What Is It Like As You Approach The Edge Of The Universe?
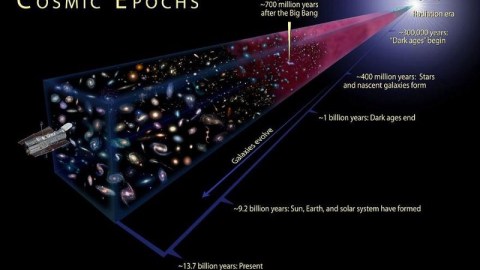
There’s a boundary to how far back we can observe everything that exists.
Despite everything we’ve learned about our Universe, there are many existential questions that remain unanswered. We don’t know if our Universe is finite or infinite in extent; we only know that its physical size must be greater than the portion we can observe. We don’t know whether our Universe encompasses all that exists, or whether it’s only one of many Universes making up a multiverse. And we remain ignorant about what happened in the earliest stages of all: in the first tiny fraction-of-a-second of the hot Big Bang, as we lack the necessary evidence to draw a robust conclusion.
But one thing we are certain about is that the Universe has an edge: not in space, but in time. Because the hot Big Bang occurred a known, finite time in the past — 13.8 billion years ago, with an uncertainty of less than 1% — there’s an “edge” to how far away we can see. Even at the speed of light, the ultimate cosmic speed limit, there’s a fundamental limit to how far back we can see. The farther away we look, the farther back in time we’re able to see. Here’s what we see as we approach the edge of the Universe.
Today, we see the Universe as it exists 13.8 billion years after the hot Big Bang. Most of the galaxies we see are clumped together in galactic groups (like the Local Group) and rich clusters (like the Virgo cluster), separated by enormous regions of mostly empty space known as cosmic voids. The galaxies within these groups are a mix of spirals and ellipticals, where a typical, Milky Way-like galaxy forms an average of about 1 new Sun-like star per year.
In addition, the normal matter in the Universe is mostly made of hydrogen and helium, but about 1-to-2% of the normal matter is made of heavier elements from the periodic table, enabling the formation of rocky planets like Earth and complex, even organic, chemistry. While there’s lots of variety — some galaxies are actively forming stars, some have active black holes, some haven’t formed any new stars for billions of years, etc. — the galaxies we see are large, evolved, and clumped together, on average.
But as we look farther and farther away, we start to see how the Universe grew up to become this way. As we look to greater distances, we find that the Universe is slightly less clumpy and slightly more uniform, particularly on larger scales. We see that galaxies are lower in mass and less evolved; there are more spirals and fewer elliptical galaxies. On average, there are greater proportions of bluer stars, and the star formation rate was higher in the past. There’s less space between galaxies, on average, but the overall masses of groups and clusters is smaller at earlier times.
It paints a picture of a Universe where today’s modern galaxies were created by smaller, lower-mass galaxies merging together over cosmic timescales, building themselves up to become the modern-day behemoths we see all around us. The Universe, at earlier times, consists of galaxies that are:
- physically smaller,
- lower in mass,
- closer together,
- larger in number,
- bluer in color,
- richer in gas,
- with higher rates of star formation,
- and with fewer proportions of heavier elements,
as compared to today’s galaxies.
But as we go farther and farther away — to earlier and earlier times — this gradually changing picture begins to transform abruptly. When we look back to a distance that’s presently 19 billion light-years away, corresponding to when only ~3 billion years had passed since the hot Big Bang, we see that the Universe’s star formation reached its maximum: about 20–30 times the rate at which new stars are formed today. An enormous fraction of supermassive black holes are active at this time, emitting enormous amounts of particles and radiation due to the consumption of surrounding matter.
For the past ~11 billion years or so, the Universe’s evolution has been slowing down. Sure, gravitation continues to collapse structures, but dark energy begins to work against it, coming to dominate the Universe’s expansion more than 6 billion years ago. New stars continue to form, but the peak of star formation is in our distant past. And supermassive black holes continue to grow, but shone at their brightest earlier on, with a greater fraction of them fainter and inactive today than during these early stages.
As we go to greater and greater distances, closer to the “edge” defined by the start of the hot Big Bang, we start to see even more significant changes. When we look back to distances of 19 billion light-years, that corresponds to a time when the Universe was just 3 billion years old, star formation was at its peak, and the Universe was maybe 0.3–0.5% heavy elements.
But as we close in on 27 billion light-years away, the Universe was only 1 billion years old. Star formation was much smaller, as new stars formed at rates approximately a quarter of what they’ll be, later on, at their peak. The percentage of the normal matter that’s made of heavy elements plummets precipitously: to 0.1% at an age of 1 billion years and to just 0.01% at an age of around 500 million years. Rocky planets, in these early environments, may well have been impossible.
