Ask Ethan: Why is the Universe electrically neutral?
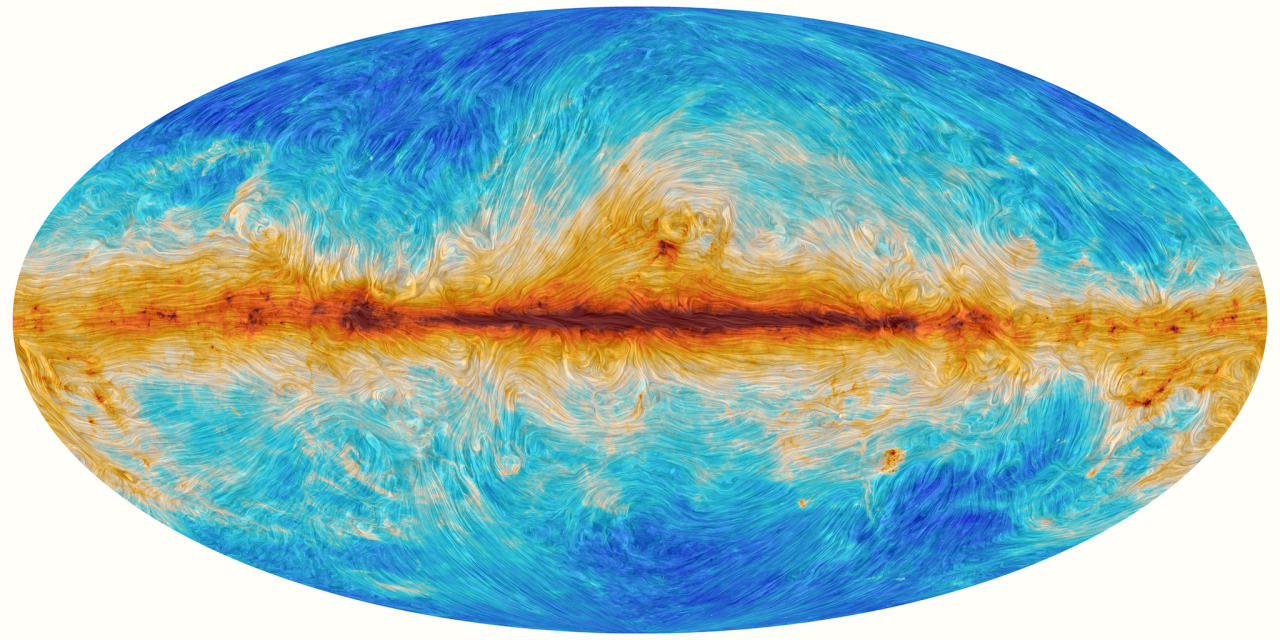
- On all cosmic scales in the Universe, from planets to the cosmic web, it’s the gravitational force that determines the structures we get, not the electromagnetic or nuclear forces.
- The nuclear forces are only short-range, but electromagnetism stretches just as far as gravitation does, and is ridiculously more powerful.
- This implies the electron and proton have not only equal and opposite charges, but equal and opposite numbers in the Universe. But why is this so?
Everywhere we look, on each and every one of the largest cosmic scales, gravitation dominates the structure that we form. From planets to stars, stellar systems, star clusters, galaxies, and clusters of galaxies on up, it’s the gravitational force that overwhelmingly and single-handedly determines what sort of entities we wind up with. But this itself has some profound implications, because the electromagnetic force between, say, two protons, is something like ~1036 times stronger than the gravitational one. It would only take a very, very slight charge imbalance for the electromagnetic force to dominate over the gravitational one.
And yet, especially on large cosmic scales, it never ever appears to do so. Does that mean the Universe, overall, is electrically neutral? And if so, why is that? That’s what Ruppert Koch wants to know, asking:
“It appears that each part of the universe is electrically neutral. But why is that? Why does the number of electrons in the universe match the number of protons? Even if one would assume that all protons and electrons came from decaying neutrons, it still leaves the question why there were exactly twice as many down quarks created than up quarks.”
It’s a great question, and the answer goes even deeper than you’d probably imagine. Let’s take a closer look.

