Ask Ethan: Does the CMB really “prove” the Big Bang?
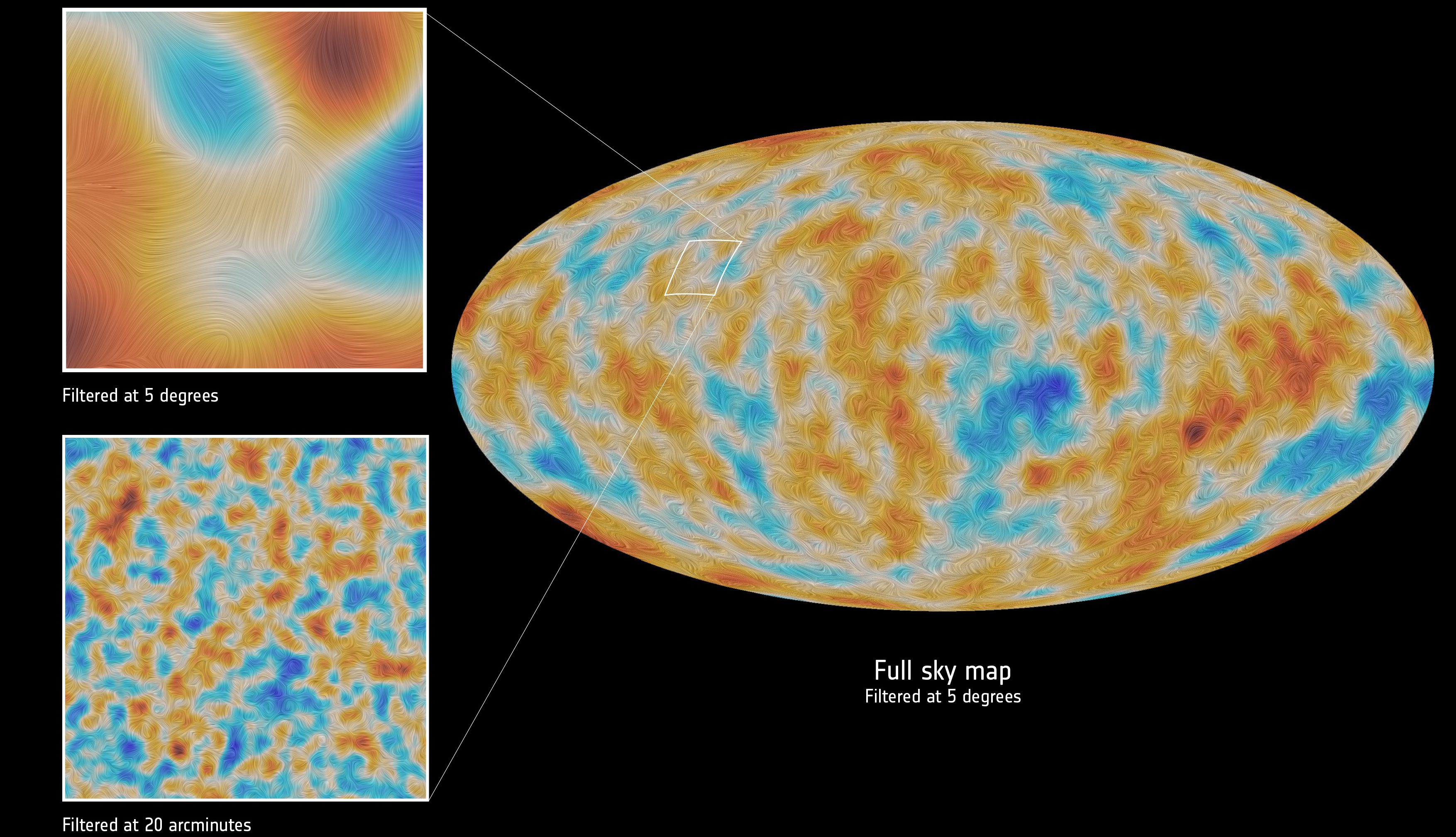
- Ever since its discovery in the mid-1960s, the cosmic microwave background has been identified with the Big Bang: as a cosmological relic from the extremely early Universe.
