NASA’s super-Hubble to finally find inhabited planets
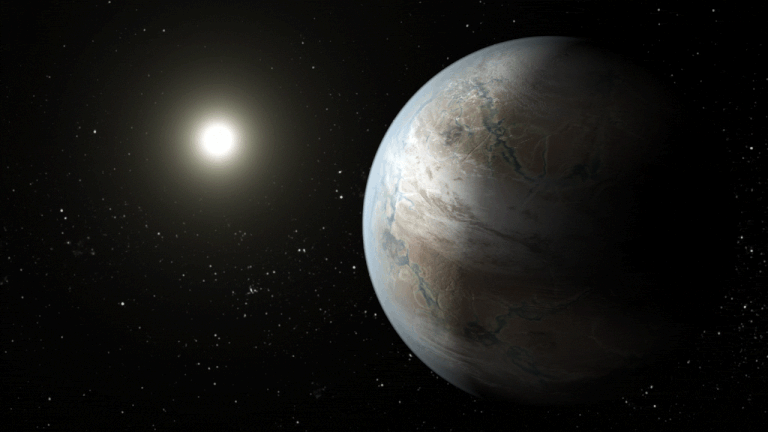
- Now that we've discovered thousands of exoplanets, we can estimate how many planets in the Milky Way are potentially already inhabited: the answer is in the billions.
- Yet there needs to be a giant technological leap in order to measure whether these worlds — exoplanets and, in some cases, exomoons — might actually be harboring life.
- These plans reflect exactly what NASA is planning as we move forward into the future, and a new "super-Hubble" telescope will be exactly the right tool to find our first signs of life beyond the Solar System.
Ever since our ancient ancestors first looked up at the stars, one question has given us a ceaseless sense of wonder: who else is out there? Out there around those glittering orbs of light in the sky, we know there are a great variety of planets and moons, including those with sizes, masses, temperatures, and compositions not found anywhere in our own Solar System. With approximately 400 billion stars in our Milky Way alone, it’s only a matter of time before we stumble upon a planet orbiting one of them that not only has the right conditions for life, but where life has emerged, survived, and thrived.
Starting in the 2030s, if all goes according to plan, NASA will launch a super-Hubble flagship mission that will identify and determine the properties of the first inhabited world beyond our own Solar System. Although it requires bold planning and a substantial (but not bank-breaking) investment to bring it to fruition, this new telescope will be the very first one with the extraordinary capability to:
- look at any star within a certain number of light-years,
- block out the light from the parent star,
- find and characterize any Earth-size planets within the star’s so-called “habitable zone,”
- and determine whether life on that planet has transformed the contents of its atmosphere.
If life is anything other than rare in the Universe, this telescope will find at least one inhabited world beyond our Solar System. Here’s the incredible science of how.
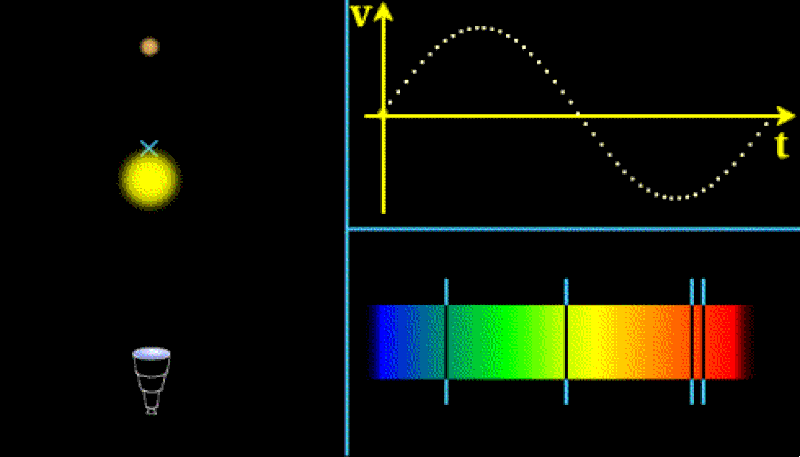
What we can do today, although it’s quite impressive, isn’t nearly enough to enable us to answer the question of whether an exoplanet has life on it or not. Although we’ve gotten very good at finding planets around stars other than our own, we have severe limitations on what we can find as well as on what we can measure.
