Could we use the Sun’s gravity to find alien life?
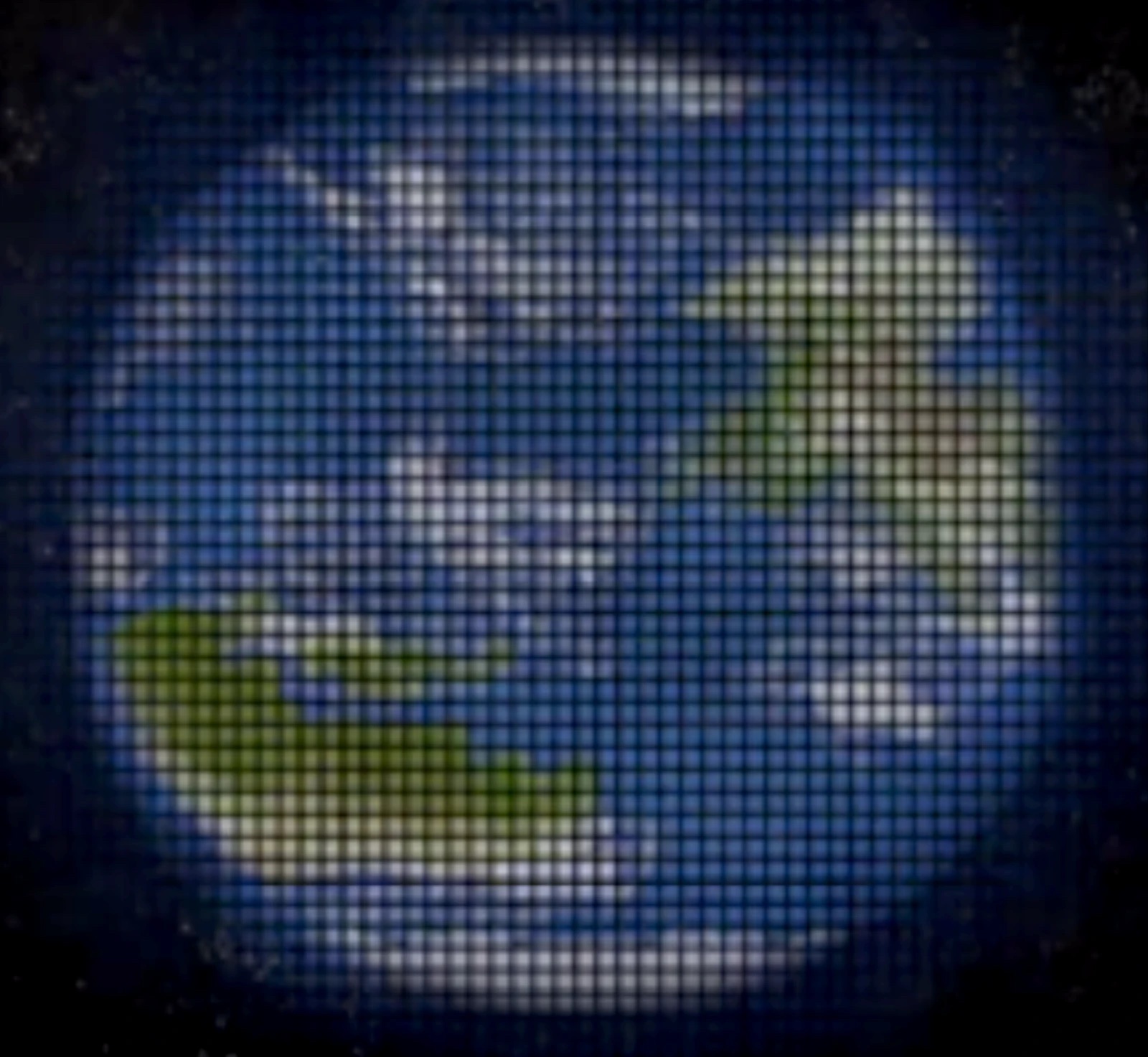
- Gravitational lensing is one of the most powerful astronomical phenomena out there, capable of stretching and magnifying the light from a background object that’s “lensed” by a massive, foreground object.
- Our strongest nearby source of gravity, the Sun, is itself capable of producing a gravitational lens, but only if the geometry is right: conditions that don’t begin until we’re 547 times the Earth-Sun distance away.
- Nevertheless, sending a spacecraft out to that precise distance, with the proper alignment to view an inhabited planet, could reveal details we’ll never see otherwise. Although it’s a long-shot, it’s one that our distant descendants might want to pursue.
