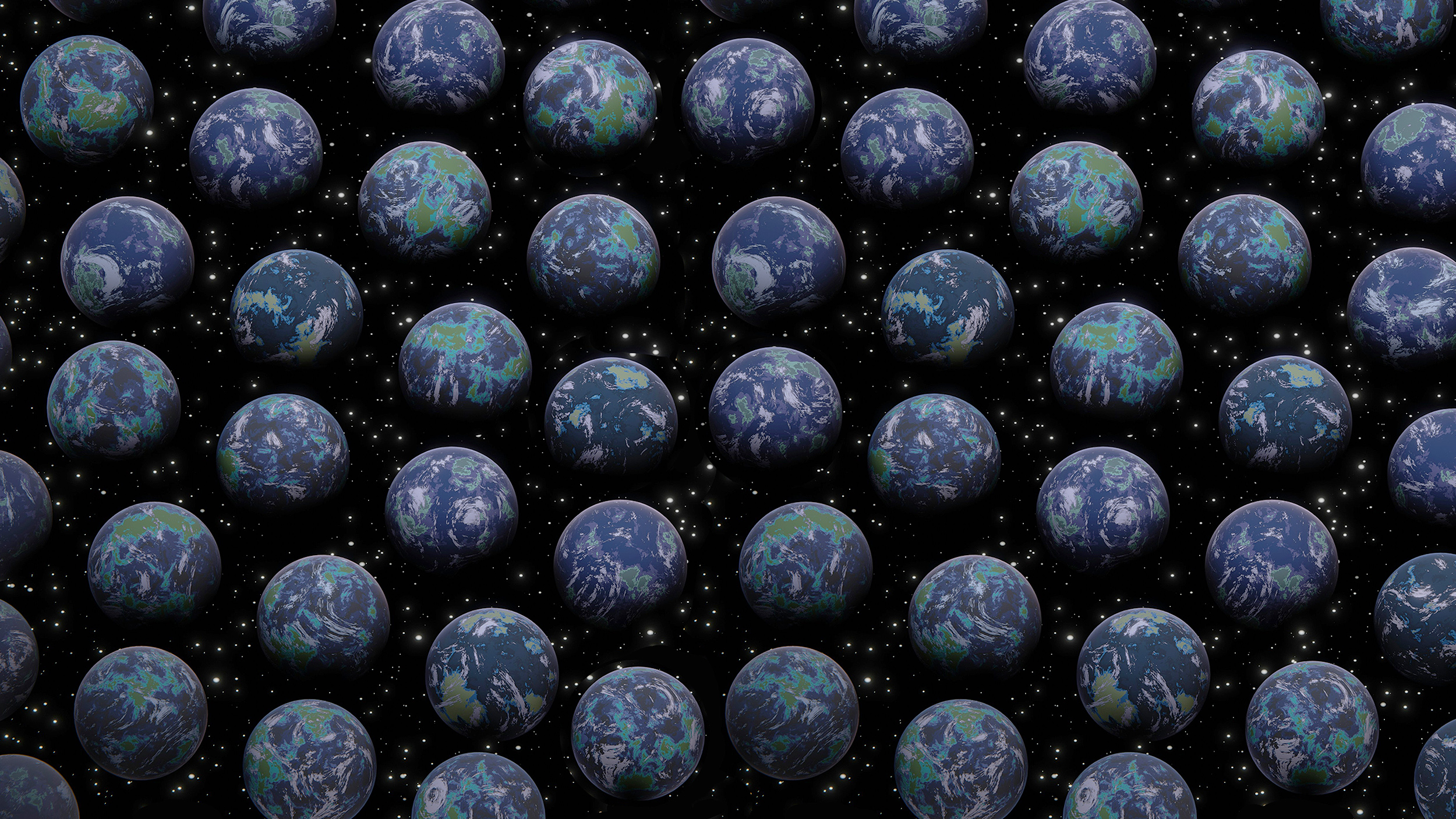
- The multiverse is a fascinating idea in theoretical physics, providing the notion that somewhere out there are identical physical systems to our own, but where different quantum outcomes could have occurred.
- This gives us hope that somewhere out there are versions of ourselves that are having better lives, as a result of having made different, superior decisions to us at critical junctures in their existence.
- Even more wild are possibilities that different pockets of the multiverse have different fundamental constants, with vastly different properties to everything in existence. What would it take for this to be true?