- However, many have floated the possibility that it’s not of primordial origin, but rather is a late-time signal or even a foreground, like a “fog” polluting our Universe.
- Could these non-cosmological explanations be viable? How confident are we that the CMB actually “proves” the Big Bang? Extremely. Here’s the science behind why.
No matter how strong the evidence is in favor of a scientific theory, there will always be contrarians who come along and encourage the exploration of alternatives. This isn’t necessarily out of stubbornness, but is often done in an attempt to subject even our most well-supported theories to rigorous scrutiny and ever-stronger testing. However, oftentimes these contrarian positions have to ignore a substantial amount of evidence in order for their preferred alternatives to survive. As cosmologist Mike Turner once famously put it, “You can only invoke the tooth fairy once,” meaning that you might be able to justify a scenario with one major modification, but once you have to start doing multiple cartwheels to get around a mountain of evidence, your alternative idea is a lost cause.
Undeterred by that notion, many — mostly crackpots but including a few serious scientists — have challenged the “cosmic” interpretation of the observed cosmic microwave background (CMB), going all the way back to Fred Hoyle some 60 years ago. Are we 100% confident that the CMB really qualifies as “smoking gun” evidence for the hot Big Bang? That’s what Tom Tracy wants to know, asking:
“Some detractors of the Big Bang theory maintain that the CMB — one of the strongest pieces of observational evidence for the Big Bang theory — does not have a cosmological origin. How are most cosmologists confident that the CMB is a clean probe of the early Universe rather than the result of a foreground, as these critics suggest?”
I typically answer a question like this once per year, so this time, let’s go even more in depth and probe this deeply. By the end, even the most die-hard skeptics will understand why the CMB’s cosmic origin is thoroughly beyond a reasonable doubt.
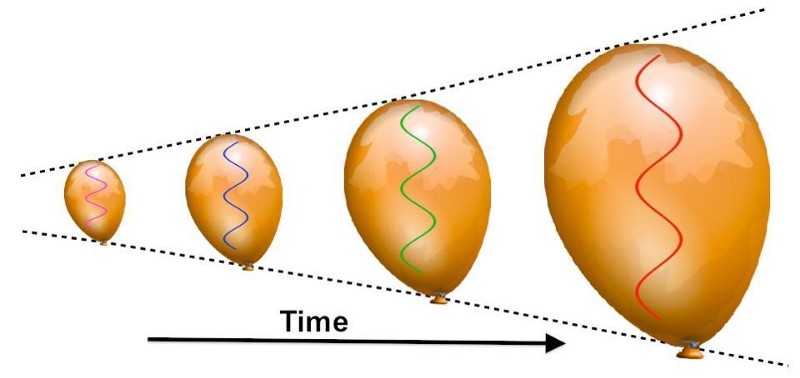
The idea of the Big Bang is relatively simple: that the Universe was smaller, hotter, denser, and more uniform in the past, and has grown and evolved to be the way we see it today because it’s expanded, cooled, and gravitationally clumped together over time. This was expected by scientists as early as the 1920s, when a theoretical prediction and a set of observational measurements both began to come together.
- First, in 1922, Soviet scientist Alexander Friedmann derived a set of equations from Einstein’s general relativity that described the behavior of a Universe that was, on average, uniformly filled with one or more species of matter and/or energy. He found that such a Universe could not remain static and stable, but must either expand or contract.
- And then, starting in 1923, Edwin Hubble began measuring the distances to extragalactic objects. Combined with the earlier observations of Vesto Slipher — which measured the amount by which the emitted light from those objects had been redshifted — they indicated that the farther away a galaxy was, the faster it appeared to recede from us.
These two facts, when put together, seemed to indicate that the Universe was expanding, and that the expansion rate could even be measured by plotting the distance versus the redshift of galaxies beyond the Milky Way.

The first to put this together was Georges Lemaître, who did it back in 1927. Einstein wasn’t impressed, writing to Lemaître, “Vos calculs sont corrects, mais votre physique est abominable,” which means “Your calculations are correct, but your physics is abominable!” Nevertheless, others swiftly came to the same conclusion. Howard Robertson derived the expanding Universe in 1928, and then Hubble published his major work in 1929. By the 1930s, the expanding Universe was undeniable, as all other alternatives — including tired light theories, a special relativistic Doppler shift alone, and an eternal static Universe — were shown to be inconsistent with the data.