Ever since the first human ancestors turned their eyes up toward the canopy of light shining in the night sky, we couldn’t help but wonder about the other worlds out there and what secrets they might hold. Are we alone in the Universe, or are there other living planets out there? Is Earth unique, with a saturated biosphere where practically every ecological niche is occupied, or is that a common occurrence? Are we rare in having had life sustain itself and thrive for billions of years, or are there many such planets like our own? And are we the only intelligent, technologically advanced species out there, or are there others for us to potentially communicate with?
For countless millennia, these have been questions that we’ve only been able to speculate about. But here, in the 21st century, we finally have the technology to begin answering these questions in a scientific fashion. We’ve already discovered more than 5000 exoplanets: planets in orbit around stars other than our own Sun. In the 2030s, NASA will likely design and build a telescope capable of determining if any of the nearest Earth-sized exoplanets to us are actually inhabited. And with future technology, we may even be able to image aliens directly.
But recently, an even wilder proposition was put forth: to use the Sun’s gravity to image a potentially inhabited planet, producing a high-resolution image that would reveal surface features to us just 25-30 years from now. It’s an enticing and amazing possibility, but how does it stack up to reality? Let’s take a look inside.
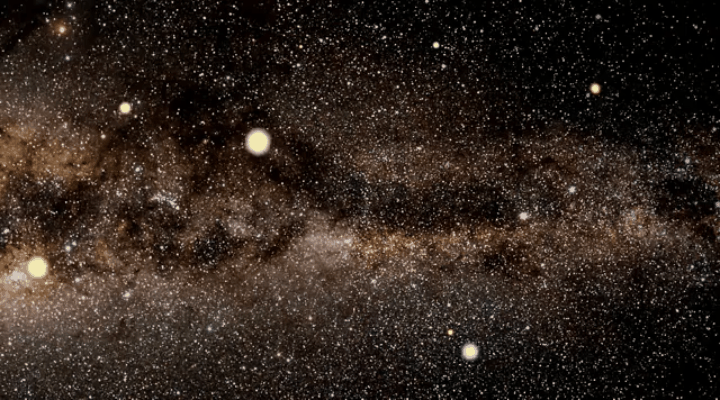
The concept: a solar gravitational lens
Gravitational lensing is a remarkable phenomenon, first predicted to arise within Einstein’s General Relativity more than a hundred years ago. The basic idea is that matter and energy, in all their forms, can bend and distort the very fabric of spacetime from their presence. The more mass and energy you have gathered together in one place, the more severely distorted the curvature of space becomes. When light from a background source passes through that curved space, it gets bent, distorted, stretched across larger areas, and magnified. Depending on the alignment of the source, the observer, and the mass that’s doing the lensing, enhancements of factors of hundreds, thousands, or even more can be possible.
Our Sun was the source of the first gravitational lensing phenomenon ever observed: where light from the background stars that passed close to the limb of the Sun during a total solar eclipse was seen to deflect from its actual position. Although the effect was predicted to be very slight — less than 2 arc-seconds (where each arc-second is 1/3600th of a degree) at the edge of the solar photosphere — it was observed, and determined to agree with Einstein’s predictions, refuting the Newtonian alternative. Ever since then, gravitational lensing has been a known, useful phenomenon in astronomy, with the most massive gravitational lenses often revealing the faintest, most distant objects of all that would otherwise be obscure due to our current technological limitations.