First off, if we boil it down to subatomic particles, there are only two major species of particle that contribute to the overall electric charge of the Universe: the proton, which is positively charged and made up of charged quarks, and the electron, which is negatively charged and is presently known to be fundamental. Our observable Universe, as we know it, began some 13.8 billion years ago with the hot Big Bang, and has expanded to occupy a size that’s approximately 92 billion light-years in diameter.
Because we know what makes up the Universe — i.e., what our ratios are of dark energy to dark matter to normal matter to neutrinos to photons — and we’ve measured the density of what’s in it quite well, we can calculate the total number of particles of each type that must be present. All told, there are somewhere around 1080 protons present throughout the Universe, including bound up in atomic nuclei, with an exactly equal number of electrons.
If the numbers of each are exactly equal, and the charges are exactly equal and opposite, then the Universe, overall, is perfectly electrically neutral.
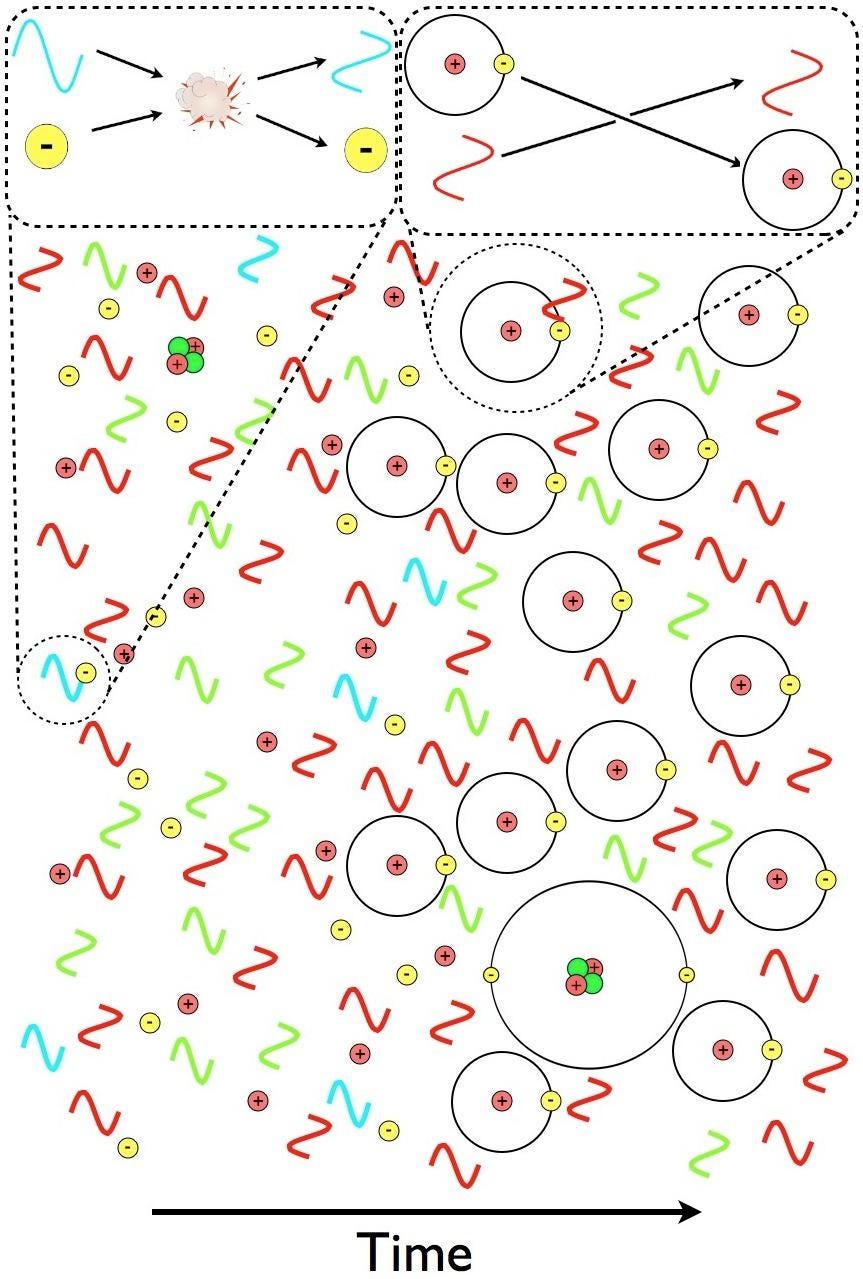
But does this have to be the case? Theoretically, there are two possible ways to have a Universe that does, overall, actually possess a net charge.
