Throwback Thursday: How Fast Do Stars Run Out Of Fuel?
At some point, a star’s core runs out of fuel. Then what?
“Man loves company — even if it is only that of a small burning candle.”
–Georg C. Lichtenberg
You normally think of stellar evolution, of stars burning, as an instantaneous thing. One moment, you’ve got a star like our Sun, burning hydrogen into helium, then later you’ve got a giant star burning even heavier elements, and finally you get the catastrophic end-of-life throes of the star, as it expels its outer layers and has its inner core either contract or collapse down depending on what type of star it is.
Either way, you have different phases and not a whole lot of (what appears to be) transitioning between them.
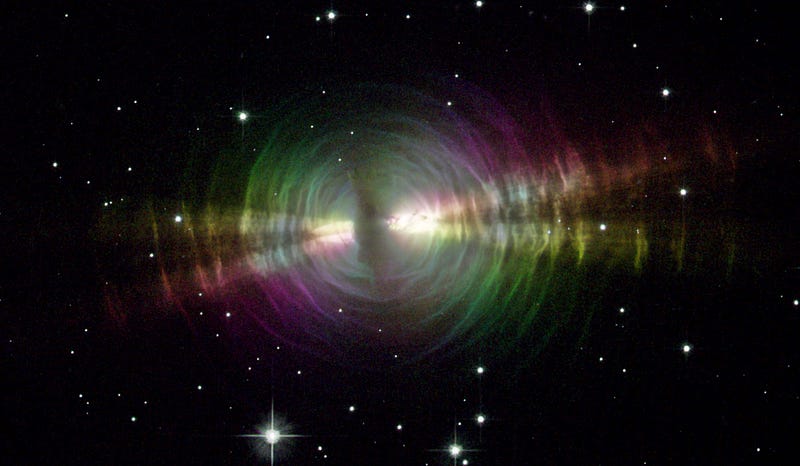
If we want to understand both what happens and how it happens, we might as well tell the entire story of a star’s life, from the beginning of its burning all the way through to the end.
To start, all we need is a newborn star. Only, those don’t happen by themselves: the only place to find them is in giant clusters!
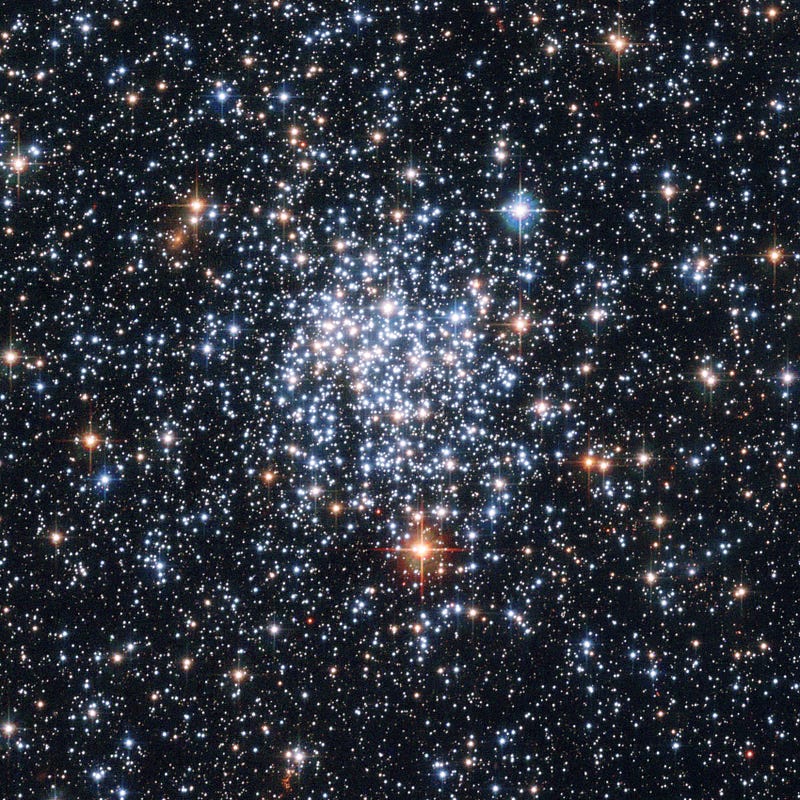
In a young star cluster (like NGC 265, above), you have stars of all different masses in there, ranging from the most massive, hottest O-and-B class stars that are tens (or even hundreds) of times as massive as our Sun, all the way down to the lowest mass, reddest and dimmest M-class stars. (There are many times more “failed stars” in there as well, but that’s a story for another time.)
