What Happens When Planets, Stars, And Black Holes Collide?

Space may be enormous, but collisions are inevitable. Here’s what happens when they occur.
The Universe as we know it has been around for nearly 14 billion years: plenty of time for gravity to pull matter into clusters, clumps, and collapsed objects. By the present day, the Universe is filled with planets, stars, galaxies, and even larger structures, all bound together against the backdrop of the expanding Universe.
But things aren’t so clean and neat. As large as space is, there are literally trillions of objects in our galaxy, moving on timescales of billions of years. Some of the systems that form will have multiple objects in them, and collisions between them aren’t just likely, they’re inevitable. Whenever a collision or merger occurs, it forever changes what we’re left with. Here’s the cosmic story of what happens.
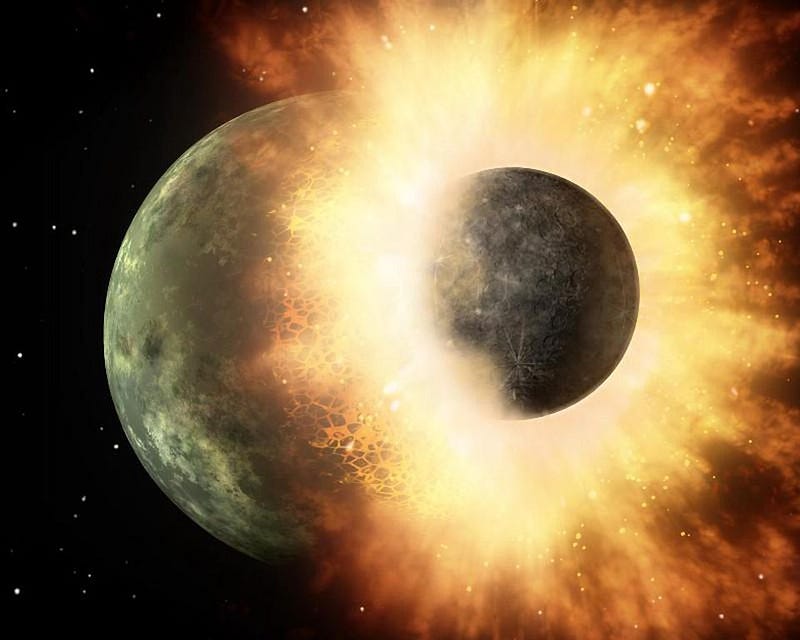
Planet-planet collisions. Early on in the Solar System, there were likely more than eight planets. There may have been a fifth gas giant out between Jupiter and Neptune; our best simulations indicate that it got ejected. But in the inner Solar System, we believe there was a Mars-sized world that collided with a young Earth, giving rise to an enormous cloud of debris that coalesced to create our Moon. The giant impact hypothesis has been thoroughly validated by a number of lines of evidence, including by the lunar samples we brought back to Earth from the Apollo missions.
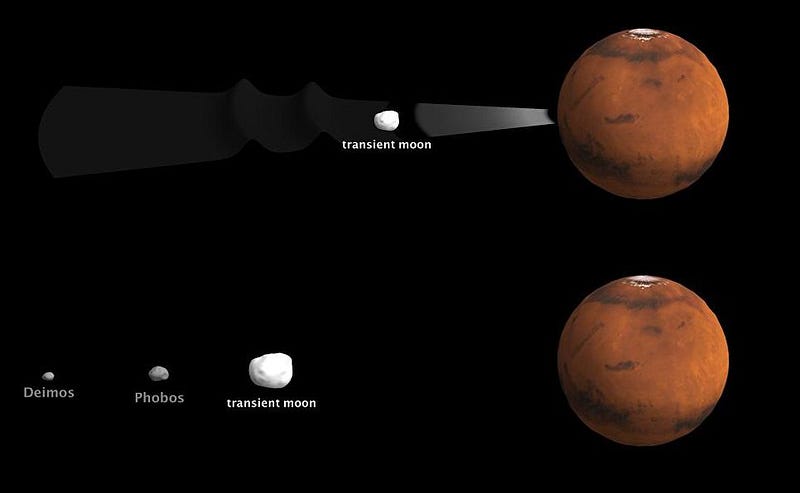
Beyond that, we also have some pretty good evidence that Mars’ moons were created, along with a third, larger one that’s since fallen back down onto the red planet, by a large proto-planetary collision, too.