- The total positive charge possessed by the proton could be very, very slightly different in magnitude from the negative charge possessed by the electron.
- The number of protons, overall, could be very, very slightly different from the number of electrons that exist in the Universe.
If either one of these things are true (or if both of them are true), then the Universe could possess a net electric charge, and wouldn’t be electrically neutral after all.
Is this possible? In theory, yes. We know that there are two types of symmetries that have enormous consequences for the physical Universe: global symmetries, which do things like conserve baryon number and lepton number, and gauge symmetries, which do things like conserve color charge or electric charge. The fact that our Universe is made of matter and not antimatter, i.e., baryons and leptons instead of anti-baryons and anti-leptons, indicates that, at some level, those global symmetries must have been violated at some point in the past. Similarly, there may have been an epoch in the past where the electromagnetic gauge symmetry was broken, allowing for a (temporary) violation of electric charge conservation, as in grand unification or higher-dimensional theories. It’s also possible that the electromagnetic gauge symmetry isn’t exact, which arises if you allow the speed of light to be variable, if you vary the fine-structure constant with time, if you add massive photons into the Standard Model, and other tweaks.
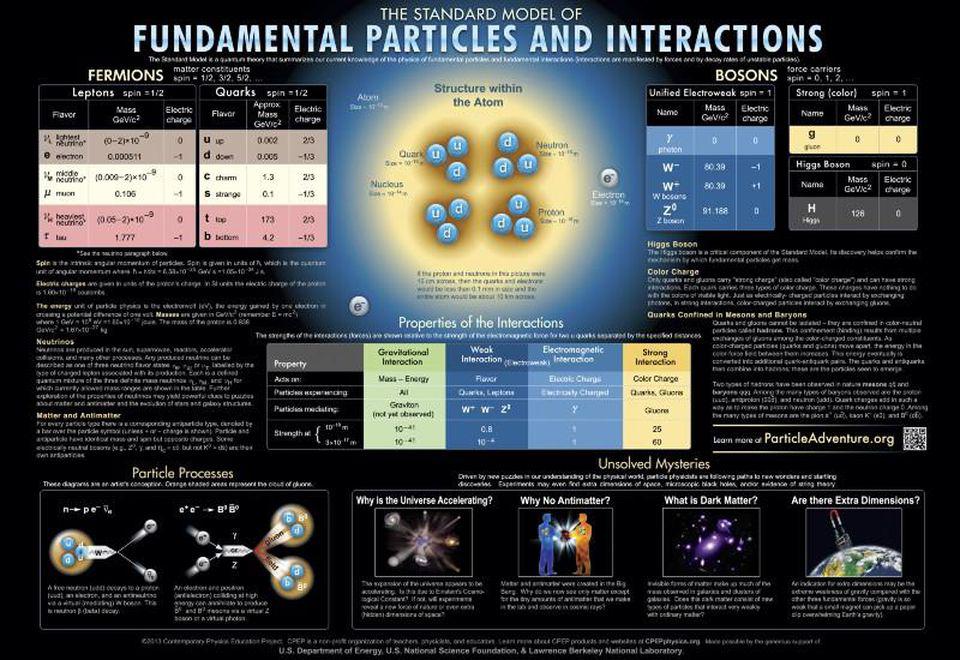
But whatever we want to admit theoretically, we have to confront it with the observable Universe that we actually have. We know that it’s possible to have places where charge conservation is violated on small cosmic scales simply by having the flow of current: allowing charge to move into or out of a region of space. What we can do, therefore, is to look at how equal certain signals that are charge-dependent are in different directions in space: at cosmic anisotropies.
Observationally, we find the following.
- Cosmic rays are incredibly isotropic, and originate from the late-time, mostly present-day Universe. They tell us that if there is a charge asymmetry today, it’s smaller than one electron for every 1029 electrons-and-protons that exist.