What gives these stars the colors and brightnesses that they possess?
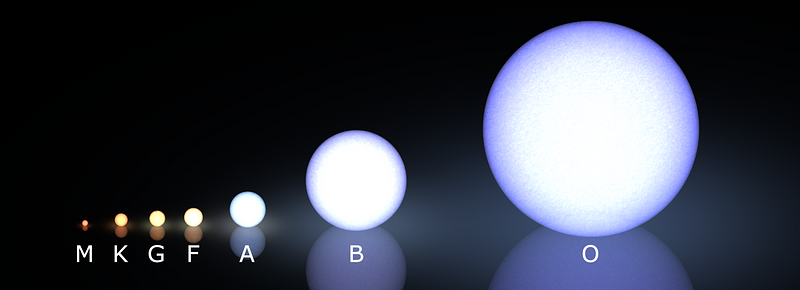
The flippant answer would be “their mass,” but the truth is a little more nuanced, and a little more enlightening.
You see, the reason these stars are shining at all is because there’s nuclear fusion taking place in their cores. After huge amounts of mass — the equivalent of some 25,000 Earths in even the lowest-mass M-class star — contract together into protostars, heating up tremendously, the densities and temperatures in the cores become sufficient to ignite a self-sustaining nuclear fusion reaction.
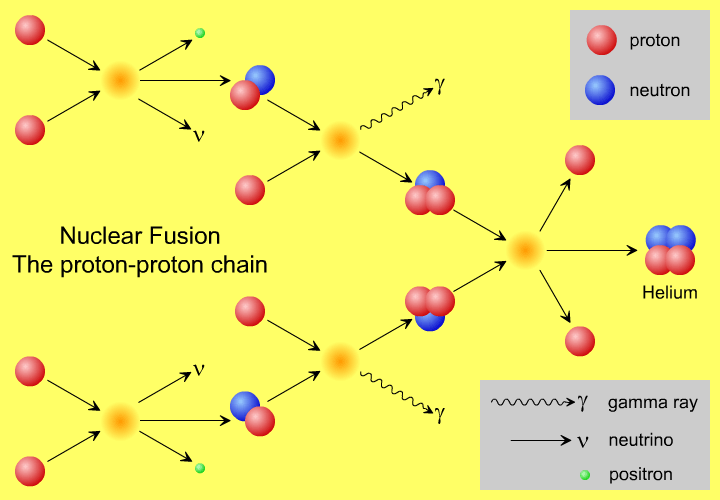
The main differences between what makes a star so blue and bright, as opposed to red and dim, has everything to do with the temperature inside! Inside the Sun, for example, the temperature at the very core is around 15 million Kelvin, and nuclear fusion happens somewhat quickly there.
But as we move farther out, the temperature drops, but the rate of fusion drops exponentially with temperature! By time we’re 25% of the way out of the Sun, the temperature has dropped by less than a factor of two, and yet the rate of fusion is less than 1% of what it is at the core!
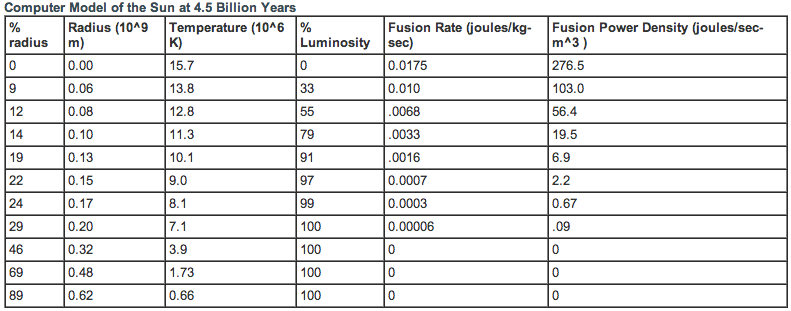
This is why a star whose temperature might be half that of the Sun can live hundreds of times as long, and an incredibly hot star — like R136a1 (at the core of the cluster below), with 260 times the mass of the Sun — will live less than 0.1% as long as our Sun.