But this, alone, isn’t enough to prove the idea of the Big Bang; it merely admits it as one possibility. In order for that possibility to become the leading theory, it must make concrete predictions that differ from all of its alternatives, and then we must go out and collect the critical evidence that either confirms and validates or otherwise refutes those predictions. The first serious attempts at these predictions were made in the 1940s by George Gamow, himself a former student of Friedmann’s, and his collaborators. In particular, they considered what you’d get for your Universe if you began from a hot, dense, uniform initial state and simply allowed everything to expand-and-cool, according to the laws of physics, from that point onward.

The three major ones that emerged were as follows.
- Initially, it would be so hot and dense that even atomic nuclei, made from protons and neutrons, couldn’t stably form. As the Universe cooled so that the first complex nucleus — deuterium — would be stable against being blasted apart, an early period of nucleosynthesis would arise, forming the nucleus of elements beyond hydrogen even before any stars formed. Even “pre-stellar” material, then, wouldn’t be truly pristine, but would consist of isotopes of hydrogen, helium, lithium, and so on.
- Then, conditions would cool further, and these heavy nuclei would finally be able to bind together with electrons without being immediately ionized again. This epoch, marking the first time that neutral atoms stably formed in the Universe, would also coincide with the photons (or particles of light) that were part of the primeval plasma ceasing to interact with the free charged particles that were present, as they would then all be bound in neutral atoms. Those photons would then free-stream: where they redshift and cool due to the expanding Universe, but where their time as part of the primeval plasma remained imprinted on them.
- And finally, once neutral atoms formed and the leftover radiation ceased interacting with it in any substantial way, that matter would clump together to form clouds of gas, which would then collapse and give rise to stars, galaxies, and the large-scale structure of the Universe: forming a grand cosmic web.