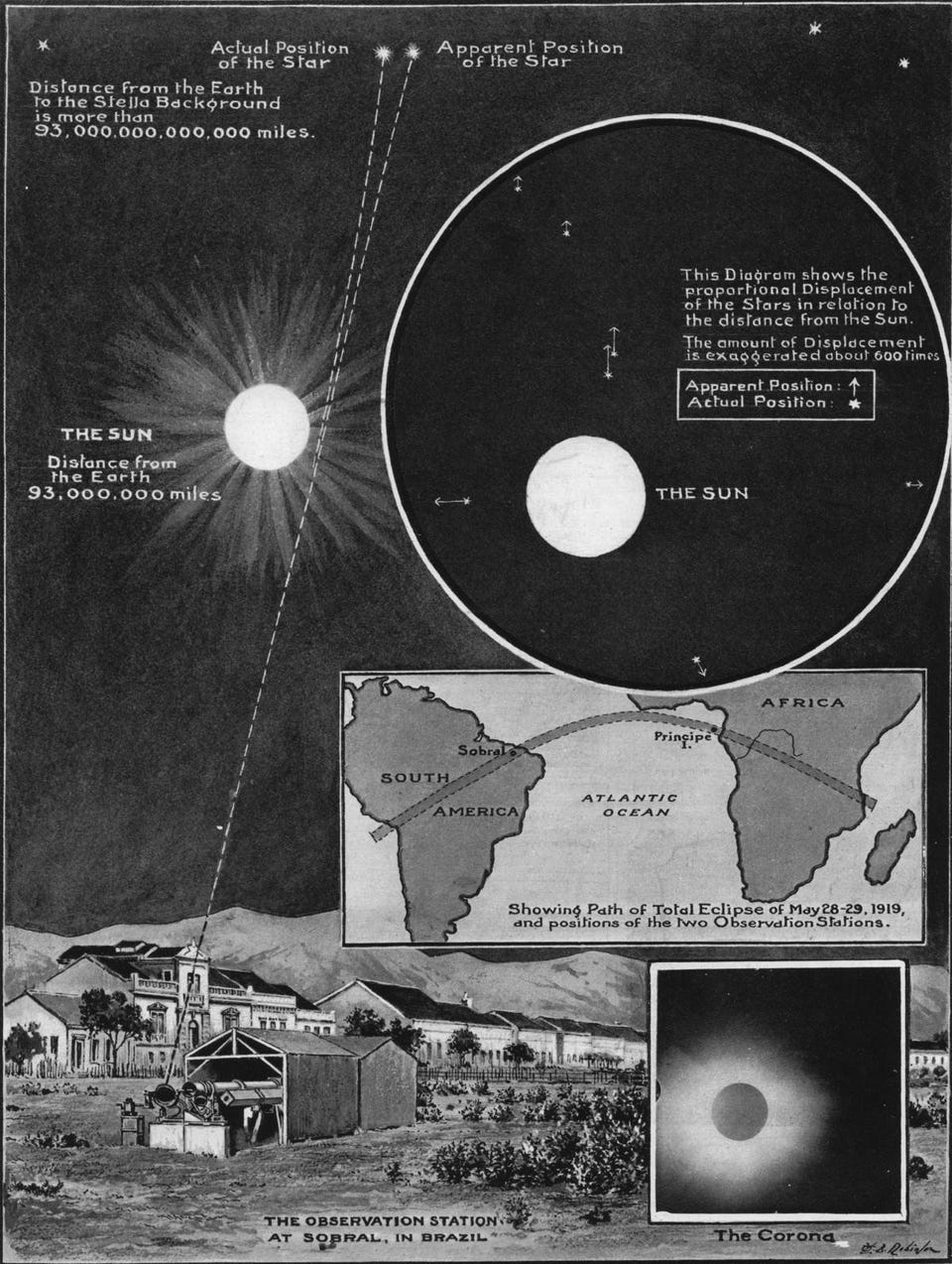
Theoretical possibilities
The idea to use the Sun as an effective gravitational lens to directly image exoplanets, however, requires a tremendous leap in imagination. The Sun, although massive, isn’t a particularly compact object: it’s approximately 1.4 million kilometers (865,000 miles) in diameter. As with any massive object, the most perfect geometry you can envision is to align an object with it and use the Sun as a lens to “focus” that object’s light from all around it onto a point. This is similar to how a converging optical lens works: the rays of light come in from a distant object, parallel to one another, they all strike the lens, and the lens focuses that light down to a point.
For an optical lens, the lens itself has physical properties, such as a radius of curvature and a focal length. Depending on how far away the object you’re observing is from the lens, the lens will focus a sharp image of that object at a distance equal to or greater than the focal length of the lens. Although the physics is very different for a gravitational lens, the concept is very similar. An ultra-distant source of light will have its shape extended into a ring-like shape with perfect alignment — an Einstein ring — where you have to be at least a “focal length” away from the lens itself in order for the light to properly converge.
