The 16 stories that shaped physics and astronomy in 2024
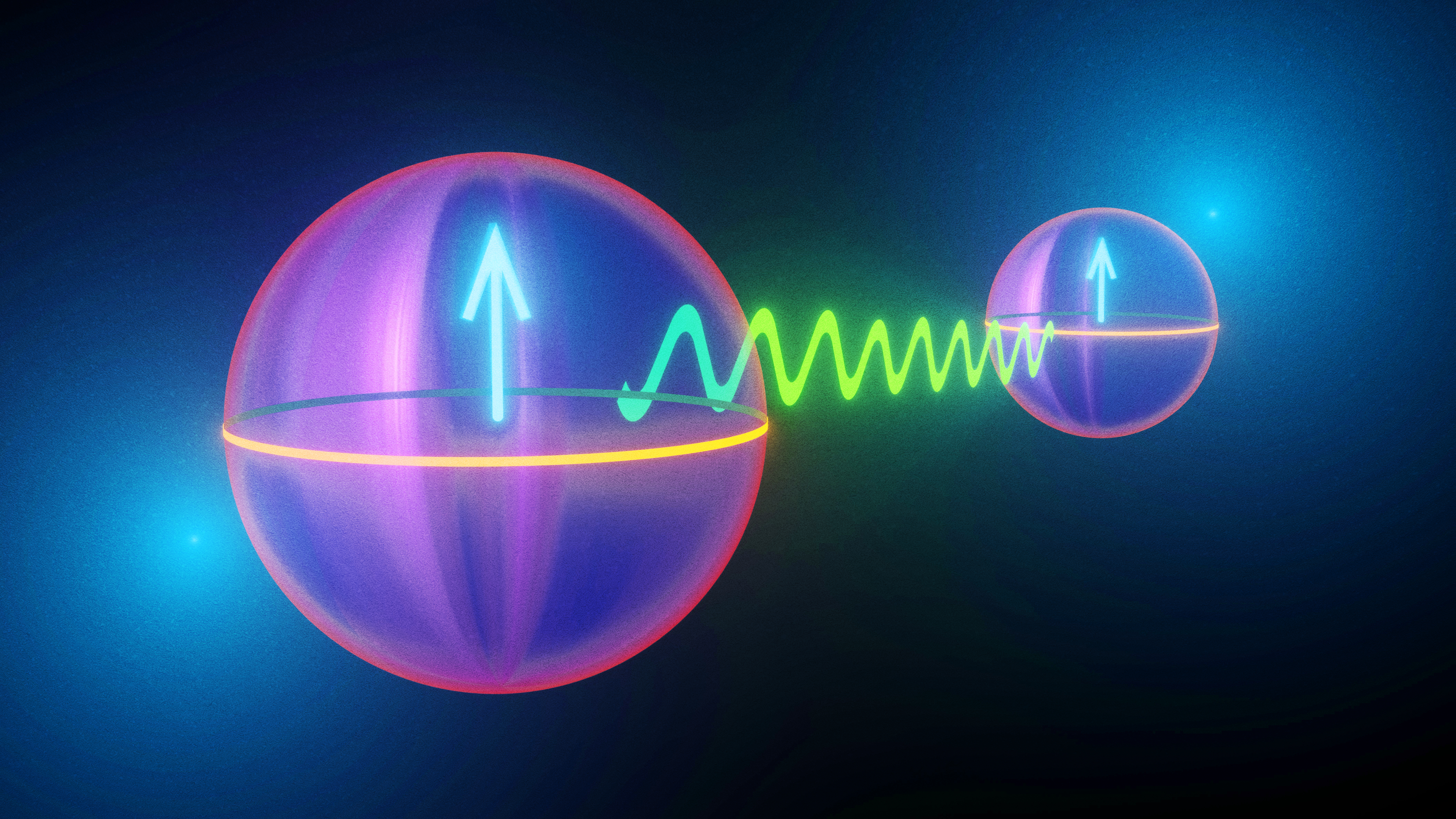
- Each year, a series of wildly speculative, unsubstantiated stories substitutes for actual “science news” in the mainstream, while actual science steadily progresses, although it’s largely unheralded.
- Still, this year saw many major advances in our understanding of the Universe, including in the areas of particle physics and astrophysics.
- These 16 stories, many of which you might never have heard of, are a snippet of the progress that’s occurred in 2024. Buckle up, because what we know about the Universe isn’t the same as it was a year ago.
In a year where science stories seemed to be dominated by rocket launches, hype surrounding artificial intelligence and quantum computing, and how political meddling threatens the enterprise of science itself, it might seem like basic science is stagnating. But that’s not true at all; that’s an indictment of how little news coverage is actually given to advances in science, because it’s been advancing forward just as steadily as ever throughout the entire year. On theoretical, experimental, and observational fronts alike, scientists have taken a great many steps towards a better understanding of our shared reality all throughout 2024.
While it’s always a challenge to narrow down any year-end list of how things have progressed throughout the calendar year, this list of 16 stories from the worlds of physics and astronomy focuses on things we’ve learned or achieved that were either unknown, uncertain, or more poorly understood at the start of the year than at the end. Without further ado, here are the top 16 stories that shaped physics and astronomy throughout 2024, including some that not only may surprise you, but that often surprised the researchers who uncovered these findings for themselves.