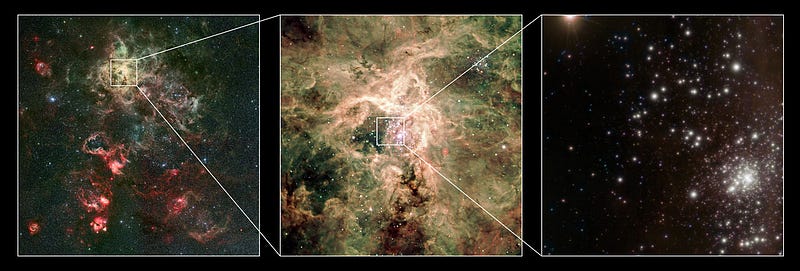
Now, that’s the difference between stars when they’re first born. But as they live and burn through their fuel, the regions with spent fuel in them starts to collapse. There are a number of different ways to change an objects volume; the collapse happens adiabatically, which means the entropy remains constant but the temperature rises inside the star! And that means both that a largerregion around the core can fuse whatever fuel is burning at the time, and also that the rate of fusion goes up.
In addition to everything else that happens, this means the temperature and luminosity of the stars are all expected to gradually rise as they age.
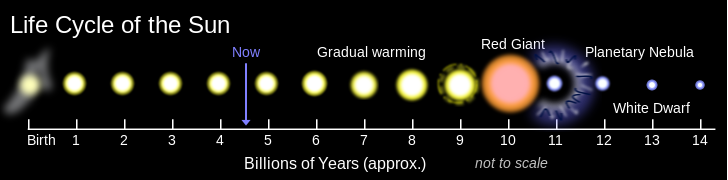
What happens over time is that there’s a certain amount of radiation pressure that needs to come out of all layers of the star in order to hold it up against gravitational collapse. The Sun is a constant radius because the outward radiation pressure at the surface is (roughly) the same as the inward gravitational pull. But when the core of a star — and this is true of any layer in the star — runs out of the fuel it’s burning, the radiation pressure drops precipitously, and starts to lose against the pull of gravity.
There are two options here: either the core can contract and heat up sufficiently to ignite more fusion — whether hydrogen, helium, or in the case of the most massive stars, carbon fusion and beyond — or it can remain inert, because it can’t heat up enough to burn the next stage of fuel, in which case the end of the star is near.
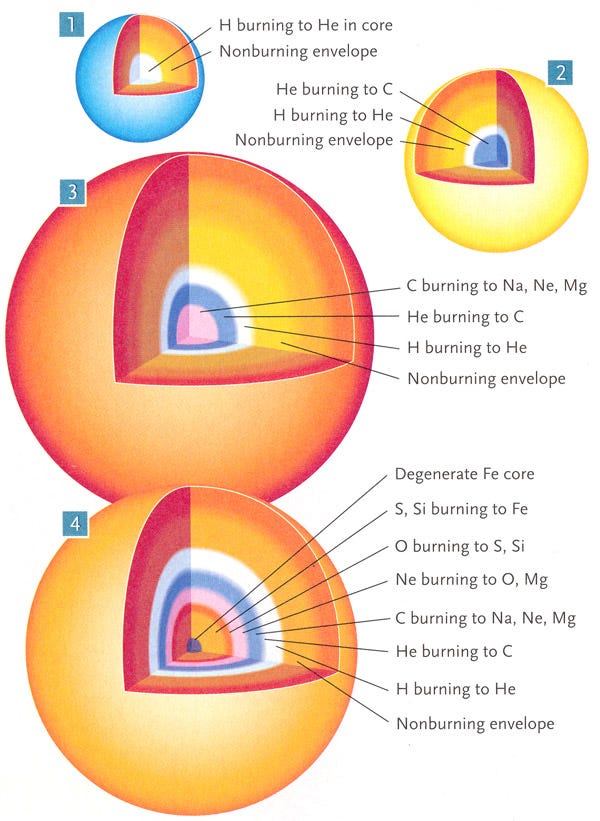
The helium core takes a long time to form — millions of years in even the most massive stars — and helium burning takes maybe 10% of the time that hydrogen burning does. In stars that achieve carbon burning, the timescales from the first fusion of carbon in the core until the iron inner core causes a supernova is on the order of a thousand years, and no more. That part of the story is fast!