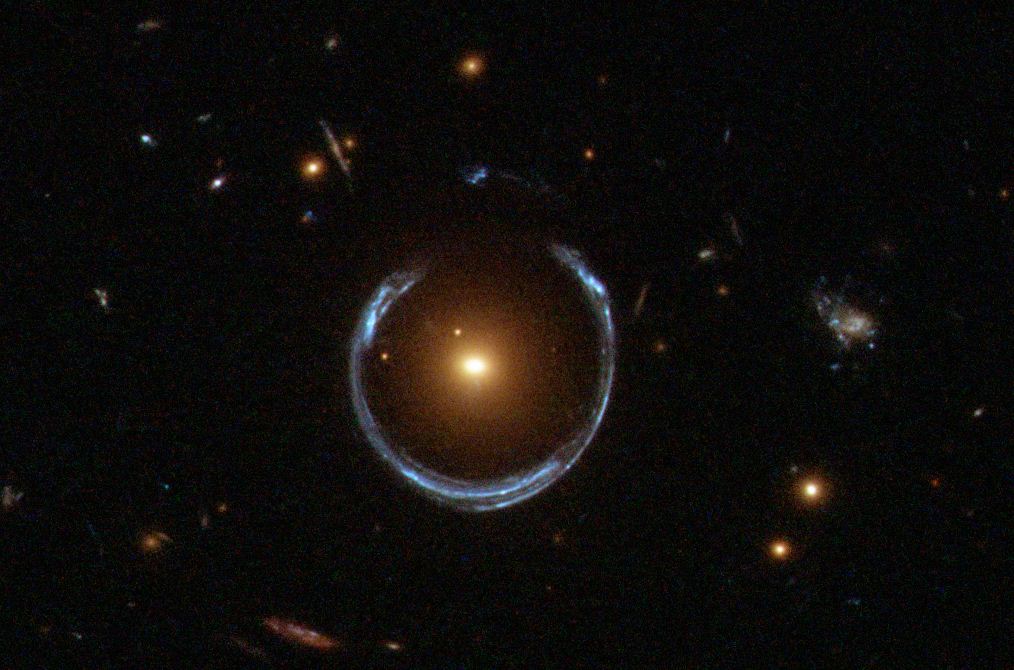
For a gravitational lens with the mass of our Sun, that focal length translates into a distance that’s at least 547 times farther away from the Sun than the Earth is at present. In other words, if we call the Earth-Sun distance an astronomical unit (A.U.), then we need to send a spacecraft at least 548 A.U. away from the Sun in order to gain the benefit of using the Sun to gravitationally lens a target of interest. As has been recently calculated in a proposal submitted to NASA, a spacecraft that could be:
- parked at this location,
- aligned with the Sun and an exoplanet-of-interest,
- and that was outfitted with the right equipment, such as a coronagraph, an imaging camera, and a sufficiently large primary mirror,
could image an Earth-sized exoplanet within 100 light-years of us with a resolution of merely tens of kilometers per pixel. Corresponding to a resolution of about 0.1 billionths of an arc-second, it would represent an improvement of about a factor of ~1,000,000 in resolving power over the best modern-day telescopes that have been designed, planned, and that are under construction today. The idea of a solar gravitational telescope offers up a tremendously powerful possibility for exploring our Universe, and isn’t one that should be taken lightly.
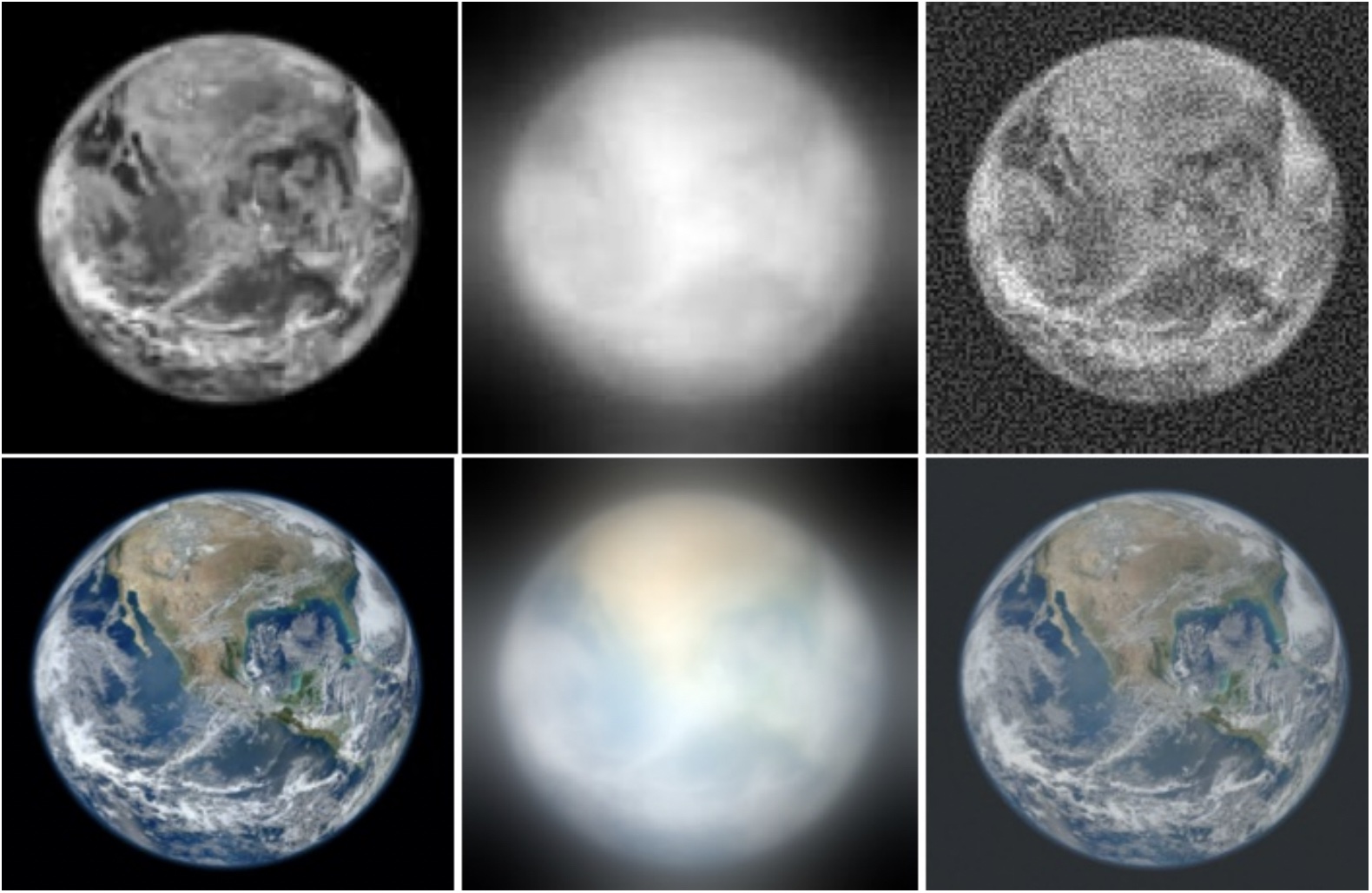
Practical limitations