Here in our Universe as we know it, once an outcome has occurred, there’s no going back. Once you open a bag of potato chips, you can never return that bag to its unopened state; the air molecules from inside and outside have already mixed with one another, even if you reseal it. Once you cut down a tree, you can never return the tree to the state it was in prior to you cutting it down. Even on a quantum level, once you measure a particle’s spin, you can never return it to its pre-measurement state. We have only the one Universe that we inhabit, and while future events do not yet have determinate outcomes, past events all do.
But what if there were copies of our Universe out there, far beyond the limits of what’s observable or measurable, that were identical to our own? Would it be possible for different outcomes to have occurred in those Universes, and what would that mean for a variety of systems: quantum particles, trees and potato chip bags, or even entire human beings? That’s what Danny Porter wants to know, writing in to ask:
“If the universe is infinite, and string theory suggests that strings can only vibrate in a finite number of ways to form particles, is it possible that a version of me could exist somewhere else in the universe? If another version of myself were to exist in a region of the universe with different physical constants, such as a significantly altered gravitational constant, how might such variations affect my physical appearance or biological structure?”
These are two very big ideas and concepts, and certainly worth investigating. In order to answer them, we’re first going to have to unpack what:
- an infinite universe,
- string theory,
- different versions of ourselves,
- and different fundamental constants,
all have to do with one another.
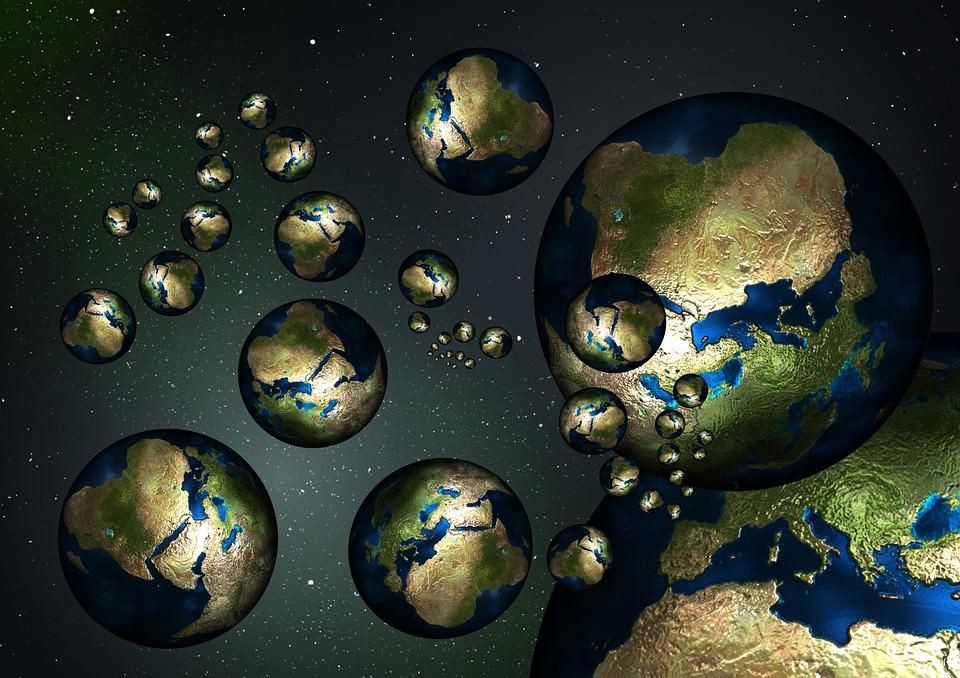
The way to do that is to first understand what the whole notion of the multiverse is, and to recognize that when scientists talk about it, there are actually two different meanings for it.
First, there’s the quantum multiverse. It comes from the notion that every time two particles interact with one another, there isn’t a definitive outcome that absolutely must occur, but rather an array of possibilities that could occur. Sometimes these possibilities are discrete: an electron can be “spin up” or “spin down” when you measure it, and as long as its spin remains unmeasured, it can exist as a combination of both states at once.
Other times, the possibilities are continuous: an electron and positron, when they interact, will annihilate into two photons, each with 511 keV of energy. But those two photons, although they’ll always travel in opposite directions from one another (in order to conserve linear momentum), have no restriction on the orientation of each individual photon. They could travel in the x-direction, the y-direction, the z-direction, or any combination of the three.