- The cosmic microwave background is also incredibly isotropic, but it doesn’t originate from today. Instead, the leftover light we see from back then was last emitted from a hot electron-and-nuclei-based plasma from a redshift of 1089, or an age of the Universe of ~380,000 years. If there was a charge asymmetry back then, it too, was smaller than one electron for every 1029 electrons-and-protons that existed back then.
- And finally, we can look at the abundances of the light elements, which arose extremely early on due to the physics of big bang nucleosynthesis. This occurred even earlier in the Universe’s history: during the first few minutes, or approximately a redshift of 400 million. A charge asymmetry in that early epoch is constrained even more tightly: to be no greater than one electron for every 1032 electrons-and-protons back at that time.
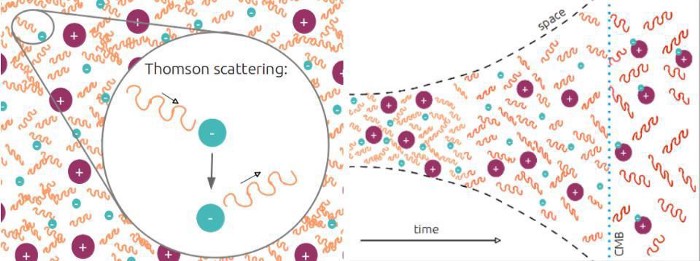
In other words, we have evidence from modern times, from the formation of neutral atoms, and from the formation of atomic nuclei that the Universe is at least almost perfectly electrically neutral, and was at all three epochs. (And hence, probably at all epochs in between.)
But there’s nothing that mandates that the Universe was electrically neutral on all scales at all times. It’s possible that the Universe was born with more protons than electrons or vice versa, even if the excess of one type over another was small. In other words, we presently don’t have constraints on what happened at even earlier times; perhaps the Universe is only electrically neutral now?
Although there isn’t a good reason to think it might have been electrically charged overall, what we can do, theoretically, is to assume that the Universe had, at some early time, either a local (confined to one location) or global (occurring everywhere, on all cosmic scales) charge asymmetry, with either more positive or negative charges present, and apply the laws of physics to see what happens.

Normally, when we think about the expanding Universe, it’s a useful approximation to visualize a small sphere that’s both full of matter (and antimatter, if you like) and radiation, where it’s expanding rapidly and it’s also gravitating. Conceptually, you can think about it as a race, where the initial expansion works to dilute the density and drive everything apart, while the gravitation of all the different forms of matter and energy work to pull things back together.
Regardless of who wins, you can always expect that the expansion will slow down, gravitation will attempt to recollapse the Universe, and in the meantime, the overdense regions will grow denser and denser into massive clumps, while the underdense regions will give up their matter and energy to the overdense regions, becoming voids themselves.
This is the standard picture of the expanding Universe, but it only works if gravitation is the only force that matters, and if the original overdense and underdense imperfections are sufficiently small in the context of the expanding Universe.

Now, let’s change the equation, and add in either a greater number of positive or negative charges. How does the story change?
Let’s assume, for simplicity, we have greater amounts of positive charges. What happens now?
- If you’re electrically neutral, you don’t feel the electric force, so nothing different happens; you simply dilute in terms of density as the Universe expands, and you clump together, gravitationally, in accordance with whatever overdensities and underdensities are present.
- If you’re negatively charged, however, you’ll see that there’s a greater density of positive charge all around you, so you’ll experience an additional attractive force that works in the same direction as gravitation. The positive charges will effectively “pull you in,” causing your density to decrease more slowly than the neutral matter, and so the negative charges will struggle to expand as rapidly as the neutral quanta do.
- Similarly, the positive charges will experience a greater density of positive charges all around them, and hence they’ll experience an additional repulsive force, opposed to gravity. This makes the positive charges get effectively “pushed out,” causing them to get less dense more rapidly than the other species of particles, effectively thinning their density out.