Not only was the cosmic microwave background significantly hotter — it would have been at infrared rather than microwave wavelengths — but every galaxy in the Universe should be young and full of young stars; there are likely no elliptical galaxies this early on.
Going farther back than this really pushes the limits of our current instrumentation, but telescopes like Keck, Spitzer, and Hubble have already begun to take us there. Once we go back to distances of approximately 29 billion light-years or farther — corresponding to times where the Universe was 700–800 million years old — we start to run into the first “edge” of the Universe: the edge of transparency.
We take for granted, today, that space is transparent to visible light, but that’s only true because it isn’t full of light-blocking material, like dust or neutral gas. But at early times, before enough stars had formed, the Universe was full of neutral gas, and hadn’t become fully ionized by the ultraviolet radiation from these stars. As a result, a lot of the light we see is obscured by these neutral atoms, and it’s only once enough stars have formed that the Universe becomes fully reionized.
This is, in part, why infrared telescopes, such as NASA’s upcoming James Webb, are so crucial to investigating the early Universe: there’s an “edge” to where we can see in the wavelengths we’re familiar with.
At distances of 31 billion light-years, corresponding to a time of just 550 million years after the Big Bang, we reach the edge of what we call reionization: where the majority of the Universe is mostly transparent to optical light. Reionization is a gradual process and takes place unevenly; it’s like a jagged, porous wall in a lot of ways. Some places see this reionization happen earlier, which is how Hubble spotted its most distant galaxy to date (at 32 billion light-years away, just 407 million years after the Big Bang), but other regions remain partially neutral until nearly a billion years have passed.
Still, beyond the limits of our current instrumentation, stars and galaxies must certainly have existed. The most distant galaxies we’ve ever found still all show evidence that previous generations of stars have lived within them, and they’re already quite bright and massive. Beyond the limits of what our current telescopes can see, however, we can still measure the indirect signs that stars have formed: through the emission of light from hydrogen atoms themselves, which only occurs when stars form, ionization occurs, and then the free electrons recombine with the ionized nuclei, emitting light in the aftermath of that.
Right now, we only have the indirect signatures of this signature of early star-formation, teaching us that young galaxies existed as early as 180–260 million years after the Big Bang. These proto-galaxies formed enough stars that we can see the first hints of their existence buried in the data, corresponding to a distance of between 34 and 36 billion light-years away. Although our current telescopes cannot see these galaxies directly, the great expectation of many astronomers is that James Webb will.
However, there are likely still sources of light — and the first ionized regions of space in the Universe — going back even before that. The very first stars of all, if we could see back that far, are expected to come about between 38 and 40 billion light-years away, corresponding to times just 50-to-100 million years after the Big Bang.
Before that, the Universe was only dark, full of neutral atoms, and radiation from the Big Bang’s leftover glow.
Going back even farther, there are additional “edges” of interest. 44 billion light-years away, the radiation from the Big Bang was so hot that it becomes visible: if a human eye were to exist, it would be able to see that radiation begin to glow red, similar to a red-hot surface. This corresponds to a time just 3 million years after the Big Bang.
If we go back to 45.4 billion light-years away, we come to a time just 380,000 years after the Big Bang, where it becomes too hot to stably maintain neutral atoms. This is where the leftover glow from the Big Bang — the cosmic microwave background — originates from. If you’ve ever seen that famous picture of the hot (red) and cold (blue) spots from the Planck satellite (below), this is where that radiation originates.
And before that, 46 billion light-years away, we come to the earliest stages of all: the ultra-energetic state of the hot Big Bang, where the first atomic nuclei, protons and neutrons, and even the first stable forms of matter were created. At these stages, everything can only be described as cosmic “primordial soup,” where every particle and antiparticle in existence can be created from pure energy.
What lies beyond the frontier of this high-energy soup, however, remains a mystery. We have no direct evidence for what occurred in those earliest stages, although many of the predictions of cosmic inflation have been indirectly confirmed. The edge of the Universe, as it appears to us, is unique to our perspective; we can see back 13.8 billion years in time in all directions, a situation that depends on the spacetime location of the observer who’s looking at it.
The Universe has many edges: the edge of transparency, the edge of stars and galaxies, the edge of neutral atoms, and the edge of our cosmic horizon from the Big Bang itself. We can look as far away as our telescopes can take us, but there will always be a fundamental limit. Even if space itself is infinite, the amount of time that’s passed since the hot Big Bang is not. No matter how long we wait, there will always be an “edge” that we’ll never be able to see past.
Ethan Siegel is the author of Beyond the Galaxy and Treknology. You can pre-order his third book, currently in development: the Encyclopaedia Cosmologica.