From all the simulations we’ve performed and the evidence we’ve accumulated, rocky planets of comparable sizes collide quite frequently in the early stages of a solar system’s creation. When they smash together, they create a single, larger planet, but with a cloud of debris that coalesces to form one nearby, large satellite and up to several smaller, more distant satellites. The Pluto-Charon system is a spectacular example of this, with four additional, outer, tumbling moons.
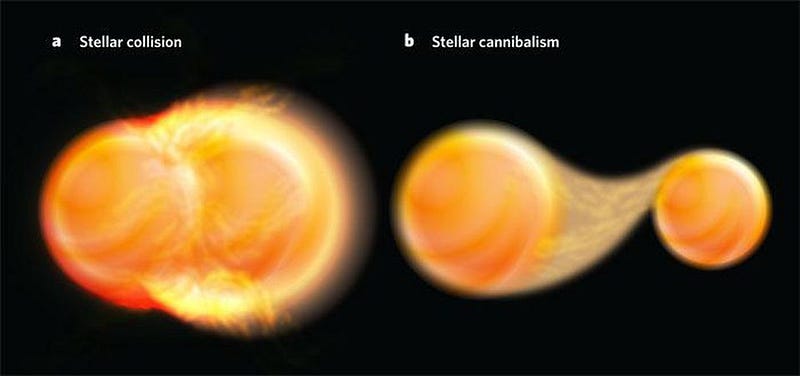
Brown dwarf collisions. Want to make a star, but you didn’t accumulate enough mass to get there when the gas cloud that created you first collapsed? There’s a second chance available to you! Brown dwarfs are like very massive gas giants, more than a dozen times as massive as Jupiter, that experience strong enough temperatures (about 1,000,000 K) and pressures at their centers to ignite deuterium fusion, but not hydrogen fusion. They produce their own light, they remain relatively cool, and they aren’t quite true stars. Ranging in mass from about 1% to 7.5% of the Sun’s mass, they are the failed stars of the Universe.
But if you have two in a binary system, or two in disparate systems that collide by chance, all of that can change in a flash.
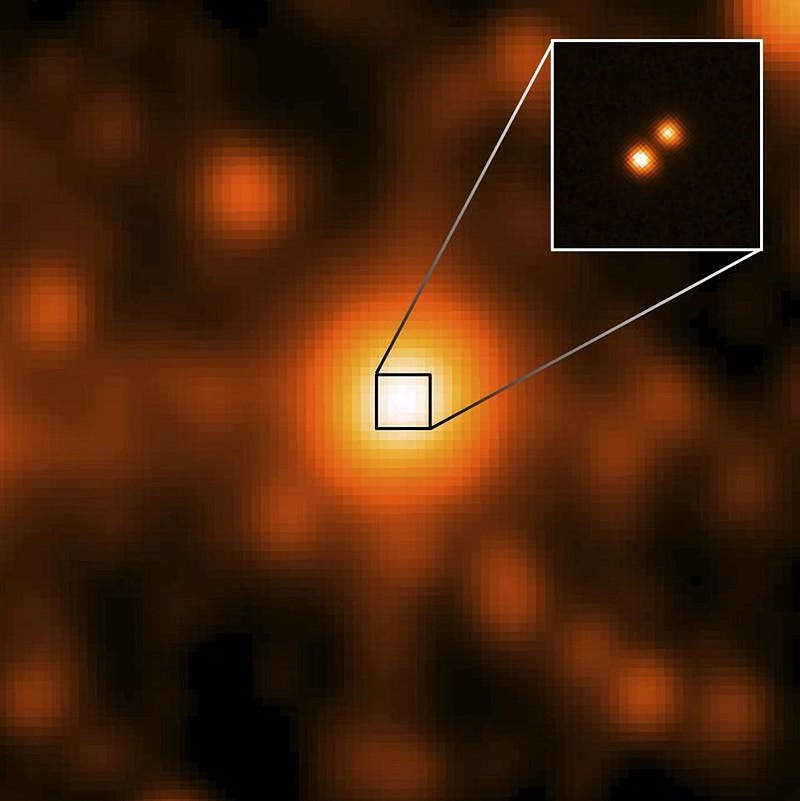