Each time a quantum interaction occurs, there isn’t a definitive outcome that we can predict. We can only predict the spectrum of possible outcomes, weighted by probability. It’s only when we make that critical measurement that we can determine which outcome actually occurred within our observable Universe.
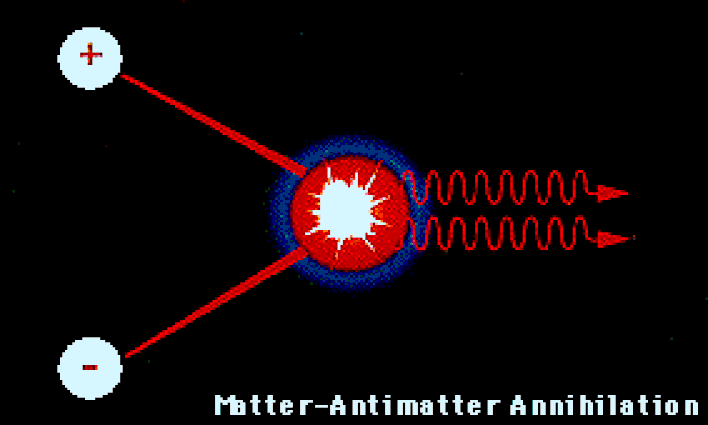
In quantum mechanics, we typically describe this behavior probabilistically: through a wave equation. Instead of particles having definite properties (like “position”) that can be known at every step along the way — what we’re used to from a classical, deterministic view of reality — we instead can recognize that particles exist in what we call a “superposition of states,” where each possibility for its value is weighted by the probability of it having that value at that moment. These entities propagate like waves, where they do not take on a definitive value while they’re non-interacting. However, when we do make a measurement (or force an interaction), we then instantly determine that value, and only one such possibility is realized by that measurement (or interaction).
The big idea of the quantum multiverse is that instead of the above picture I just described, which aligns with the standard Copenhagen interpretation of quantum mechanics, you can instead consider an interpretation where what we observe as occurring in our Universe as a “measurement” with a specific “outcome” instead is just one possible realization of all possible outcomes. Those outcomes might well all occur, just in other universes from our own. Mathematically, those universes fit into a mathematical structure known as a Hilbert space. But if we want to consider a quantum multiverse, we might imagine that, somewhere far beyond our own observable Universe’s boundaries, what we might call those other “parallel universes” do indeed exist, where all of the other possible outcomes have occurred as well.
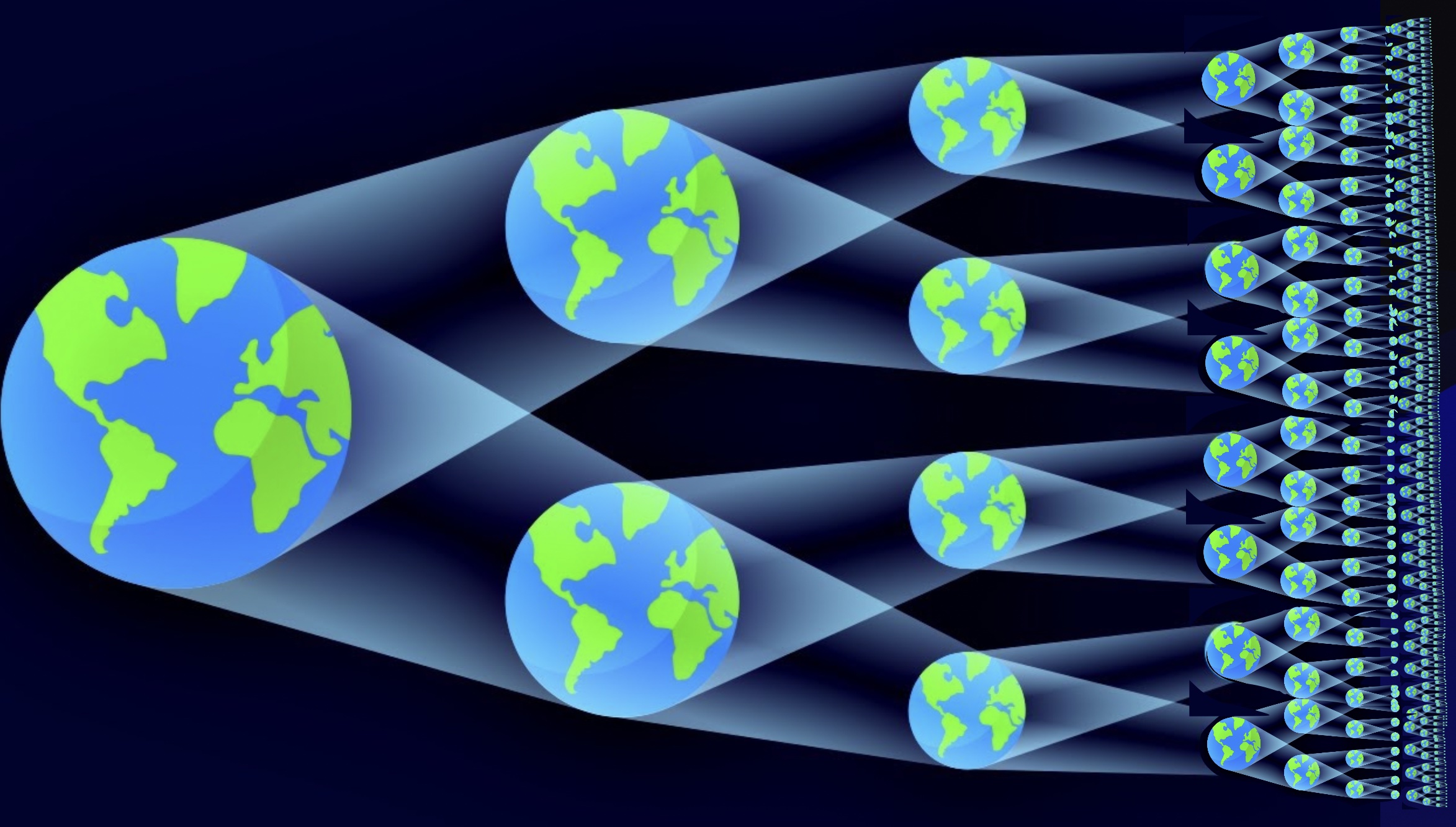
That “quantum multiverse” is one notion of what a multiverse can be. But there is another, and that comes to us from the world of inflationary cosmology.
According to the theory of cosmic inflation, prior to the Big Bang, space wasn’t filled with particles of matter and radiation, but was rather empty, save for energy that was inherent to space itself: field energy that was inextricable from the very fabric of space. Under those conditions, space expanded at what we call an exponential rate: where after a certain brief amount of time, all three dimensions double in extent, and then when that same amount of time elapses again, the scale of space has doubled yet again, and so on and so on. This can take even a minuscule region of space, initially, and “inflate” it to gargantuan proportions. When inflation ends in a specific region of space, that energy that was inherent to space gets converted into matter and radiation, and that signals the onset of a hot Big Bang.