Along with the expanding Universe, these form the four cornerstones of the Big Bang model.
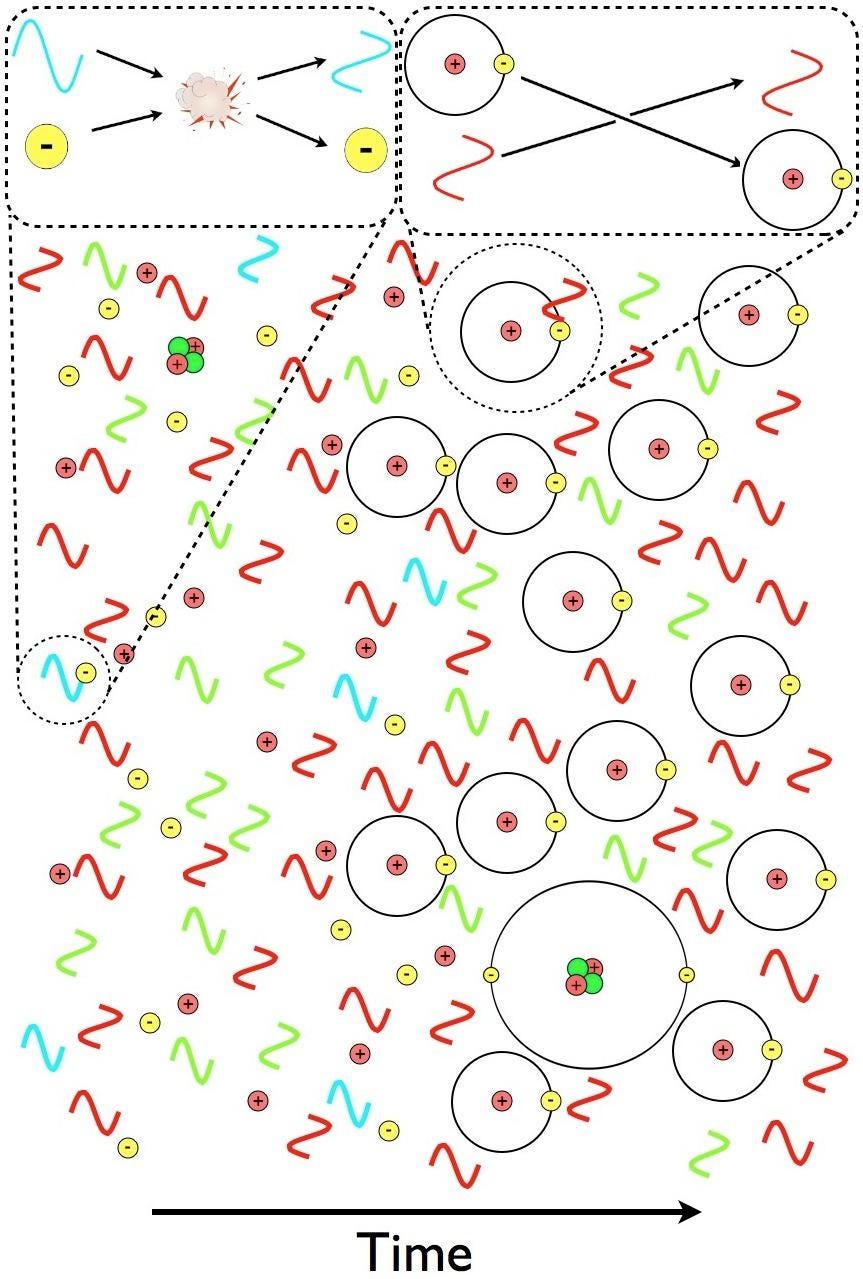
While Gamow’s camp spent most of the late 1940s and all of the 1950s primarily arguing about the first point (the abundance of the elements) with Fred Hoyle’s Steady-State camp, a team over at Princeton — led by Bob Dicke but also including Jim Peebles, David Wilkinson, and Peter Roll — began calculating the gory details of the predicted properties of that second prediction: the relic bath of radiation left over from a hot, dense, early period in cosmic history. In particular, that radiation ought to be:
- omnidirectional,
- the same temperature and energy density everywhere,
- just a few degrees above absolute zero,
- and blackbody in terms of its spectrum.
While the galactic plane was expected to get in the way, the remainder of the sky, except for wherever the Sun itself was located, should display identical properties for this radiation.
In the mid-1960s, it wasn’t the Princeton team (although they had designed and were building a radiometer with the intention of flying it to high altitudes to detect this radiation) that came through, but rather an unlikely duo: Arno Penzias and Bob Wilson. Working for Bell Labs and equipped with a powerful radar-detecting device — the Holmdel Horn Antenna — they saw a uniform background of noise, omnidirectionally, that they could not explain. After a variety of attempts at calibration and “zeroing” out the noise failed, including by cleaning pigeon nests and detritus out of the horn itself, they were shown the idea of this cosmic background of radiation. Unknowingly, they had discovered the “smoking gun” of the Big Bang.
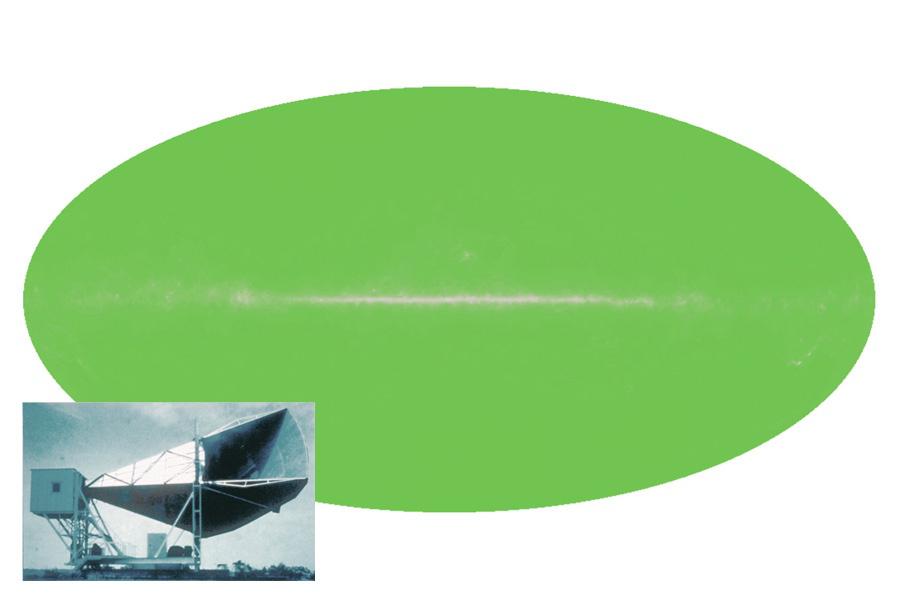
Very swiftly, the entire astrophysics community embraced this discovery. Here was a clear prediction of a theory that was observationally borne out with great precision. But there were still more checks to do. For one, the Penzias and Wilson observations only measured this radiation at one frequency. It takes measurements at a large number of other frequencies to confirm that the radiation is indeed blackbody in nature. Let’s explore why that is.