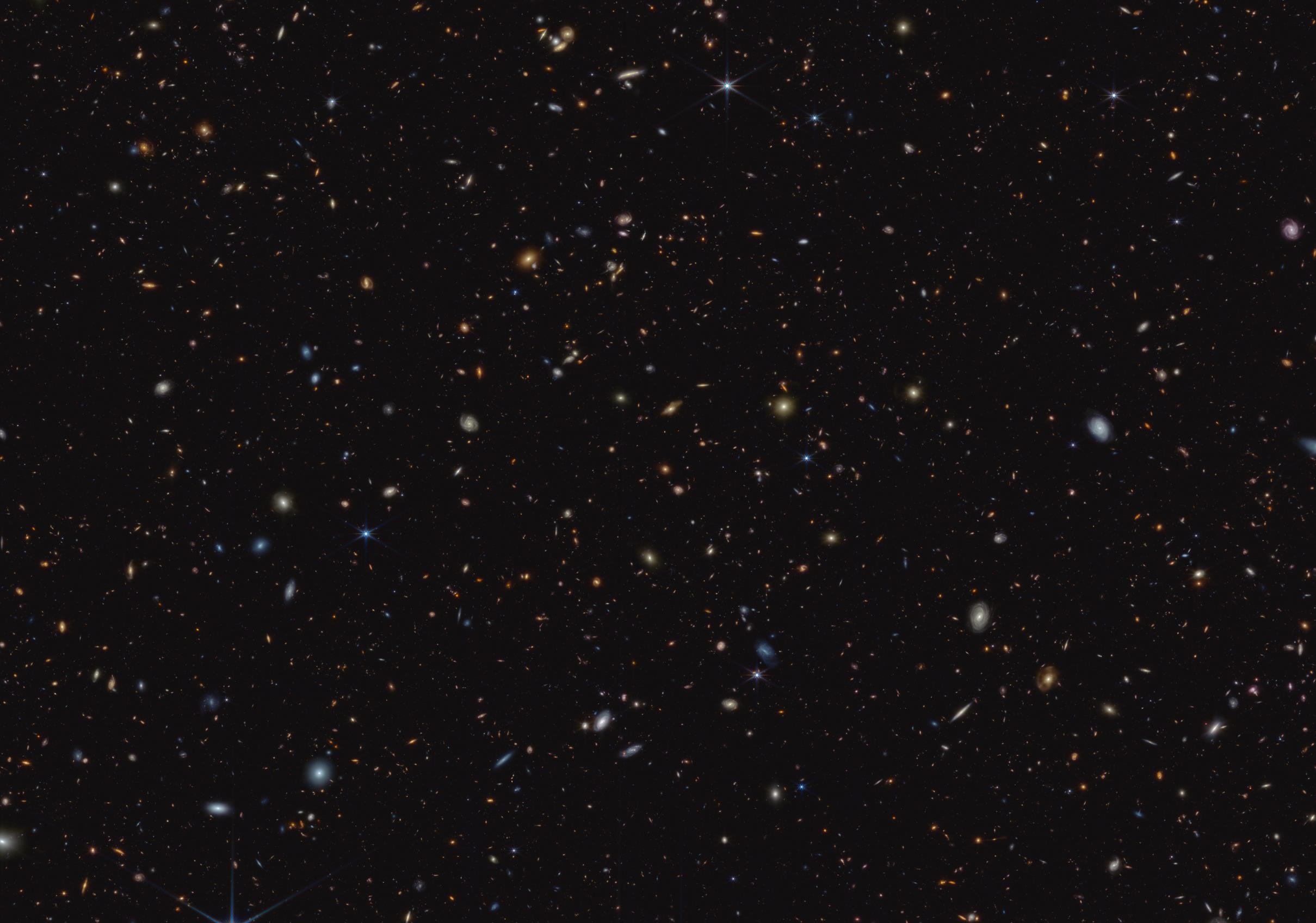
1.) The tiniest galaxies, not the largest, brightest ones, primarily reionized the Universe.
Just 380,000 years after the start of the hot Big Bang, our Universe stably formed neutral atoms. Hundreds of millions of years later, starlight streamed through the depths of space, traveling unimpeded until it runs into another object. And somewhere, in the in-between times, the earliest stars and galaxies reionized those neutral atoms, enabling that starlight to travel through space without getting absorbed.
Was it the numerous large, bright, massive galaxies that formed early on in cosmic history that did it? Nope; as shown by several teams using the James Webb Space Telescope (JWST), those big galaxies can only account for 20%, at most, of the light needed to reionize the Universe. The remaining 80% or more, as JWST observations showed, must have come from the far more numerous, but far more modest tiny dwarf galaxies that existed early on. It’s a cosmic lesson that “strength in numbers” is often stronger than the brightest, biggest, most massive outliers can be.

2.) The first candidate glueball, or bound state of hadrons with no valence quarks, was discovered.
When you talk to people about experimental particle physics, most of them act like the Large Hadron Collider (LHC) is the only game in town. While it’s certainly the most energetic collider ever built, there’s an enormous amount of physics that can be probed at lower, more modest energies with extreme precision: at electron-positron (rather than hadron) colliders. A whole slew of exotic, short-lived particles can be created and studied with great precision, enabling us to detect and test their properties.
One fascinating particle, the X(2370), was shown to exhibit properties that suggest it isn’t made up either of quarks, antiquarks, or both, but instead was a pure bound state of gluons alone: the first glueball. Predicted by our theory of the strong interactions but never observed, this particle is surely going to be studied further in subsequent years. If it does turn out to be our first bound state of gluons, alone, it would significantly advance our understanding of how the strong force works, while providing a new challenge for those who work on the theoretical side, particularly in the field of Lattice QCD.