The reason for that is that very little about the compositions of these failed stars changes over time. They’re still made of 70–75% hydrogen each, and when they merge together, they still have all of that unburned fuel. If the total mass of the merged object now exceeds that critical threshold of 0.075 solar masses, the Universe will have created a new star! With this much mass in a single object, temperatures will rise past that critical 4,000,000 K to ignite hydrogen fusion. Instead of two brown dwarfs, we’ll have created a red dwarf: a bona fide M-class star. The nearby binary brown dwarf system Luhman 16, just 6.5 light years away, is tantalizingly close to having the exact parameters necessary to eventually become a red dwarf star.
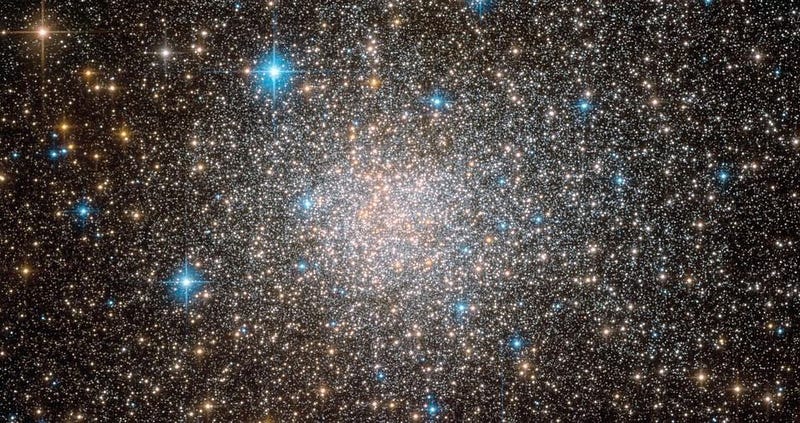
Two stars colliding. Stars come in a wide variety of masses, with the lower-mass ones appearing redder, cooler, and burning through their fuel more slowly, while the higher-mass ones are bluer, hotter, and live for shorter amounts of time. When we look at star clusters, we can get an idea of how old they are by viewing the highest-mass stars that are left, since the most massive ones die the fastest.
Yet when we look at some of the oldest star clusters of all, we find a population of stars that are bluer and hotter than ought to be present. They simply don’t match up with the rest of the stars that are around. These blue straggler stars are real, though, and they have a fantastic explanation: stellar collisions.