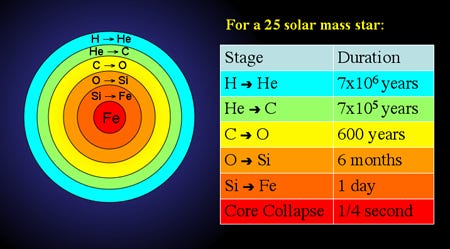
In reality, once the inner core of a Sun-like star runs out of hydrogen fuel in its central region — something that will happen to our own Sun in 5-to-7 billion years — it will expand into a subgiant star first, where it will remain for hundreds of millions of years before igniting helium and becoming a red giant. But that first step of becoming a subgiant is when it will leave the main sequence for good.
After that, all the other steps are relatively rapid, as the “main sequence” is called that for a reason: it’s where all stars spend the main amount of their lifetimes.
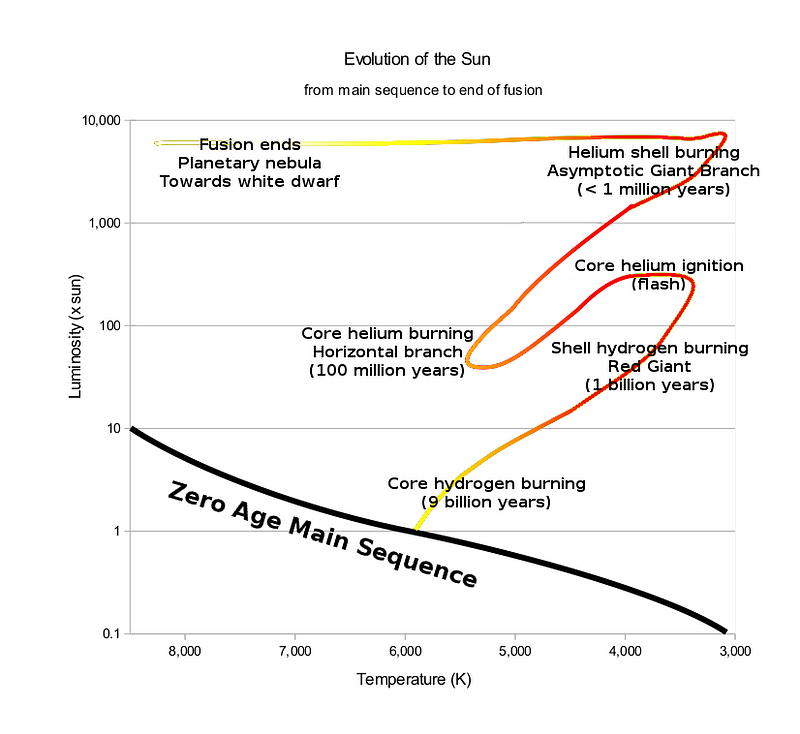
Inside a higher-mass star, temperature is everything, and convection is too slow to mix the elements thoroughly enough. And that’s why even the helium our Sun is making in its core today will help quench the fusion reactions billions of years from now; it would take hundreds of billions of years for the whole core of a star to circulate new elements into it. (Something that does happen, but only in M-class stars.)
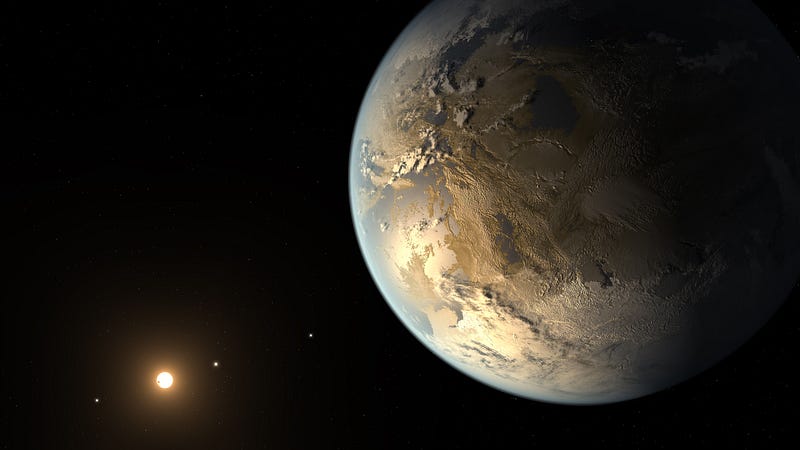
And that’s how stellar evolution really happens: a lot closer to “all-at-once” than most of us realize!
Leave your comments at our forum, and support Starts With A Bang on Patreon!