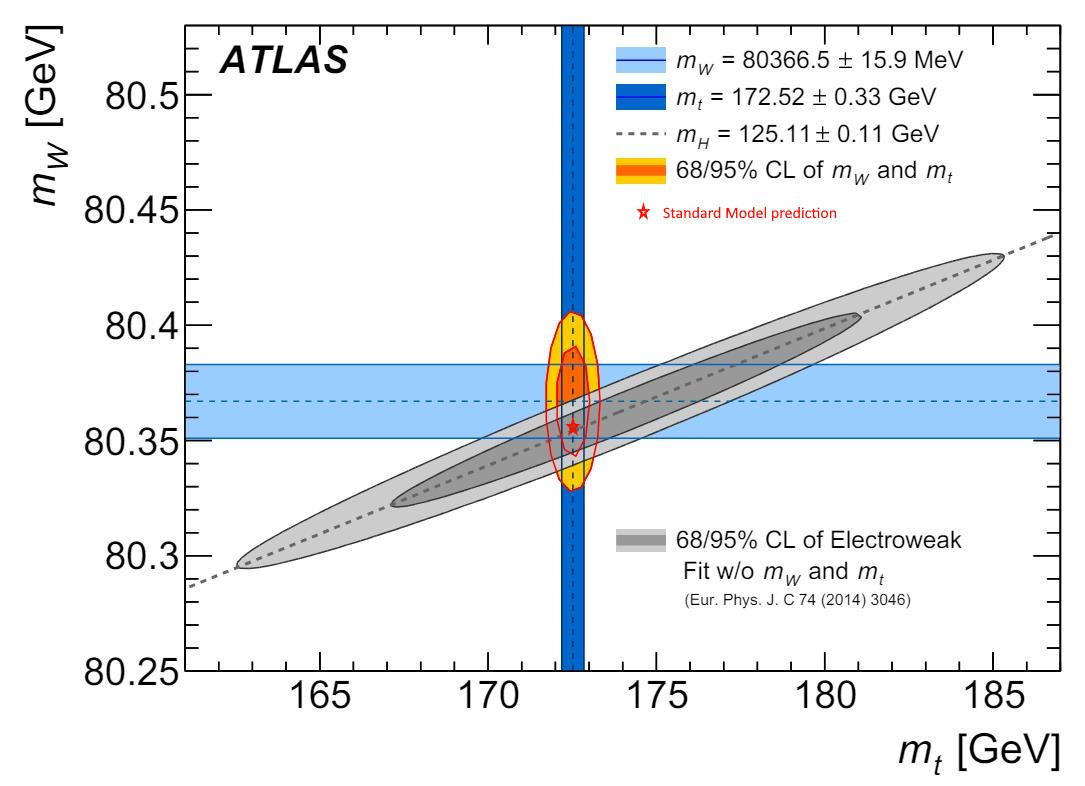
3.) The mass of the W-boson, contradicting earlier (Fermilab) measurements, supports the Standard Model.
Back in 2022, the CDF collaboration from Fermilab published an extraordinary claim that surprised many: they reported a mass of the W-boson, with the smallest errors/uncertainties ever, that conflicted with the predictions of the Standard Model. Combined with the known measurements of the top quark and the Higgs boson mass, the value they had arrived at suggested that something was either wrong with the Standard Model, implying new physics, or with their experiment and analysis, implying that data from the LHC would eventually contradict their results.
That latter option is precisely what happened earlier in 2024: the ATLAS collaboration announced their results for the W-boson’s mass, and it was not only consistent with the Standard Model’s predictions, it refuted and contradicted the CDF result. Two years ago, the data seemed to suggest that Fermilab’s results blew a hole right through the Standard Model. As of this year, the LHC has shown that that hole, most likely, is attributable to some hitherto unidentified error in the CDF collaboration’s analysis, and that the Standard Model is perfectly healthy with respect to collider data.
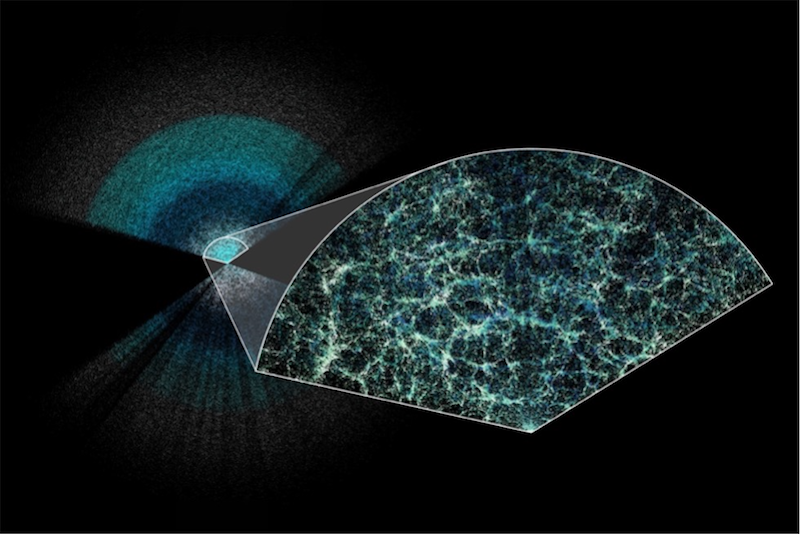
4.) Physicists now question the “heat death” fate, driven by constant dark energy, of our Universe.