The theory of cosmic inflation thus provides us with an “inflationary multiverse,” or a second type of multiverse. Although the quantum multiverse had been around in some form or another since 1957 and was greatly popularized in the 1970s, it was only with the notion of cosmic inflation that these so-called parallel universes finally had a potential place to live. While it’s untrue that the inflationary multiverse is large enough to hold all of the possible outcomes of the quantum multiverse (it is, in fact, not) in general, there still remains the possibility that either:
- inflation went on for an infinite amount of time to the past,
- or that the universe, including the part that underwent inflation, was born infinite,
which would indeed give all possible quantum outcomes a potential place to reside.

The quantum multiverse is the notion that there are “different versions of ourselves” or our reality, and the inflationary multiverse represents how large the total volume of all types of space, combined, are, and whether it’s large enough to contain all of those different possibilities. If we assume that the inflationary multiverse is indeed infinite in size, then the quantum multiverse being real is indeed a possibility, and we can consider that perhaps there really are not only copies of us within the multiverse, but an infinite number of such copies.
What does any of this, though, have to do with string theory?
Within our observable Universe, we see that the fundamental constants are the same everywhere we look, as well as at all moments throughout our cosmic history. The fundamental parameters of our Universe do not change. Although we do not understand what determines those parameters — they may as well be God-given for all we know — they are not determined by any sort of known physics or dynamics that occurs. These constants that underpin our reality simply have the values that they have, and there’s no other possibility for what they could be, nor is there any reason to expect they would be different outside of the observable Universe, whether in other pockets of the multiverse or in any other scenario you can imagine.
But if you bring in ideas from string theory — marrying string theory with cosmic inflation — those constants could indeed be different in other pockets of the multiverse.