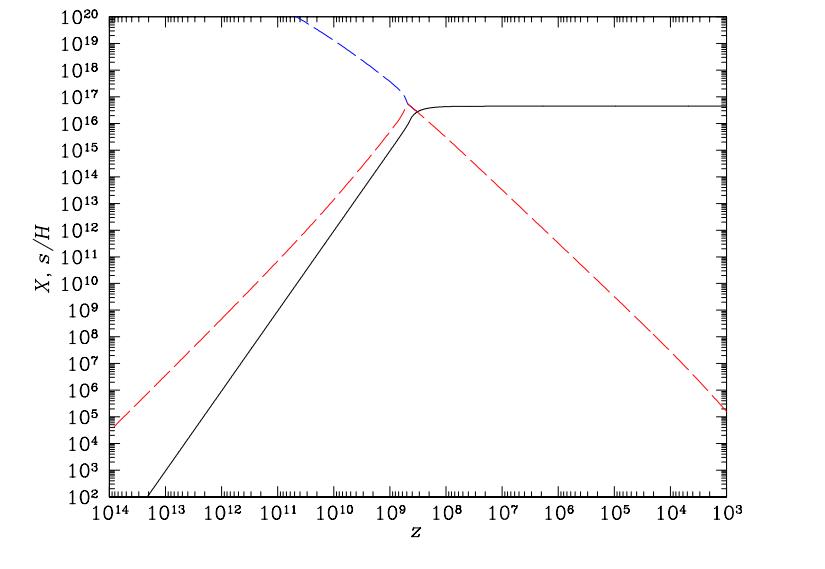
How quickly does this happen? In other words, if the Universe is either born with or acquires a charge imbalance, how long can it persist versus how much time does it take to get wiped out?
A 2007 study showed that, for electric charges, it all depends on what the damping rate is compared to the expansion rate. If you have a charge imbalance early on, it gets damped by a factor of about 100,000 when the Universe is only about a nanosecond old, and that damping factor increases all the way up to a factor of around a few quadrillion when the Universe is around a minute old. Finally, it drops, but remains above the ~100,000 mark until the Universe is hundreds of thousands of years old: at the time the cosmic microwave background is emitted.
In other words, an electric charge imbalance cannot survive in the early Universe; it gets wiped out, as the “greater” density species gets diluted and expands more quickly, while the “lesser” density species gets diluted more slowly, allowing the densities to equilibrate.
Electric currents fare even worse, getting wiped out more strongly at early times (before nucleosynthesis) and then with the same strength (after nucleosynthesis) as charge asymmetries.
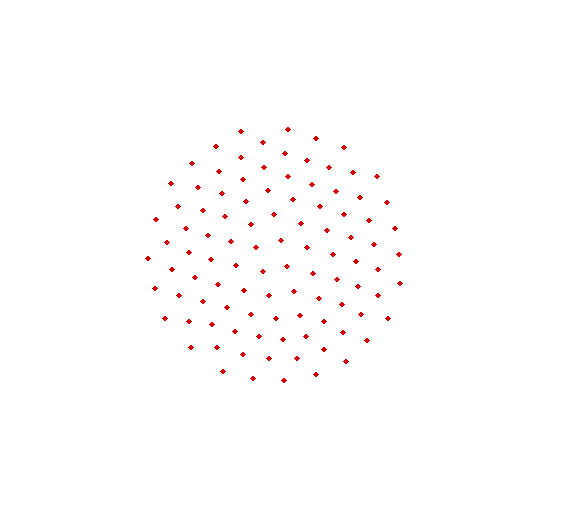
Does this mean the Universe is driven to a state of electrical neutrality regardless of how it starts off, and then it stays neutral, everywhere and at all times?
Fascinatingly enough, the answer appears to be yes to the first part, but no to the second part. The reason is a little more complex, but was worked out the year prior: in 2006. Basically, even when you drive the Universe toward a neutral state on all scales, electrons and atomic nuclei experience slightly different effects as they gravitate. Yes; they both expand with the expanding Universe. Yes; they both gravitate, and feel equal gravitational forces. Yes; they even both experience equal-and-opposite electromagnetic forces, and that experience balances out.
But they have different masses from one another, and therefore they have different charge-to-mass ratios. A proton’s charge-to-mass ratio is 1836 times smaller than an electron’s, and the charge-to-mass ratio of a helium nucleus is some 3646 times smaller than an electron’s. Back before the formation of neutral atoms, therefore, the radiation pressure that’s present, due to photons, “pushes out” on the electrons more efficiently than it pushes out on protons and nuclei. This creates a small charge separation, which leads to plasma oscillations, an electric current, and both a small charge imbalance and a small magnetic field. On cosmic scales, it’s small, but it’s not negligible.