Back in the late 1990s, astronomers gathered evidence that pointed to a new form of energy present in our Universe: dark energy. Over the next 25 years, almost all of the cosmic evidence pointed to a picture where:
- dark energy truly exists,
- it represents the dominant form of energy in our Universe at present,
- and where it’s been observed to be consistent with the simplest form of dark energy imaginable, a cosmological constant.
Under these conditions, dark energy will maintain a constant energy density forever, driving all unbound structures in the Universe, such as individual galaxy groups and clusters, including our Local Group, farther and farther away from one another as time goes on. It leads to a cold, empty, lonely Universe: a “heat death” fate.
But new evidence from the DESI collaboration, with the most precise large-scale structure data ever, throws this fate into doubt. Instead of a constant dark energy, they provide evidence for a Universe where dark energy is evolving, and in particular, where it has weakened substantially over the most recent few billion years of cosmic history. As we look ahead to data from ESA’s Euclid, NSF’s Vera Rubin Observatory, and NASA’s Nancy Grace Roman Observatory, the question of whether dark energy is constant or whether it evolves with time will get a definitive answer, and cosmologists everywhere will be watching.
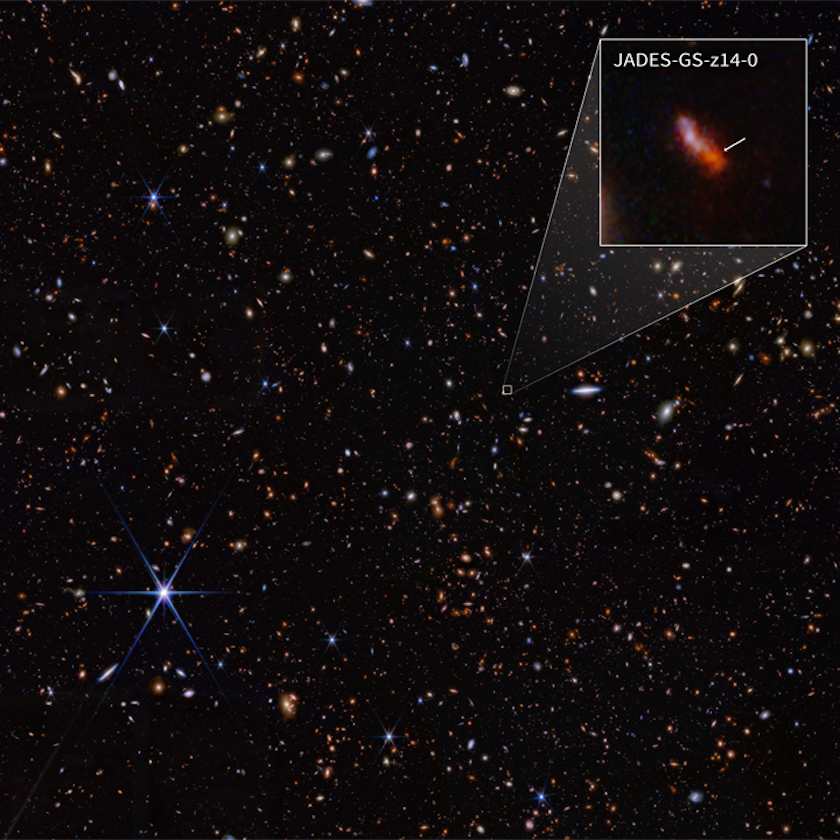
5.) JWST galaxy JADES-GS-z14-0 discovered, breaking the cosmic record for most distant object.
Back in mid-2022, just before JWST’s science operations officially began, the most distant cosmic object known was GN-z11, discovered by the Hubble Space Telescope. As 2024 draws to a close, that galaxy now isn’t even in the top 10 of most distant objects, as all 10 spots are now held by galaxies either discovered or spectroscopically confirmed by JWST. This year saw a new galaxy rise to the top of that leaderboard: galaxy JADES-GS-z14-0, discovered as part of the JWST Advanced Deep Extragalactic Survey (JADES) collaboration.
It’s incredibly bright and massive for its early time in cosmic history, as its light arrives from a time when the Universe was only 285-290 million years old, or just 2.1% of its current age. It’s also very unlikely that this galaxy will hold the record for very long; plenty of candidate galaxies appear to be at even greater redshifts/distances, and are just awaiting spectroscopic confirmation. With another 20 years of JWST science data to look forward to, this is one record you can bet will fall many times over in the years to come during the 2020s and 2030s.

6.) First samples of the lunar far side returned to Earth, potentially solving the mystery of the Moon’s two faces.
Ever since humanity snapped the first images of the lunar far side during the early stages of the space race, we’ve wondered at why the near side and far side of the Moon look so different from one another. The near side has abundant dark “seas” of basaltic material: lava flows that filled in enormous depressions known today as the lunar maria. The far side? It’s at significantly higher elevation than the near side, with only a few small maria. It’s led to an incredible mystery of why these two faces of the Moon are so different from one another.
One compelling and fascinating theory is about to be put to the test, thanks to the sample return mission of China’s Chang’e-6 mission. While the Apollo missions brought a great many moon rocks back to Earth, they were all from the lunar near side. Now, with the Chang’e-6 samples, we have samples from the lunar far side here on Earth as well. Although the samples still await the critical analyses, it’s eminently possible that we already have the data in hand needed to validate or invalidate our picture of how the Moon formed and how the near side and far side came to be so different. Stay aware of this one!

7.) Intermediate-mass black hole discovered: the “missing link” in black hole physics.