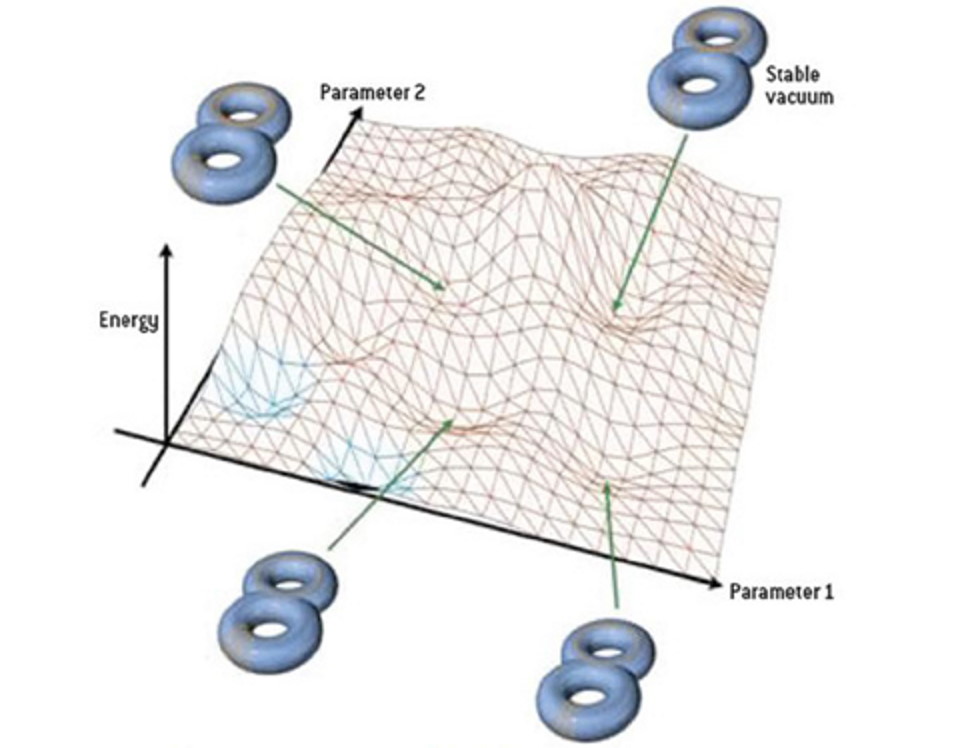
Some of you may have heard terms like the string landscape before, or the notion that there are something like 10500 possible universes out there according to string theory. This is because, in string theory, the fundamental constants aren’t just parameters that must be measured in order to determine them. Instead, those constants are determined by what we call the expectation value of the various string vacua: the values that a universe “settles on” when it transitions from a high-energy state to a lower-energy state. Just as a ball rolled across a hilly landscape will eventually settle into one of the valleys, string theory posits that, from some high-energy state where the ball could settle into many different valleys (like a roulette wheel), as the energy of the universe drops, the ball will land in one particular valley.
If you put inflation and string theory together, you can imagine a scenario where inflation gives you a wide variety of high-energy states, and that wherever inflation ends and you get a hot Big Bang out, each of those “baby universes” has its own unique values for the various string vacua. That would lead to different fundamental constants in each pocket of the multiverse, where those various baby universes would then unfold according to their own laws and rules, rather than the laws and rules that we’re used to here in our own observable Universe. If there are 10500 possible string vacua (although many have calculated there to be far greater numbers), then only 1-in-10500 of the baby universes generated will be similar to our own.