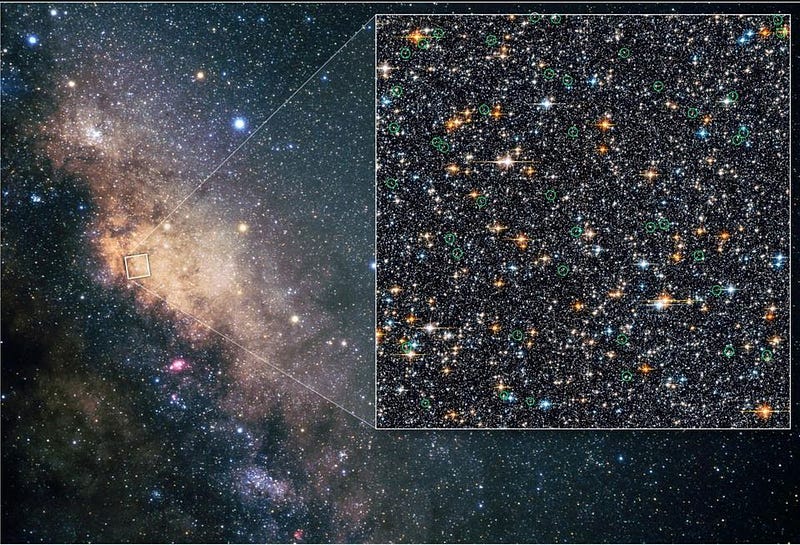
Take any two (or more) stars and merge them together, and they’ll make a single, more massive star. Even when all that remain are the redder stars, say one of 0.7 solar masses and one of 0.8 solar masses, if they merge together, they can create a bluer (1.5 solar mass) star, even if the star cluster they exist in is too old to have a 1.5 solar mass star remaining.
Blue stragglers are common in the dense environments of globular clusters, and demonstrate that even long after all the stars as massive as the Sun have burned out, we will still create new ones simply by gravitational mergers.
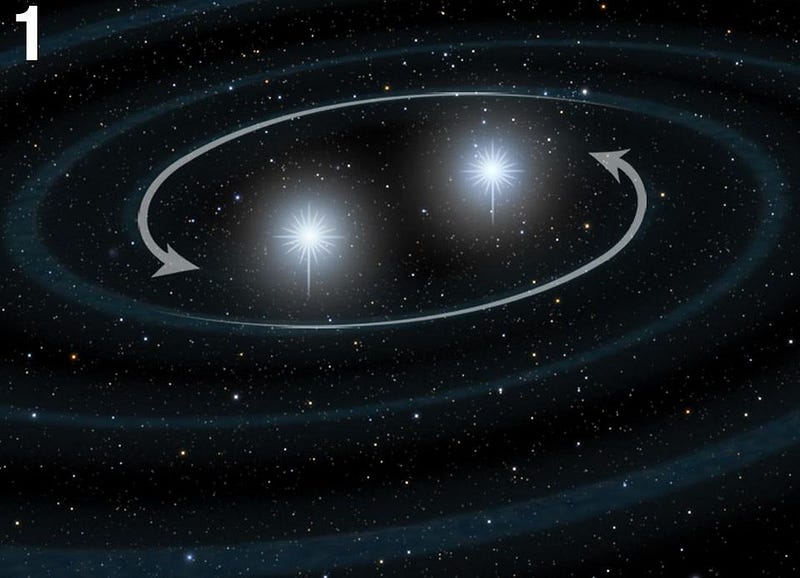
White dwarf collisions. So, your normal, main-sequence star lived through its life, burning through all the fuel it will ever burn. As a remnant, its core became a white dwarf star: the future fate of our Sun. And then, floating out there in the depths of interstellar space, it collided with another white dwarf star.
BOOM!
White dwarf-white dwarf collisions lead to Type Ia supernovae, and may yet be the most common way these cataclysms originate. When such an event occurs, the stars undergo a runaway fusion reaction, giving off a tremendous amount of light and energy, and utterly destroy both white dwarfs that gave rise to the event. This is the one type of collision that completely destroys both the colliding objects.