Throughout the Universe, two types of black holes have been shown to be quite common. First, there are stellar mass black holes, known to arise from the collapse of massive stars in the late stages of their lives. They range anywhere from a few solar masses up to perhaps a couple of hundred solar masses, and can also form from the mergers of lower-mass objects like neutron stars or from other black holes. And second, there are supermassive black holes: behemoths found at the centers of galaxies that range from millions to billion of solar masses.
But where are the in-betweeners? Where are the intermediate-mass black holes that fall in between those two main populations?
Thanks to new research that tracked the long-term orbits of stars near the center of globular cluster Omega Centauri, we now know of at least one: an 8200 solar mass black hole at the core of one of the Milky Way’s most massive globular clusters. This intermediate-mass black hole represents the first irrefutable evidence for such a population of objects, and is the first “missing link” between stellar mass and supermassive black holes.
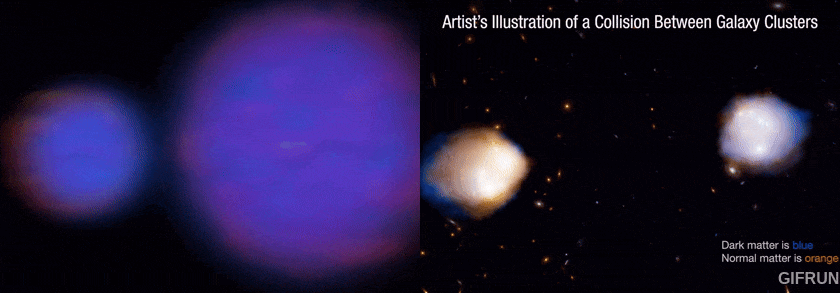
8.) The puzzle of ultra-fast galaxy cluster collisions is now solved.
According to our theory of cosmic structure formation, galaxy clusters should exhibit certain ranges of speeds when they collide, of anywhere from ~1000 km/s up to around ~4000 km/s, but probably not much greater. These colliding cluster systems provide strong evidence for the existence of dark matter, as they show a separation between normal matter (identifiably from X-ray emissions) and the observed gravitational signal (inferred from gravitational lensing).
However, the first such system, the Bullet Cluster, was reconstructed to have a collision speed of ~5400 km/s, throwing this interpretation into doubt.
Doubt no more, world. Here in 2024, we discovered a very similar system but happened to catch the collision oriented along our line-of-sight, enabling us to measure the cluster collision speed more directly. What we found was fascinating: the speeds observed were more than ~1000 km/s less than we would have inferred previously, as new shocks and astrophysics involving the circumgalactic medium were found to be very important. Knowing that we’ve likely overestimated the speed of the Bullet Cluster (and possibly others) makes this puzzle disappear, cementing dark matter as an ingredient of our Universe.
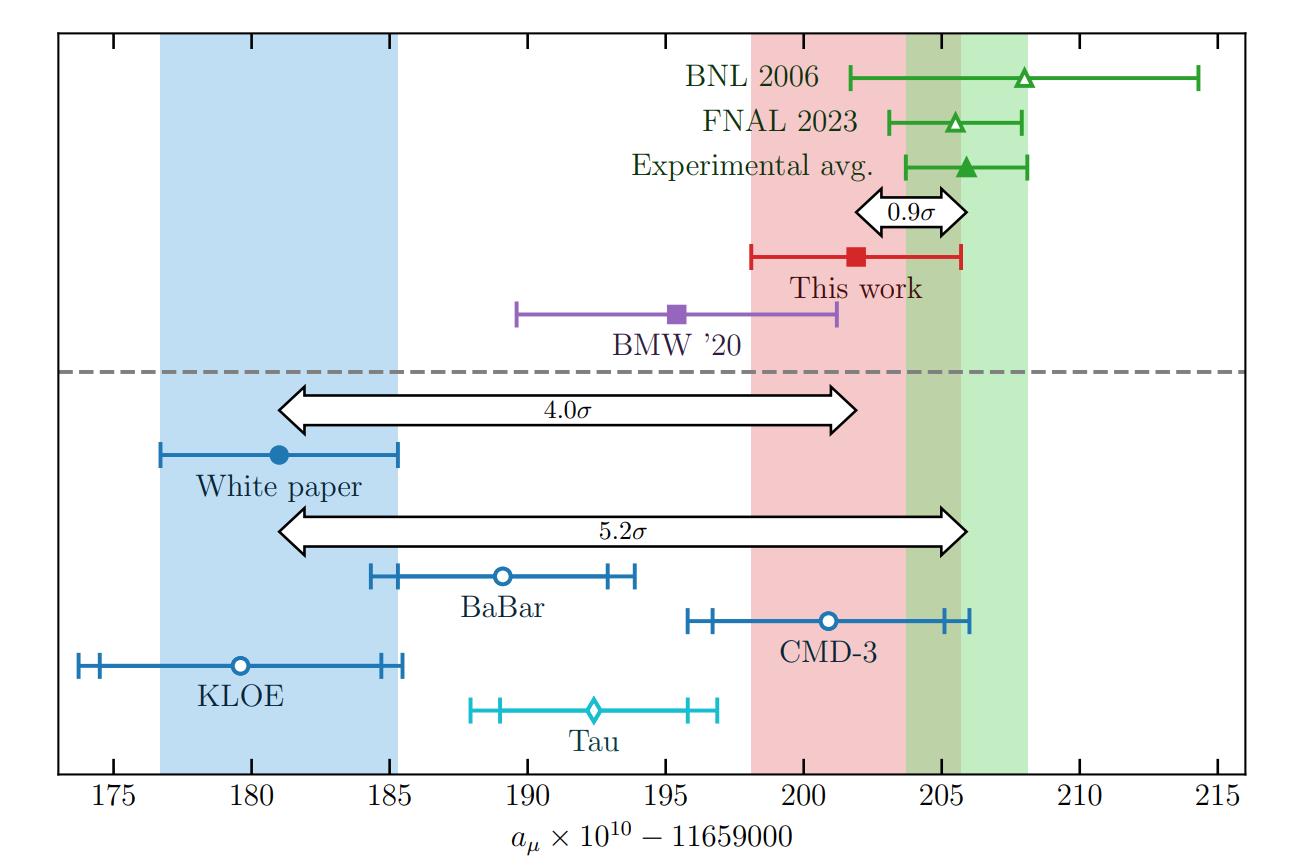
9.) New lattice QCD calculation eliminates the muon “g – 2″ discrepancy.