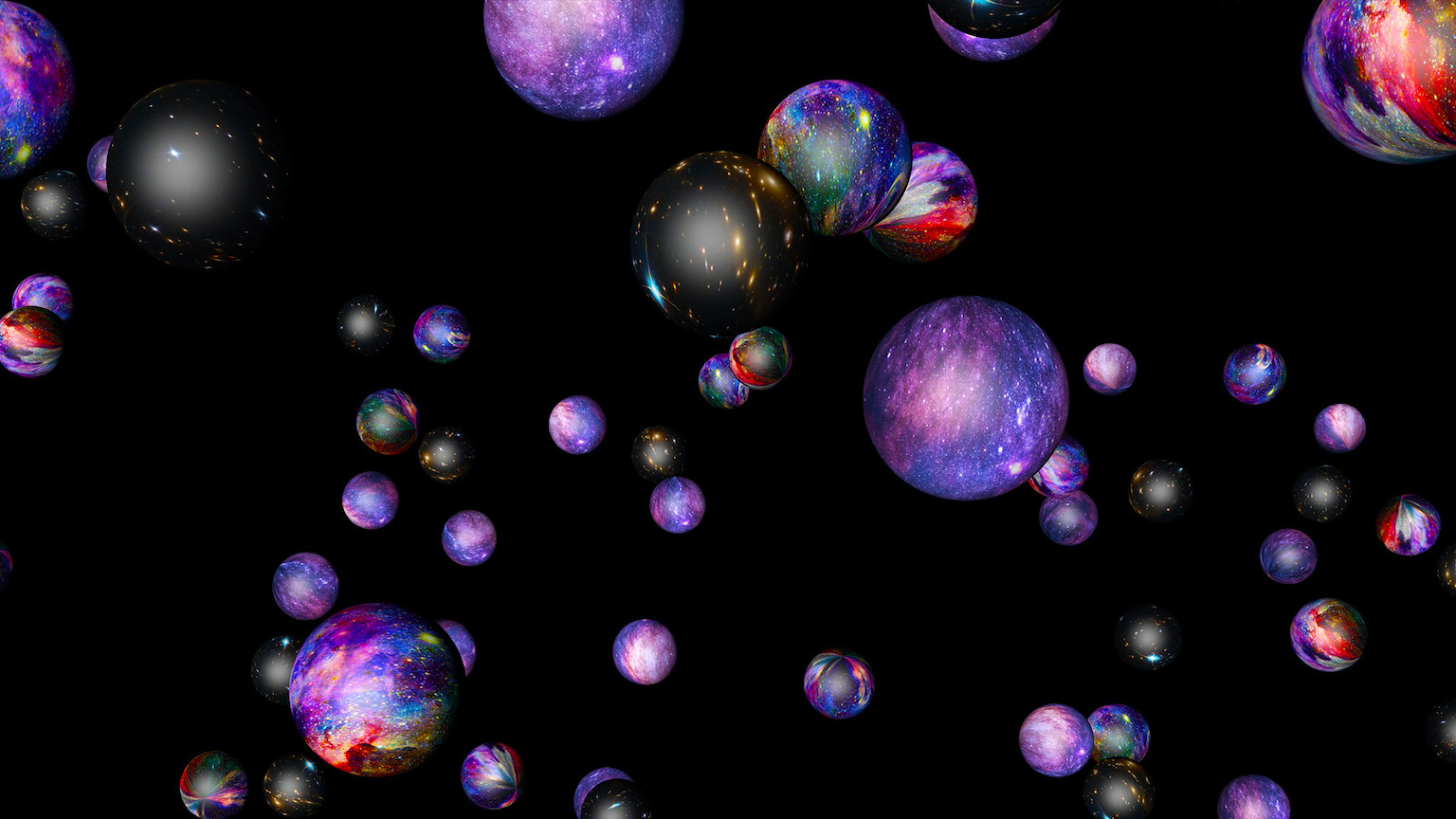
The inflationary multiverse is strongly suspected to be physically real, however, based on the known pillars of physics today. Simply by combining what’s known to be true about modern quantum field theory and inflationary theory, both of which are well-supported by a large suite of scientific evidence, this idea of disconnected regions of space which all have their own hot Big Bang, but are separated by ongoing regions where inflation continues, is generally accepted as an inevitable consequence of the physics we know today.
However, what about the speculations we build atop it?
String theory, despite how many adherents it has and how promising it is as a theory of quantum gravity, remains firmly in the speculative category as far as theories go. There is no known prediction of string theory that we can extract that differs from the predictions of our best modern physics theories without string theory, and no way that we can go out and test those two competing predictions against one another. Moreover, even if string theory is correct, and even if the idea of the string landscape is correct, there is no guarantee that the process of cosmic inflation is energetic enough to “reset” the values of the fundamental constants. In a stringy multiverse, it may well be the case that all pockets of the multiverse, and every “baby universe” that’s out there, have the same values for the fundamental constants that our Universe possesses.