The two main methods we have for finding exoplanets are:
- the transit method, where an orbiting planet periodically passes in front of its parent star, blocking a portion of its light whenever it does,
- and the radial velocity/stellar wobble method, where an orbiting planet gravitationally tugs on its parent star, causing the star to move back-and-forth along our line-of-sight, periodically, in a fashion that reveals the planet’s mass (up to its uncertain angle of inclination) and orbital period.
Unfortunately, the transit method can only reveal planets where the alignment is serendipitously perfect, where the transit is rapid and frequent enough, and whose planets are large enough compared to their parent star to block an appreciable fraction of their light. If we were to view a Sun-like star with Kepler, TESS, or any of our other best planet-finding observatories, all would be incapable of finding an Earth-sized planet in an Earth-like orbit in that star’s planetary system.

If you do have a transiting planet, at least you have the potential to measure its atmospheric contents. During each transit, a tiny portion of the starlight will filter through the planet’s atmosphere, and by measuring the absorption spectrum of the planet, it’s possible to reveal the presence and composition of the planet’s atmosphere, dependent on the density of molecules present.
This is hugely important, because the one surefire way we have to tell whether a planet is inhabited or not — not just “potentially” habitable — is to measure the light coming from it and to determine what sort of chemistry has occurred on that world. If there’s evidence of widespread, ubiquitous, long-term biological activity, a planet-wide analysis of its light will reveal it.

Unfortunately, well over 99% of planets don’t transit their star from our perspective, and that means there’s only one way to examine the atmospheric contents of those worlds: through direct imaging. Using today’s technology, planets can be directly imaged, but only if:
- the light from the parent star can be effectively blocked,
- and the planets are large enough,
- self-luminous enough,
- and far away enough from their parent star to not get lost in its glare.
To date, the only exoplanets that have ever been directly imaged are large and well-separated from their parent stars.
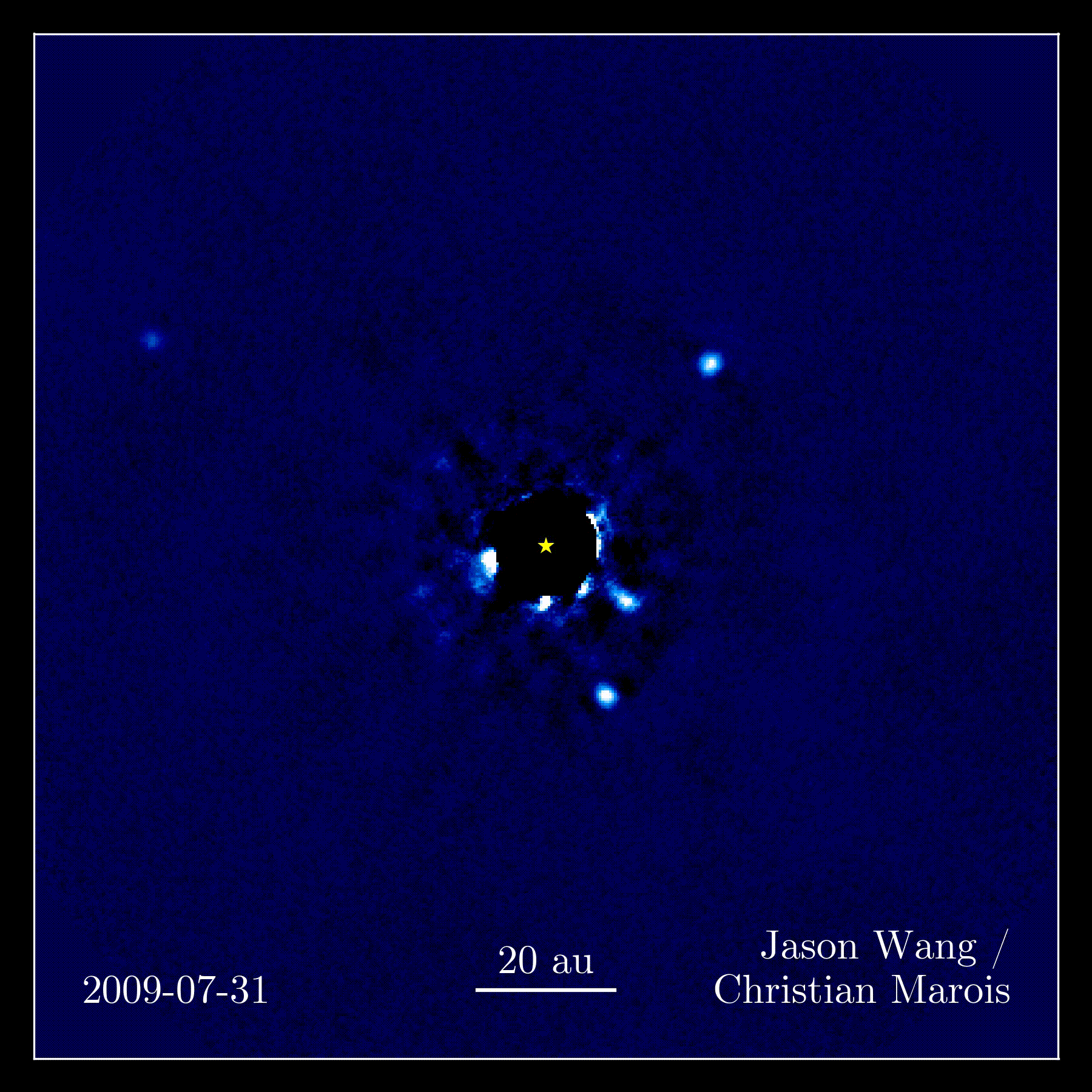
If you want to block out the light from a parent star, there are really only two ways to go about it.