If you take a collection of particles and heat them up to a certain specific temperature, those particles won’t all move at the same speed and they won’t all have the same amount of energy. Instead, the particles will follow a very specific distribution as far as speed and (kinetic) energy go: a Maxwell-Boltzmann distribution. A prediction that emerges from the hot Big Bang picture is that even though photons all move at the same speed — the speed of light in a vacuum — their energy is distributed in precisely the same way as it would be for massive particles.
This means that if there truly was a hot, dense phase to the early Universe, one where photons and charged particles collided so frequently that they reached thermal equilibrium, a perfectly blackbody energy spectrum would be realized.

It would be as though the photons were a perfect fluid that was raised to a uniform temperature and then cooled for one reason alone: due to the cosmological redshift imprinted on them by the expansion of the Universe.
Today, that radiation is observed to be peaked at microwave frequencies: hence the name, cosmic microwave background (CMB). However, what would the spectrum of this radiation prove to be? It’s possible that the radiation wasn’t of primordial cosmic origin, but rather came about from some later phenomenon.
The leading candidate would be reflected starlight. Stars generate energy through nuclear fusion in their cores, and that energy propagates through the star until light gets emitted from their photospheres. If there were enough stars all throughout the Universe, and enough locations for that starlight to reflect off of (i.e., they heated dust grains up to a uniform temperature, and then those dust grains radiated blackbody radiation), then the spectrum wouldn’t be a perfect blackbody. Instead, it would appear as a sum of a large number of blackbodies, as all stars (even our Sun) emit light that can be best approximated as a series of surfaces at increasing temperatures the farther you descend into a star’s outer layers. The fact that the spectrum is not only observed to be a perfect blackbody, but in fact the most perfect blackbody ever observed in the entire Universe, is another confirmation of the Big Bang and a refutation of all non-cosmic alternatives for the origin of this radiation.
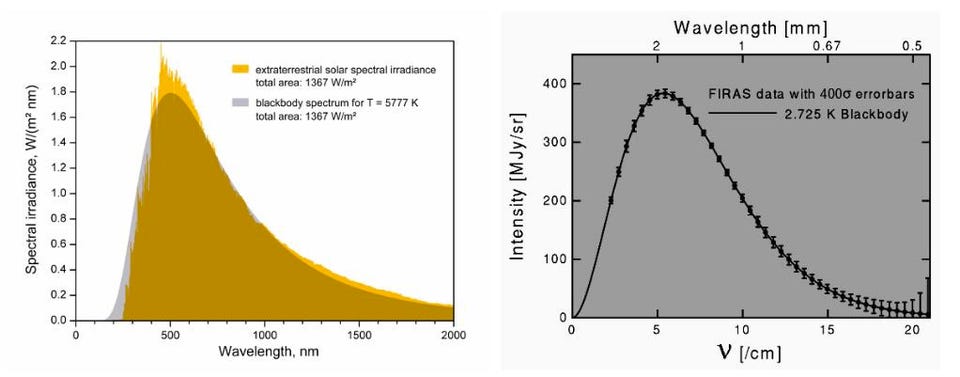
But we can go even deeper than this. The Big Bang theory also predicts that structure forms in this Universe through gravitational clumping. From a near-uniform set of initial conditions, the Universe has grown up by forming stars, galaxies, groups of galaxies, clusters of galaxies, and an even grander-scale cosmic web. On average, galaxies are expected to move relative to one another — and to whatever “rest frame” the CMB is a perfectly uniform temperature in — at anywhere from a few hundred km/s up to a couple of thousand km/s: a phenomenon known as peculiar velocity in cosmology. If we embarked on a quest to measure any imperfections in the temperature of the CMB, that’s the first thing we’d expect to see: evidence for our local motion relative to the CMB itself.