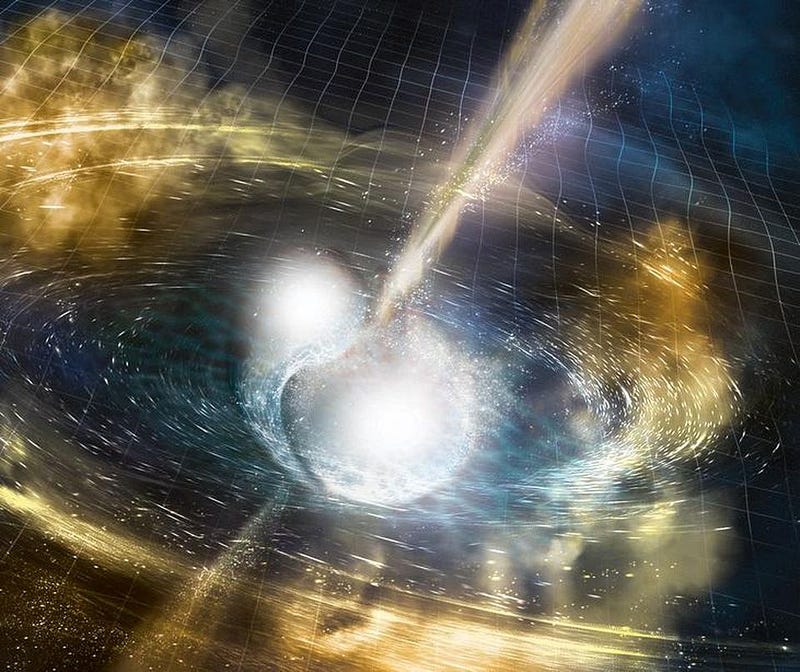
Neutron star collisions. Arising from even more massive stars than those that give rise to white dwarfs, neutron stars can often exist in multi-star systems. Recently, we’ve observed two neutron stars in a binary system inspiraling and merging: a kilonova event. When this occurs, a large burst of energy is given off, and a substantial fraction of mass is ejected. The critical 2017 event that occurred marked the first time that the same object was observed in both gravitational waves and electromagnetic radiation.
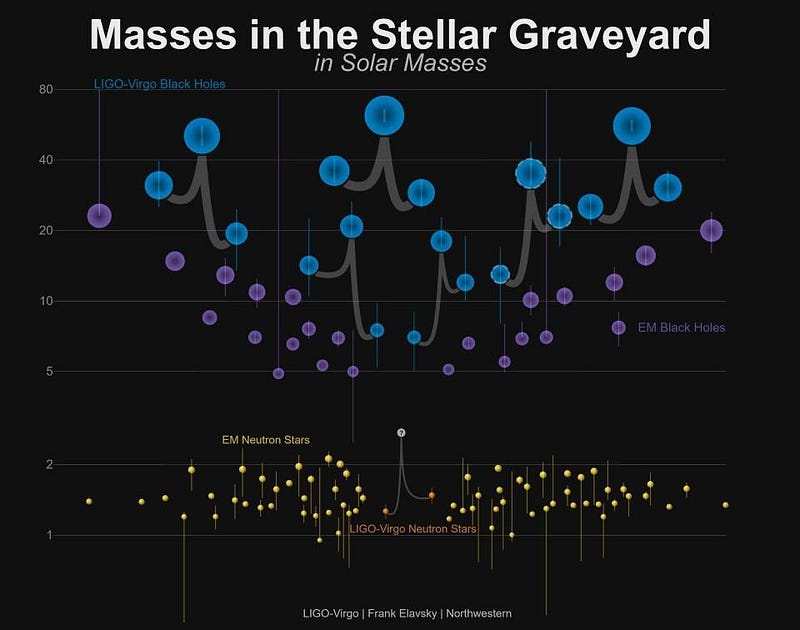
If the two neutron stars merge together to create a single one, they either:
- become a more massive neutron star (if their total is less than ~2.5 solar masses),
- become a neutron star that spins and then collapses to a black hole (if the total is under 2.75 solar masses),
- or collapses directly to a black hole (if the total mass is over 2.75 solar masses).
Over the coming years and decades, we hope to observe many of these events to refine the accuracy of these statements even further.