Every charged particle we know of also has an inherent “spin” to it, and that means it must also have an inherent magnetic moment. This gyromagnetic ratio, known as g, is something physicists have worked hard to both measure and calculate, as any differences could indicate new physics beyond the Standard Model. The muon, the heavier cousin of the electron, is of significant interest for this endeavor, as an experiment being conducted at Fermilab has been getting more and more precise, and its results appear to conflict with theoretical calculations that have long been conducted.
But the theoretical approach has significant uncertainties, and a different approach aims to calculate those “uncertain” terms directly: Lattice QCD. Earlier this year, in July of 2024, a major advance happened, as a Lattice QCD team published a direct refutation of earlier theoretical papers, instead bringing the new theoretical prediction directly in line with Fermilab’s observations. While the experimental data is needed to tell us what our Universe is doing directly, proper theoretical calculations are equally necessary. With more work to be done, this is the strongest evidence yet that there is no discrepancy for the muon, and that the Standard Model is likely to hold here as well.
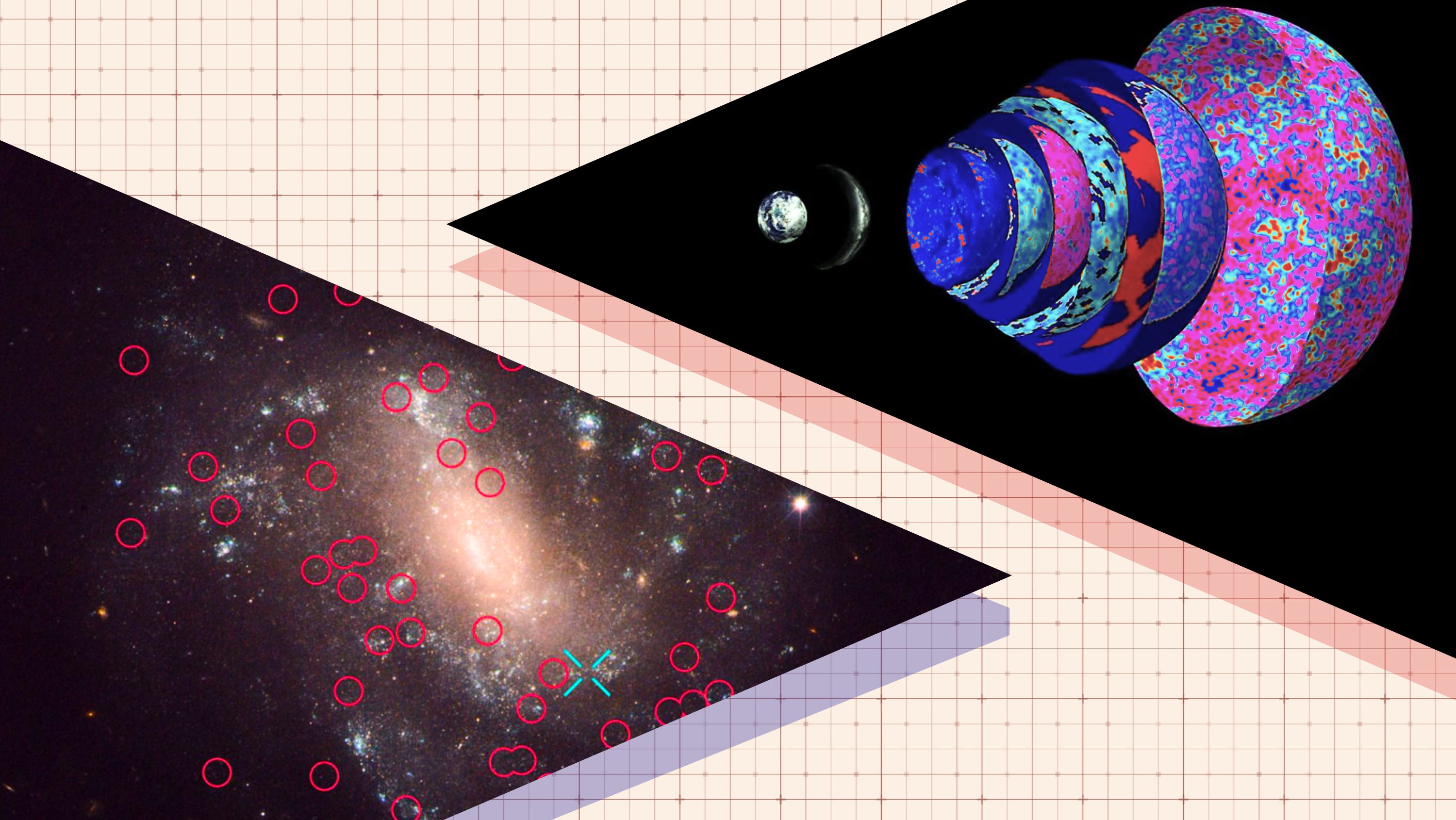
10.) The Hubble tension is stronger than ever, and is strengthened, not questioned, by new JWST results.