Of course, all big dreams, as important as they are for firing our imaginations and spurring us onward to create the future we’d like to see, have to be met with a reality check. The authors of the proposal asserted that a spacecraft could be launched to this destination and could begin imaging a target exoplanet in just 25-30 years.
That, unfortunately, is way beyond the limits of current technology. The authors demand that the spacecraft leverage solar sail technology that does not yet exist.
Compare that with our present reality, where the only five spacecraft that are on current trajectories to exist the Solar System are Voyager 1, Voyager 2, Pioneer 10, Pioneer 11, and New Horizons. Of all of these spacecraft, Voyager 1 is presently the farthest and is also leaving the Solar System the fastest, and yet in the 45 years since it was launched, it has only traversed approximately a quarter of the necessary distance. It also leveraged numerous planetary fly-bys to give it gravity assists, which have also thrown it out of the plane of the Solar System and launched it on a trajectory that can no longer be controlled or even sufficiently altered.
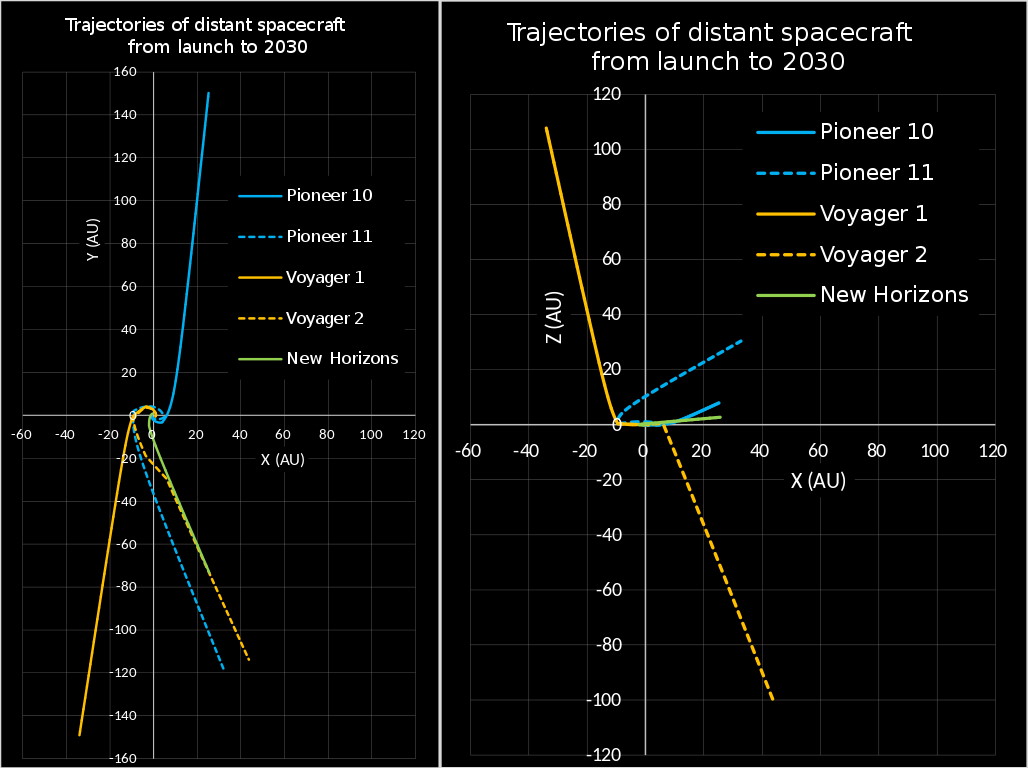
Yes, we could do something similar today, but even if we did, it would take nearly 200 years for the spacecraft to reach its target. Unless we develop new propulsion technology, the combination of rocket fuel and gravitational assists aren’t really capable of getting us to the needed distance in a shorter amount of time.