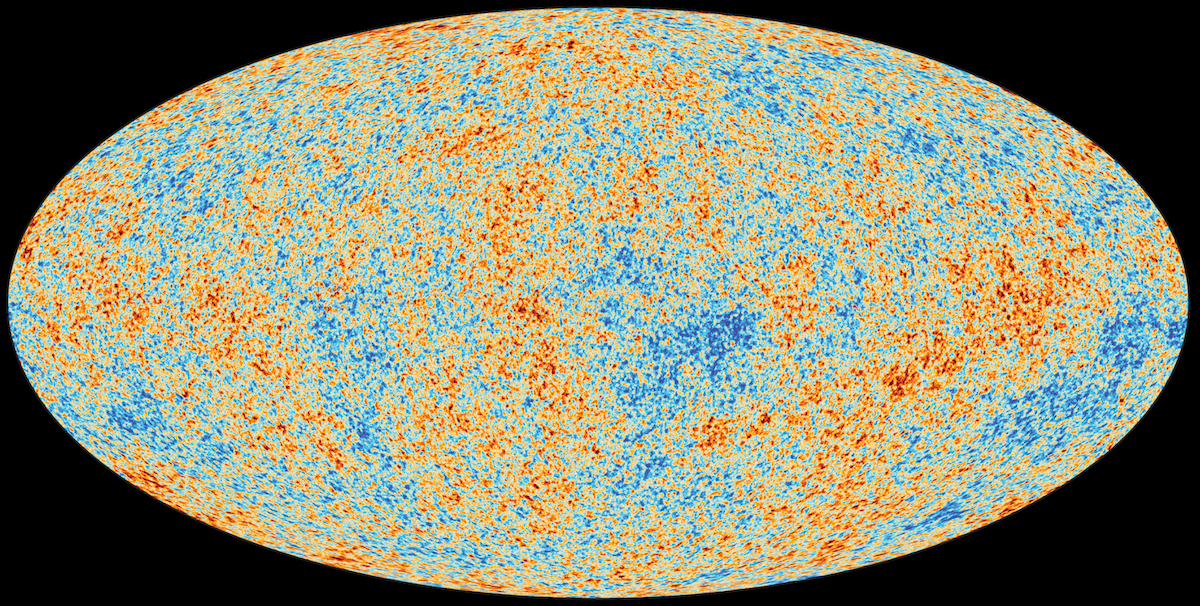
The reason why is not generally appreciated. In the theory of inflation, there’s an energy scale at which inflation occurs, and then when inflation ends, that energy gets converted into matter-and-radiation in the form of particles and antiparticles: into the known particles of the Standard Model. When we examine the fluctuations in the cosmic microwave background, they provide constraints as far as “how hot” the Universe got during the earliest stages of the hot Big Bang. With data from WMAP, the Planck satellite, and several other projects, we now know that energy scale is somewhere below around 1016 GeV, or less than one-thousandth of the energy needed to reach the Planck scale.
The Planck scale is the important comparison point here, because that is not only the energy scale at which our currently known laws of physics break down (and where a theory of quantum gravity would be needed to make sense of physics), but that’s supposedly where the string scale comes into play. If inflation occurs at an energy scale that’s well below the Planck scale, then there’s no reason to believe that inflation has the ability to:
- determine or change the fundamental constants,
- plunk one baby universe into a different value for any of the string vacua than any other baby universe,
- or explore different aspects of the string landscape.
Based on what we can observe, we have no compelling reason to be certain that inflation has the ability to touch the values of the fundamental constants at all. It is simply an unsupported assumption about reality, much like most of string theory.

However, it’s important to recognize what string theory is: a tremendously rich theory with a much more complex set of relationships between particles, fields, interactions, and anything else you can imagine than the Universe we know, love, and inhabit. String theory is (at least) a 10-dimensional theory, rather than the 4-dimensional Universe we inhabit. String theory has hundreds of additional degrees of freedom, which correspond to potential interactions, particles, and fields that go way beyond what exists in our Universe; most of what is possible is not reflected in our reality. It’s why I have likened string theory to an unlikely broken box, where something “sticks a key in it and turns it,” and only a tiny fragment of the original box remains to describe our actual Universe.
In most instances, it’s a challenge to “get rid” of all the pathological possibilities that would make the formation of even a single atom within the universe impossible and forbidden. In most cases, the universe that would arise in string theory would either dissipate so quickly that no two quanta would ever interact, or alternately would recollapse so quickly that nothing as complex as an atom could ever form at all. For every googol possible universes (where a googol is 10100) that string theory gives us, fewer than one of them even admits the possibility of forming an atom at all. It’s actually one of the worst scenarios imaginable for producing our Universe; you have to imagine a stupendous number of failed universes in order to get our own.