This was measured in the late 1970s for the first time, and it turns out that the combination of:
- the Earth’s motion around the Sun,
- the Sun’s motion around the Milky Way,
- the Milky Way’s motion within the Local Group,
- and the Local Group’s motion relative to the large-scale structure of the Universe,
all result in an apparent motion relative to the cosmic frame of reference that defines the CMB. We do, in fact, see that one “side” of the CMB is about 3.36 millikelvin higher than average, while the opposite “side” appears an equal-and-opposite amount colder than average. Those two sides are opposed to one another by exactly 180 degrees, and correspond to a cumulative motion of ~370 km/s relative to the rest-frame of the CMB itself. This measurement is completely consistent with the cosmic interpretation of the CMB, and has no other viable explanation.
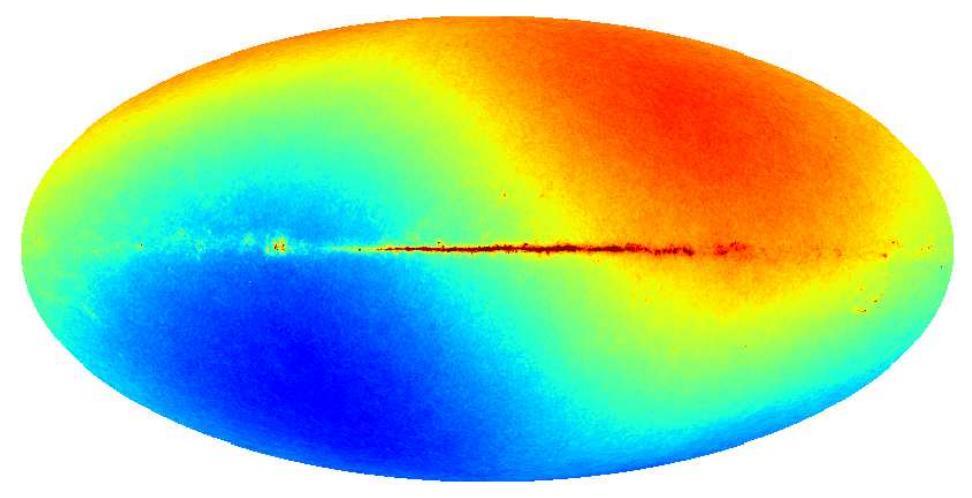
But that’s not all!
We can go even a step farther, and search for measurements of the tiny initial imperfections — or anisotropies — that must have been imprinted in the CMB from very early times. The Universe couldn’t have been born perfectly uniform, or there would be no large-scale structure today. Instead, there must have been a set of minuscule “seed” imperfections, or density fluctuations, that the Universe was born with, in order to produce the structure we see today. According to the theory of cosmic inflation, these fluctuations should be:
- isotropic and homogeneous (favoring no direction nor a location in space),
- of a small magnitude (less than 1-part-in-10,000),
- with a gaussian (random variable) distribution,
- with almost (but not perfectly) a scale-invariant spectrum,
- and should show up as a series of peaks-and-valleys on different angular scales in terms of the temperature differences between different regions.
We can make these measurements in two ways. First, we can actually observe the large-scale structure of the Universe, both today and at great cosmic distances, and can measure that the Universe is isotropic and homogeneous in structure, with a nearly-perfectly scale invariant spectrum, and where galaxy clustering reveals an “acoustic scale” of about 500 million light-years.
Then, alternatively, we can measure these fluctuations directly in the CMB itself. This was what the COBE, WMAP, and Planck satellites did so exquisitely in the 1990s, 2000s, and 2010s, respectively. The discovery of the form of these anisotropies, and again, their sparkling agreement with theoretical predictions, is why John Mather and George Smoot were awarded the 2006 Nobel Prize in physics.