But that’s not the only problem or limitation that we’d need to reckon with. For any planetary target we’d dream about imaging, the “imaginary line” onto which the Sun would focus that planet’s light is only about 1-2 kilometers wide. We’d have to launch the spacecraft with such precision that it wouldn’t simply hit that line, but that it would remain on that line, and that’s a line that doesn’t begin until we’re nearly 100 billion kilometers away from the Sun. For comparison, the New Horizons spacecraft, launched from Earth to Pluto, was able to reach its target — at just 6% of the distance that a solar gravitational telescope would need to achieve — with an astounding precision of just ~800 kilometers. We’d have to do nearly a thousand times better over a journey that’s more than ten times distant.

But then, beyond that, we’d have to do something we’ve never done before: once the spacecraft arrived at its destination, we’d have to slow it down and stably keep it right on that 1-2 kilometer wide line in order to successfully image the planet. That means either loading the spacecraft up with enough on-board propellant that it can successfully decelerate itself, or developing the technology where it can auto-navigate itself to find, direct itself to, and enable itself to remain on that imaginary line so that it can conduct the necessary imaging.
More technological advances are needed in order to make this mission feasible, over and above current technology. We’d need a successful “double coronagraph,” one to block the light from our own Sun and one to successfully block the light from the parent star whose light might otherwise overwhelm the light from the target planet. We’d need to develop “pointing technology” that’s far superior to the limits of current technology, as the goal is to move within this 1-2 kilometer wide cylinder to construct a full map of the planet. This would require pointing and stability technology that represents approximately a factor of ~300 improvement over what a telescope like Hubble or JWST can achieve today; a remarkable leap that goes beyond our current capabilities.
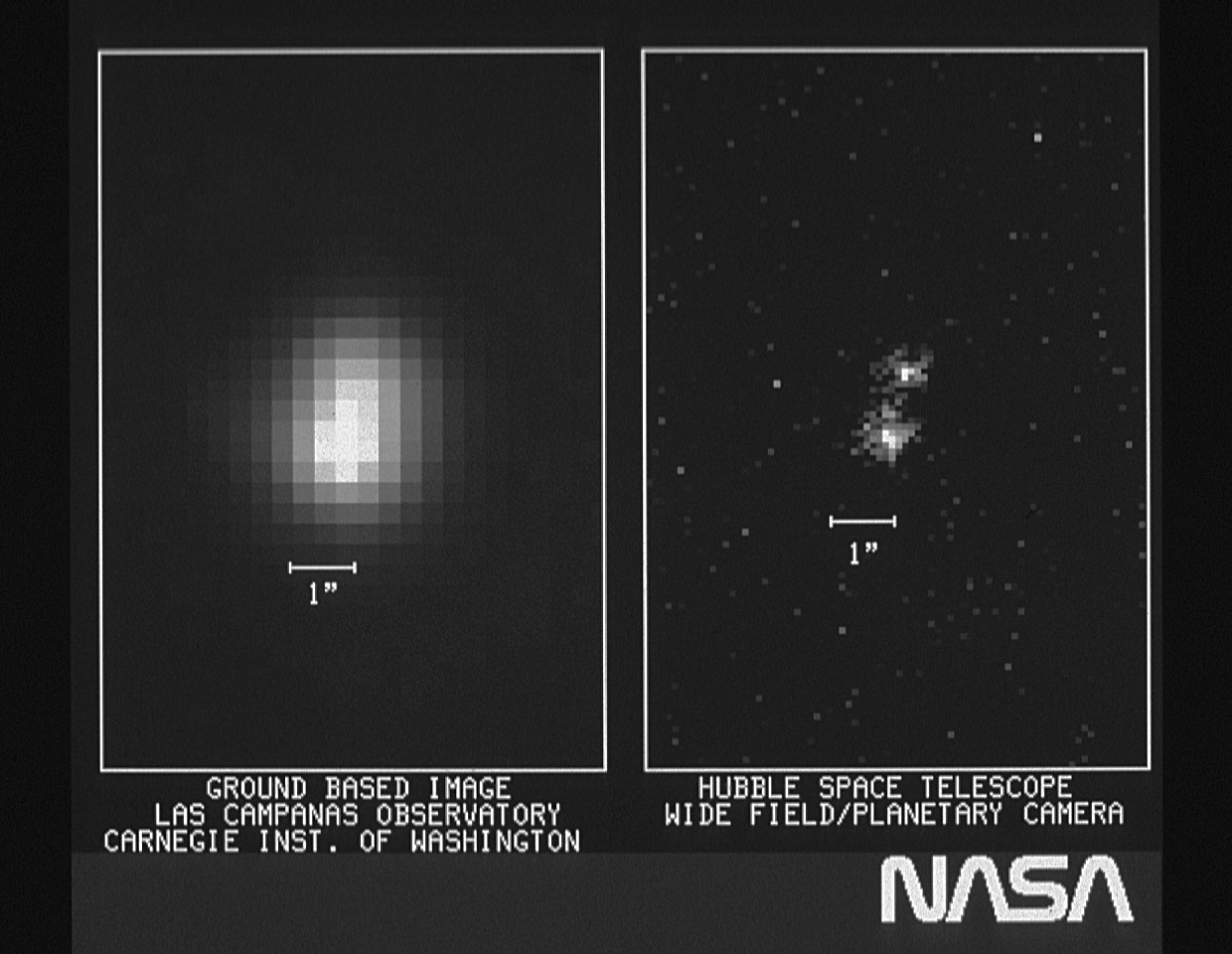
The proposal seeks to overcome some of these difficulties by appealing to new technologies, but those new technologies have drawbacks of their own. For one, instead of a single spacecraft, they propose using an array of small satellites, each with ~1 meter telescopes on board. While each satellite, if it reaches the proper destination, could take an image that corresponds to a particular “pixel” on the planet’s surface, but a million such pixels would be necessary to reach the goal of creating a megapixel image, and instead of needing to accurately shepherd one spacecraft to a hard-to-hit target, you’d need to send an array of them, compounding the difficulty.