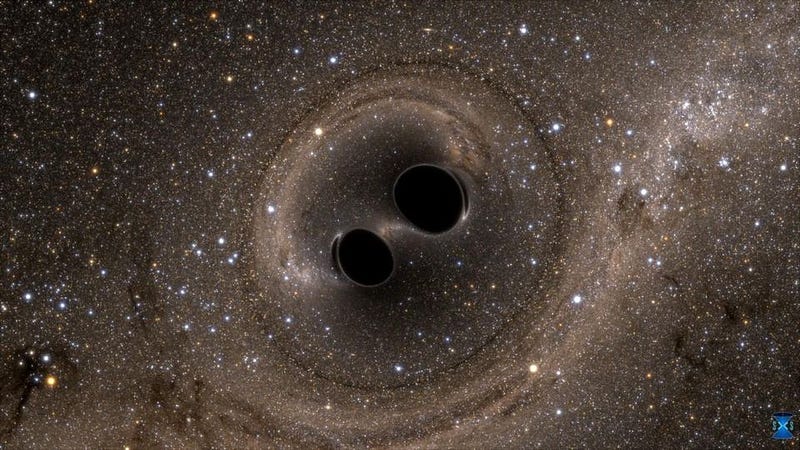
Black hole collisions. Merge a black hole with a black hole, and you get an even more massive black hole. But there’s a catch: up to around 5% of that mass gets lost! The first merging black hole pair we ever saw was a 36 solar mass black hole merging with a 29 solar mass black hole. But it created a black hole whose final mass was just 62 solar masses! A total of three suns worth of mass was simply lost.
Where did it go? It was emitted in the form of gravitational radiation: the gravitational waves that LIGO detected from over a billion light years away. For a brief moment lasting less than a second, two merging black holes can emit more energy into the observable Universe than all the stars within it combined.
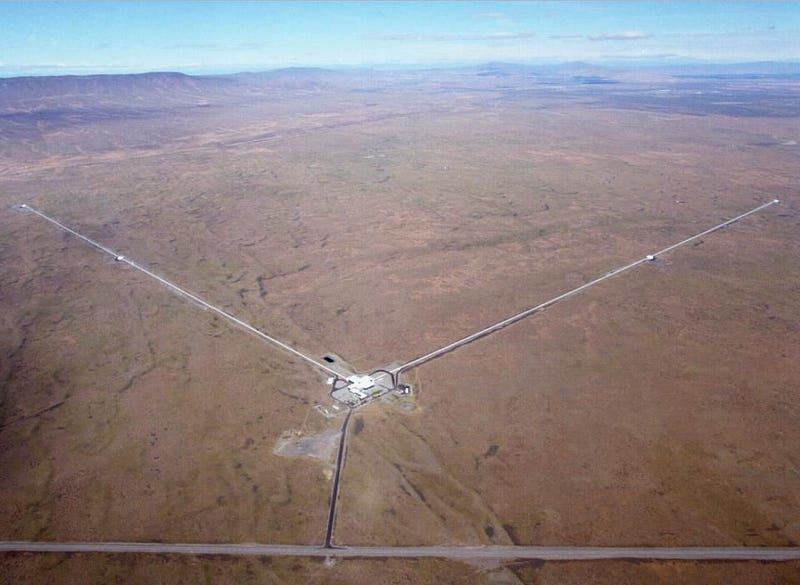
Other collisions are expected, such as black hole-neutron star, neutron star-white dwarf, neutron star-normal star, or even black hole-normal star. Objects like active galaxies or microquasars may be triggered by a black hole devouring stars or gas clouds. We have yet to observe any of these collisions as they happen, however, although we have discovered a candidate for a Thorne-Zytkow object: a neutron star at the core of a red giant star. Space may be a very big place, but it’s far from empty. Particularly within galaxies and star/globular clusters, the density of planets, stars, and stellar remnants are tremendous, and collisions such as these are inevitable. Whatever the consequences may be, it’s up to us to find out!
Ethan Siegel is the author of Beyond the Galaxy and Treknology. You can pre-order his third book, currently in development: the Encyclopaedia Cosmologica.