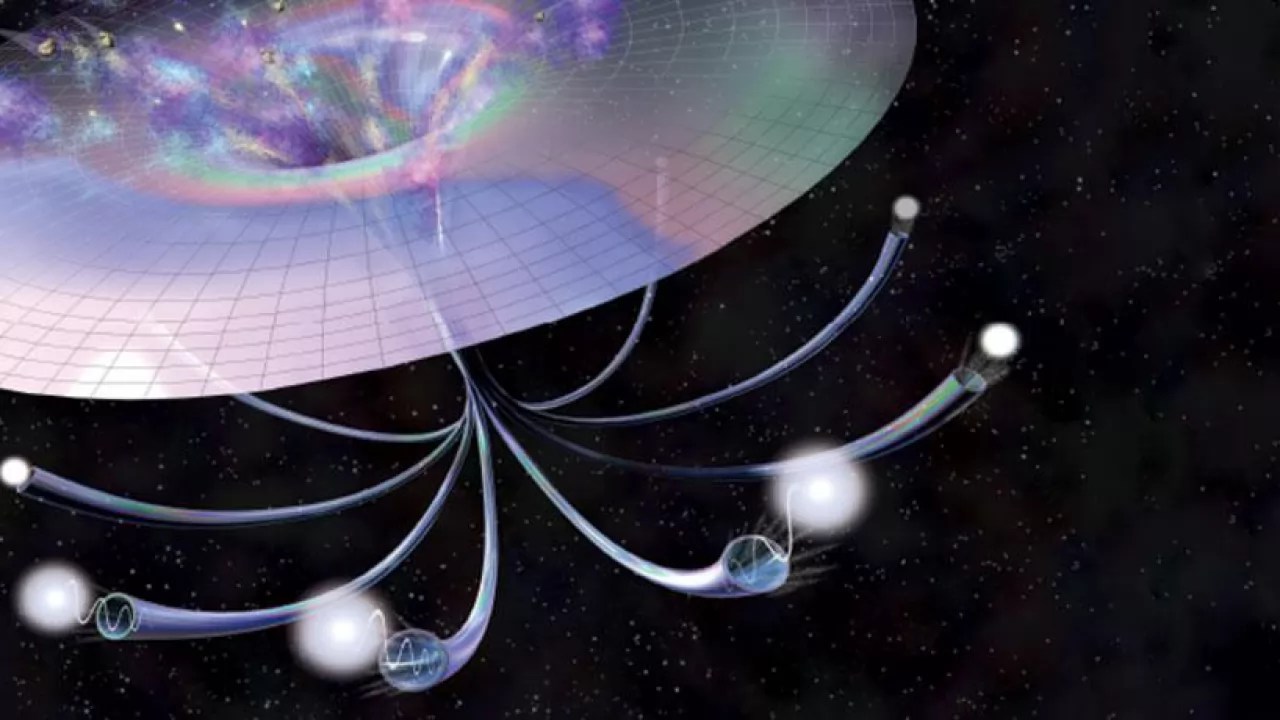
And yet, if the inflationary multiverse is truly infinite — either in spatial extent or in duration — then there must be copies of our entire Universe somewhere within it, including copies of ourselves that are identical to how we are now. If string theory, and the idea of the string landscape being fully populated within the inflationary multiverse, is indeed correct, then we could have nearly-identical versions of ourselves out there as well.
However, while the inflationary multiverse gives us ever-growing places to hold all of these possibilities, places that grow at an exponential rate, the number of possible outcomes for a matter-and-radiation-filled universe grows even more quickly: in a combinatoric fashion. These discrete and continuous possibilities for each quantum process results in a greater increase in what’s possible than the rate at which inflation generates more and more potential space for those possibilities to exist. As time goes on, the fraction of universes that admit a “copy” of our current observable Universe gets smaller and smaller, always asymptoting toward zero.
While parallel universes are a fun thought experiment and a playful arena for theoretical physicists to explore ideas, there is no evidence at all that this idea has any tie to the reality that we inhabit. While theory allows us to imagine worlds and universes that might be, it’s vital to keep in mind that most of them, and perhaps even all of them, are worlds and universes that never were.
Send in your Ask Ethan questions to startswithabang at gmail dot com!