For another, they propose whipping these spacecraft within ~10 million kilometers of the Sun to give them a gravity assist, but those distances risk frying many components of the satellite, including the needed solar sail; something that requires advances in materials that have not yet occurred. And at the accelerations required near perihelion — at distances comparable to the closest approach of the Parker Solar Probe — the sail supports themselves would not have enough material strength to withstand the forced they’d experience. All of these proposed solutions, to make the journey more feasible, come along with problems themselves that have yet to be overcome.
Additionally, this mission would only be doable for one target: we’d get one planet that we could choose to image with a mission such as this. Given that the optical alignments need to be accurate to within better than a billionth of an arc-second to make this type of imaging possible, it’s an extremely expensive, high-risk mission unless we already know that this is likely to be an inhabited planet with interesting features to image. Such a planet, of course, has not yet been identified.

What’s the best we can realistically hope for?
The best we can hope for is to pursue the development of new technologies for an advanced concept such as this — a novel coronagraph, greater precision in telescope pointing, rocketry technologies that allow for greater precision in hitting a distant target and decelerating to remain at such a target — while simultaneously investing in the nearer-term technologies that would reveal exoplanets that actually are inhabited. While today’s telescopes and observatories are capable of:
- measuring the atmospheric contents of Neptune-like (or larger) planets that transit in front of their parent stars,
- using coronagraphs to block a parent star’s light while directly imaging large, giant exoplanets that are located at least tens of A.U. from their parent stars,
- and to potentially characterize the atmospheres of exoplanets down to super-Earth (or mini-Neptune) sizes around the lowest-mass, coolest red dwarf stars,
the goal of measuring the habitability of an Earth-sized planet around a Sun-like star remains out of reach with the current generation of observatories. However, NASA’s next flagship astrophysics mission after the Nancy Grace Roman Telescope — a super-Hubble that would be larger than JWST and outfitted with a next-generation coronagraph — could find our first truly inhabited, Earth-sized exoplanet potentially as soon as the late 2030s.