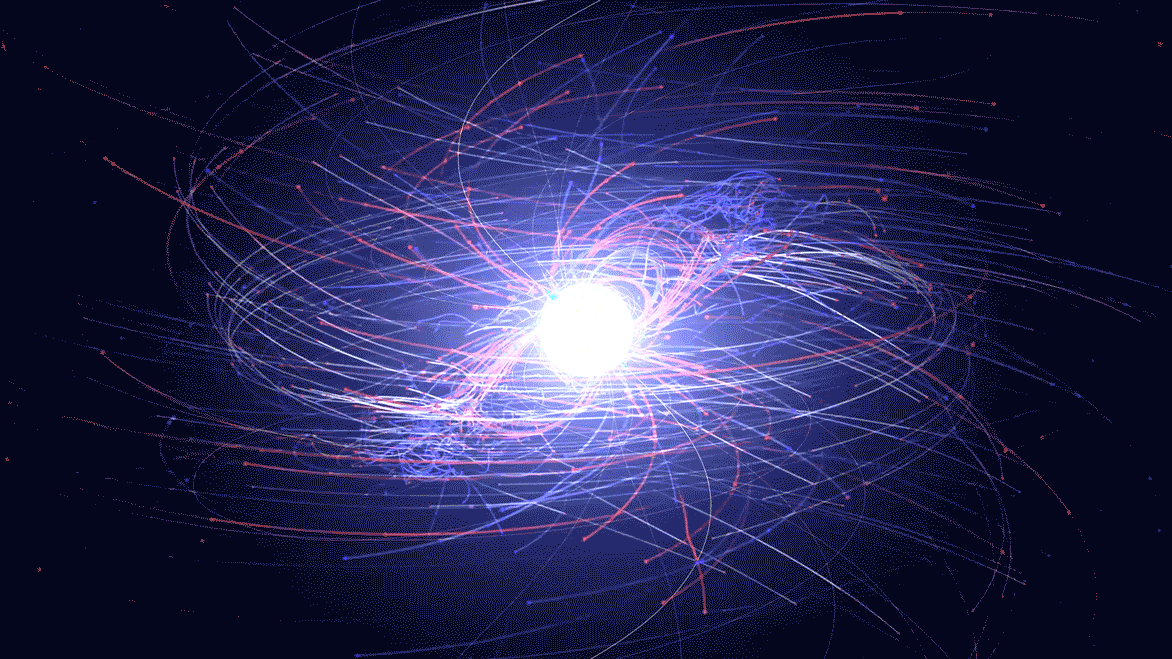
Overall, you’d expect that a small charge imbalance, on the order of about 1 part in 1034 on what will expand into galaxy-like scales, gets created. It also means that tiny magnetic fields, on the order of 10-24 gauss, get imprinted into the seeds of what will eventually grow into galaxies. This tiny excess of electric charges won’t persist; the “excess” charges get pushed to the outskirts, and will become part of the plasma that populates the space between the galaxies: the warm-hot intergalactic medium. It exists only on local scales, and is expected to get less severe on larger cosmic scales, down to about 1 part in 1046 on the scale of the modern-day cosmic horizon. This is well below the limit of detectability, but should occur whether you begin with a perfectly charge-symmetric Universe or not.
But the fact that tiny-magnitude magnetic fields get created on galactic (and larger) scales at very early times, whether you start with a charge imbalance or not, is profound. We see magnetic fields permeating pretty much every galaxy today, but don’t know where they come from. Stars generate magnetic fields, but galactic-scale magnetic fields are coherent on the scales of hundreds or even thousands of light-years, much larger than the scales of individual stars. Hot, ionized gases and plasmas collide in the interstellar medium, producing magnetic fields as well, possibly even before stars form. But at even earlier times, this charge asymmetry and current-generating mechanism takes place, and is a third candidate for creating magnetic fields on galactic scales (and greater) in the Universe.
Overall, we know things are electrically neutral to a high degree. As is often the case, however, working out the full details of a problem such as this can reveal effects one might never have imagined. I’m not one to tell physicists to shut up, but there’s never an excuse to not calculate!
Send in your Ask Ethan questions to startswithabang at gmail dot com!