Earlier this year, the Carnegie-Chicago Hubble Project (CCHP) collaboration released information that shocked the world: they claimed that using data from JWST, they arrived at a new measurement of the expansion rate of the Universe that was lower than all other values using similar methods, of ~68 km/s/Mpc. Whereas all “distance ladder” methods seem to converge on a value of ~73 km/s/Mpc and all “early relic” methods prefer a lower value of ~67 km/s/Mpc, their announcement of a distance ladder result that preferred a low value offered an alternative story: one where the Hubble tension might disappear. It led to the CCHP leader, Wendy Freedman, being the only astrophysicist named to Nature’s year-end list of top 10 science leaders who influenced science in 2024.
The only problem?
The new measurements actually strengthen and intensify the Hubble tension, rather than relaxing it. JWST results decrease the errors on the measurements of these galaxies, and it’s only by cherry-picking a few galaxies from a more full sample that a lower-value result is obtained; when you look at the full suite of data, the tension is stronger than ever. There’s a reason why, six months after that paper was submitted, it still hasn’t passed peer review. Expect the Hubble tension to remain a topic among cosmologists for years to come; we’re no closer to solving this puzzle despite some splashy claims that were made here in 2024.

11.) Largest black hole jets ever, at a scale of 23-24 million years, discovered.
Of all the individual objects in the Universe, black holes are the most massive. When they feed on matter, they can accelerate it, cause it to heat up and ionize, and then those charged particles can be kicked with incredible energies. Over time, they can reach extraordinary distances, as seen in a variety of galaxies near and far. In 2024, we discovered the longest, strongest black hole jets ever seen: jets that extend for somewhere between 23-24 million light-years in overall length. The object in question, Porphyrion, breaks the old record by some ~6 million light-years.
It’s the longest, strongest black hole jet ever discovered, and I was lucky enough to score an interview with Dr. Martijn Oei, the researcher who led the discovery. With black hole jets on the scale of the cosmic web, it now leads to the fascinating possibility that individual objects, such as these supermassive black holes, can create features on the scale of the cosmic web. That’s a new discovery that happened just this past year, here in 2024.

12.) Even top quarks, the shortest-lived particles of all, exhibit quantum entanglement.
Quantum entanglement is one of the spookiest, most counterintuitive phenomena in all of physics. Awarded the 2022 Nobel Prize in Physics, it’s the only way we know of to do better than “random guessing” when it comes to predicting the measured outcomes of quantum particles.
But is there a “speed limit” to quantum entanglement, or is it truly an instantaneous phenomenon?
One such test we long sought to perform was to look at the shortest-lived particles of all: top quarks. With a mean lifetime of just 5 × 10-25 seconds, they decay before ever forming bound states like mesons or baryons. Yet here in 2024, scientists using data from the LHC were able to demonstrate that not only do top quarks, the shortest-lived particles of all, demonstrate quantum entanglement, but that the entanglement also appears to be instantaneous. It’s another victory for the standard predictions of quantum physics and the Standard Model, and another experimental challenge that our leading theories have now passed.

13.) A new speed record has been set for spacecraft launched by human beings.
This one hasn’t actually happened just yet, but is about to. On December 24, 2024, the Parker Solar Probe (which just had its orbit changed by its most recent, and final, gravitational encounter with Venus) will pass closer to the Sun than ever before, traveling faster than any spacecraft in history as it reaches perihelion: its closest approach to the Sun. At that moment, it will:
- come within 8.86 solar radii of the Sun’s limb,
- just 6.2 million kilometers or 3.8 million miles away,
- achieving a maximum speed of 192 km/s (119 mi/s) in the process.
The Parker Solar Probe will continue on this orbit for the remainder of its mission life, until its instruments fail from the incredible heat and radiation emanating from the Sun. Faster than the Voyager, Pioneer, or New Horizons spacecrafts, it’s a testament to the fact that if you want to go fast, falling into the Sun leads to greater speeds than any attempts to escape from it.