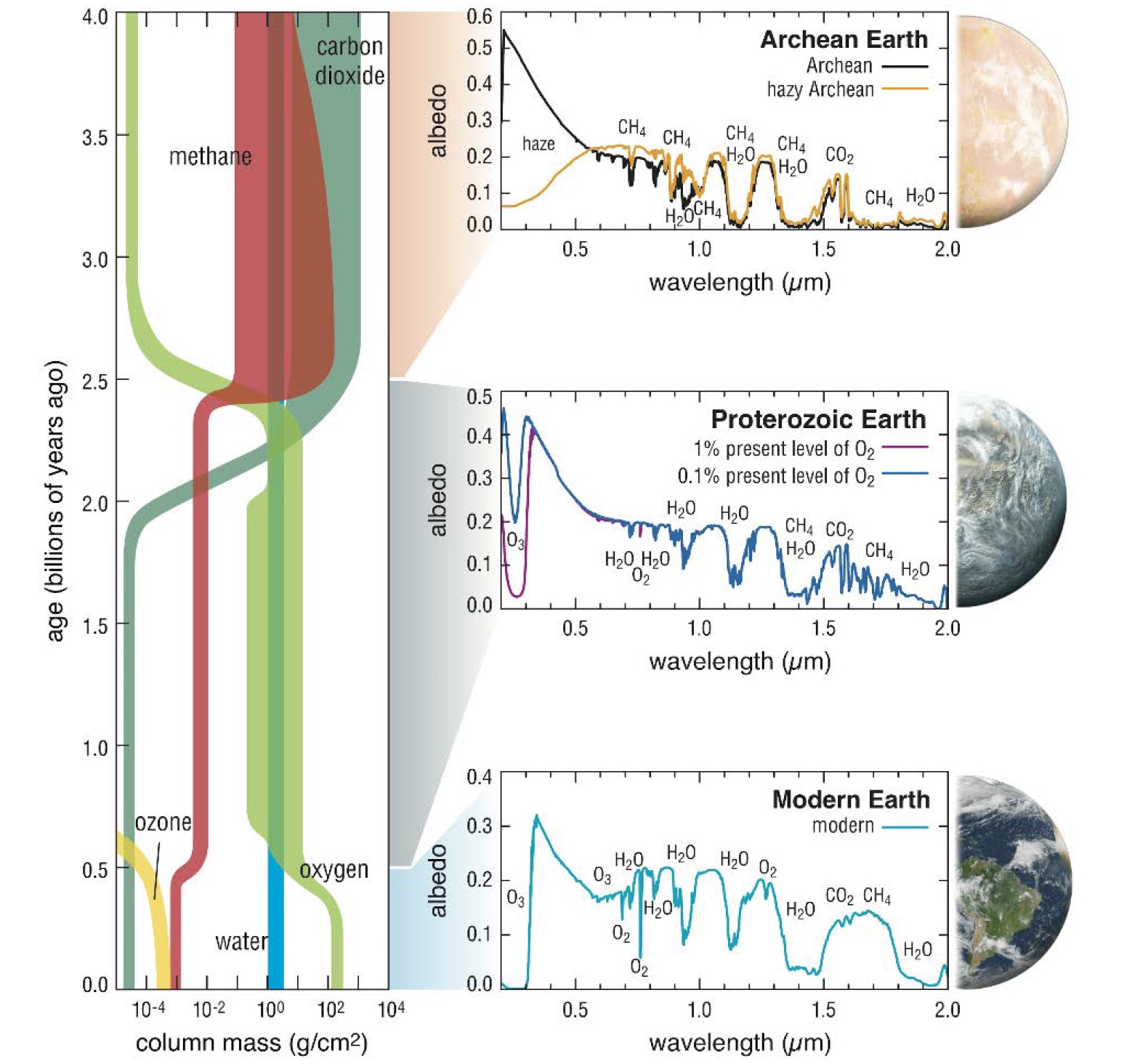
The most interesting planet to image, from the perspective of inhabitability, would be one that’s “saturated” its biosphere with life, just as Earth has. We don’t need to image an exoplanet in gory detail to detect such a change; simply measuring a single pixel of light and how it changes over time can reveal:
- whether the cloud cover changes as the planet rotates,
- whether it has oceans, icecaps, and continents,
- whether it has seasons that cause planetary color changes, such as from brown-to-green-to-brown,
- whether the gas ratios in the atmosphere change over time, as they do for gases like carbon dioxide here on Earth,
- and whether there are complex molecular biosignatures present in the planet’s atmosphere.
But once we have our first signs of an inhabited exoplanet, we’ll want to take that next step, and know precisely, in the greatest detail possible, what it looks like. The idea of using a solar gravitational telescope offers the most realistic possibility of creating a high-resolution image of an exoplanet’s surface without having to physically send a space probe multiple light-years away to another planetary system. However, we’re nowhere near being able to conduct such a mission on timescales of two or three decades; this is a multi-century project for us to invest in. That doesn’t mean it isn’t worth it, however. Sometimes, the most important step in reaching a long-term goal is simply figuring out what to strive for.