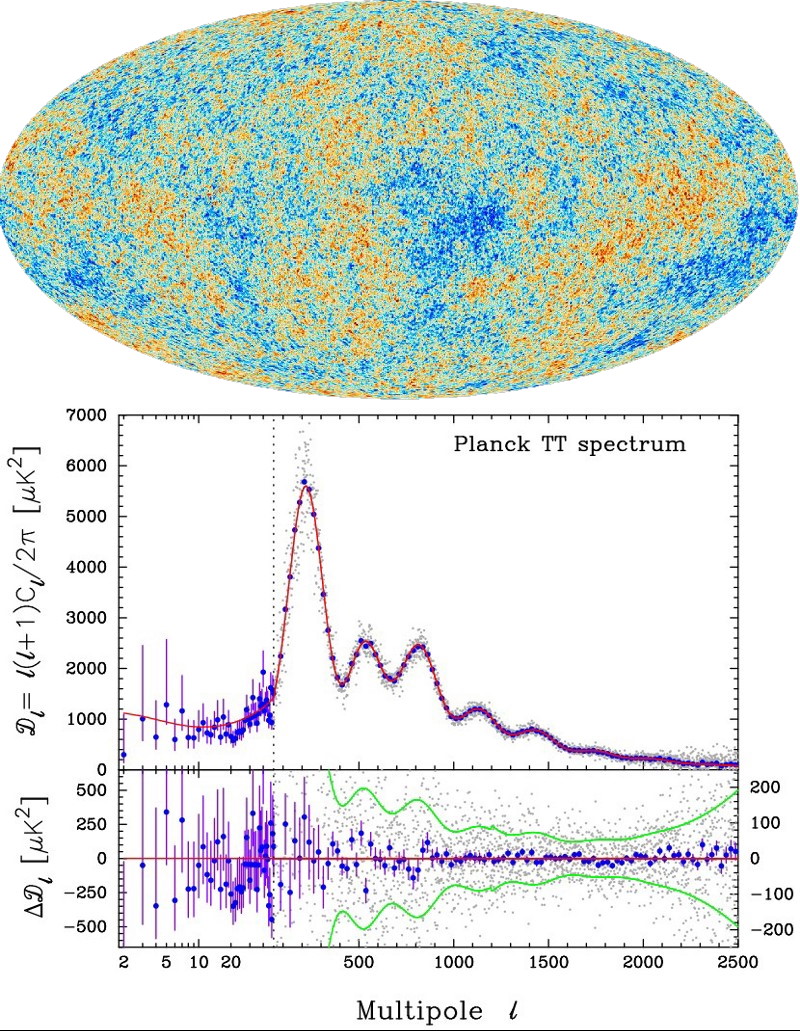
This consistency between extremely good data sets, including:
- the CMB,
- the large-scale structure of the Universe,
- and the cosmic distance ladder (including acceleration revealed by type Ia supernovae),
all points to a single unifying picture of our Universe. The hot Big Bang arose with a set of initial conditions predicted by cosmic inflation, and leads to a Universe made of:
- 68% dark energy,
- 27% dark matter,
- 4.9% normal matter,
- a tiny bit of neutrinos and radiation,
- with initial 1-part-in-30,000 fluctuations,
- and where the departure from scale-invariance (an n = 1 spectrum) is slight but robust (currently measured at n = 0.968),
with all observations pointing to precisely this picture, even despite the ongoing Hubble tension.
There is no known non-cosmic explanation for the CMB, including for its temperature, perfectly blackbody nature, dipole anisotropy, or the minuscule fluctuations seen on all scales. It’s true that we can’t “prove” that the Big Bang happened, but that’s not because of a lack of evidence; it’s because science can only ever give us our best current approximation of reality. But if we follow the evidence, there is no other viable conclusion, as all alternative approaches must ignore not just one but several of the aforementioned points. Despite the evergreen popularity of claims that “all mainstream scientists are wrong,” the purveyors of those claims are writing checks that the data simply invalidates as void.
Send in your Ask Ethan questions to startswithabang at gmail dot com!