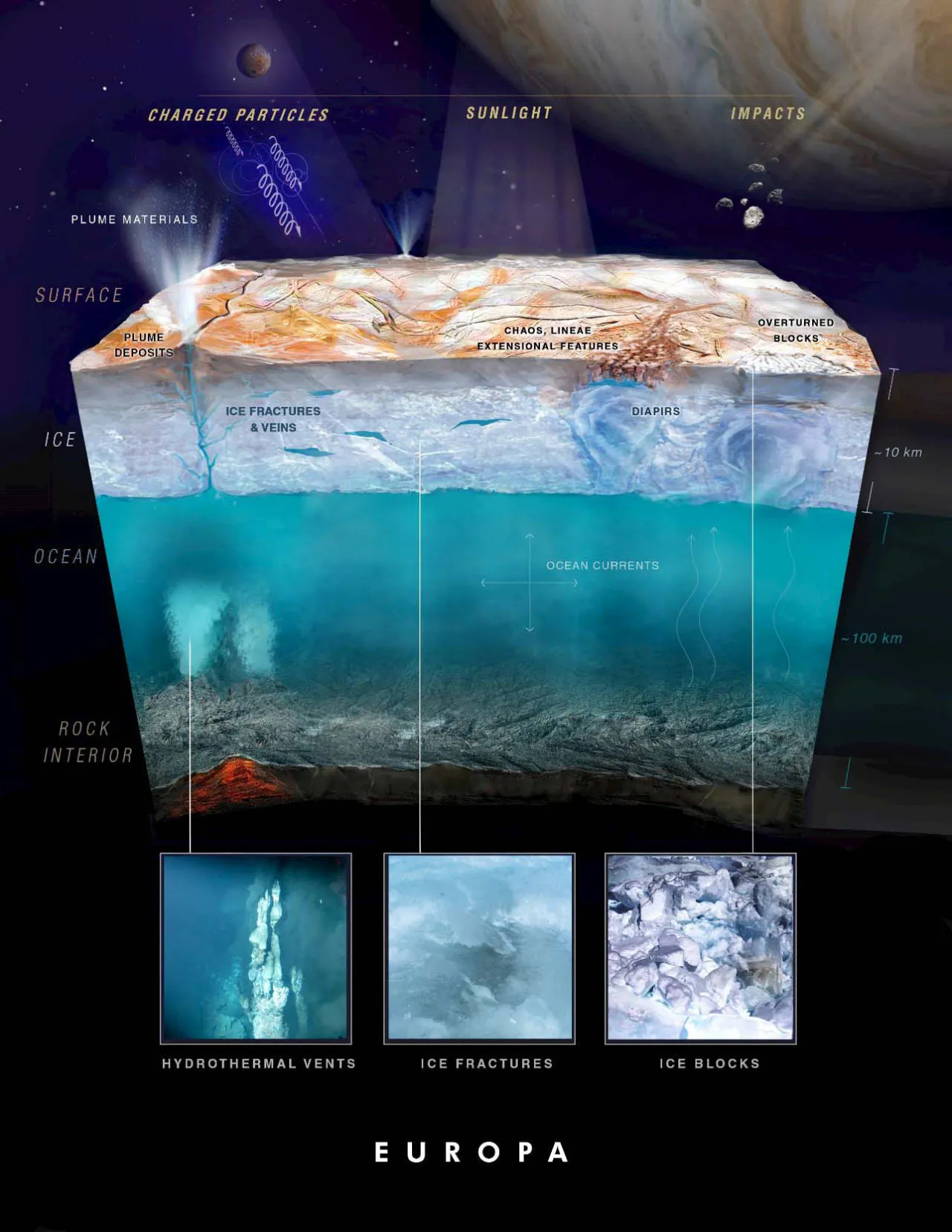
14.) Our first mission to Europa, a large moon of Jupiter with a suspected subsurface ocean, launched successfully in 2024.
Is there life elsewhere in the Universe? Why not look in our own backyard, and check out the worlds of our Solar System? Europa is a fascinating candidate: the second Galilean moon of Jupiter, it appears to have an icy crust that houses a suspected subsurface ocean beneath it. The Europa Clipper mission, launched by NASA in October of 2024, will be the first dedicated mission to an ice-covered world with a known subsurface ocean ever, and will arrive at Jupiter’s moon Europa in 2030 after two gravity assists, from Mars and Earth, in 2025 and 2026 respectively.
Although we have to wait for 2030 to get our first results, the launch was successful and the Europa Clipper mission is well on its way. This is the one rocket launch from 2024 that deserves an end-of-year-highlight, as this mission truly has the potential to change how we view our Solar System, and the hunt for extraterrestrial life, all in one fell swoop.

15.) Vega, a nearby young star, shows little evidence for planets, belts, or features in its debris disk.
Over the past few decades, we’ve learned a whole slew of lessons about planetary systems. Planet formation usually commences just 1-2 million years after a star’s birth, and usually completes just a few million years later. Almost all stars with a certain fraction of heavy elements, more than about 10% of what’s present in the Sun, are seen to have planets around them. And in the JWST era, we examined the nearby young star Fomalhaut, a star with a debris disk around it, and found that it didn’t just have an asteroid belt analogue and a Kuiper belt analogue, but a third, intermediate belt as well.
And then there’s Vega.
The “other” nearby, bright star with a debris disk around it, Vega is a rapidly rotating star viewed almost perfectly face-on from Earth. But when JWST looked at it, it found a big surprise: almost nothing. No gaps in the disk, no belts, and no evidence for planets. In fact, there can be no Jupiter-mass, Saturn-mass, or Neptune-mass planets in this system at all; the heaviest a planet can be and still be consistent with the data is 6 Earth-masses.
So where are Vega’s planets, belts, and the other features we expected it to have? That’s a question for 2025 and beyond!

16.) B-meson decays hint at physics beyond the Standard Model.
And finally, in a paper that was just published a few weeks before the end of the year, scientists using data from the LHC have assembled evidence that B-mesons, particles that contain a bottom quark or antiquark, may decay in ways that defy the Standard Model’s predictions. Like many such hints that came before it, this one may go away, or “regress to the mean,” with more and better data, which is precisely what the LHC is collecting. But there’s always a first “hint” of any new physics that’s ever been discovered, and when it comes to beyond the Standard Model physics, we’re still looking for it.
Could B-meson decays be the first crack revealed at particle colliders in the Standard Model itself?
Time will tell. But headed into 2025 and beyond, it’s one place that physicists will be sure to focus on when it comes to the intersection of theory and experiment. There’s still a whole Universe out there to discover, and 2024 saw us learn more and more about it, increasing our knowledge to its highest peak ever. The more we learn, the more we refine the questions we need to be asking, and 2024 was an exceptional year for these (and many other) advances in exactly that regard.