- You can build a coronagraph into your telescope, a “shield” that blocks the light from a parent star while allowing the light from nearby to enter your telescope unimpeded. This is how we’ve traditionally imaged planets thus far, but there’s a drawback. If you simply create a “disk” to block the light from the star, the optical “edge effects” from the perimeter of the disk will create a series of bright concentric circles surrounding the blocked star, many of which will still be bright enough to drown out the faint signal of a planet. Even the state-of-the-art coronagraphs aboard Hubble and the JWST possess this problem; neither one are even capable of detecting Jupiter-size planets in Jovian orbits around Sun-like stars.
- You can, alternatively, fly a “starshade” a large, specific distance away from your spacecraft: so that it blocks out the light from your target star-of-interest. With an optically perfect shape, so long as it remains properly positioned, it can allow planets that emit as little as one-ten-billionth of a parent star’s light to be imaged directly. The big catch is that it only works for one target star at a time, and literally takes a year or longer to position it properly.

Looking for life on planets beyond our Solar System is a numbers game, and doing anything in astronomy from space requires a cost/benefit analysis. Even if we could identify potential Earth-sized planets around Sun-like stars in advance with the radial velocity method, adding a (very expensive) starshade to a future space mission would only get us the light from a handful of relevant planets, tops. In an endeavor where large numbers of statistics are necessary to get the result you want, a starshade is a proposition fit for a gambler, not a scientist.
Fortunately, there are ways that modern coronagraphs can be vastly improved, and NASA’s very next flagship mission after JWST is going to do precisely that. The Nancy Grace Roman Telescope, with similar capabilities to Hubble but with between 50-and-100 times the field-of-view, will be outfitted with a new type of coronagraph: one never used before, particularly on a space telescope. Instead of a spherical “disk” to block the light, it will use a series of concentric circles to help counteract the natural interference patterns produced by the edge of the main coronagraphic disk. By using a similar technique to adaptive optics, the coronagraph can be tweaked to minimize the specific pattern produced by any one particular star, further increasing the power of the telescope to tease out the planetary light that might otherwise be lost in the glare of its parent star.
The coronagraph scheduled to be aboard the Nancy Grace Roman Telescope should enable a great step forward in exoplanet direct imaging. For the first time, we should be able to measure and detect the light from Jupiter-analogue worlds that orbit Sun-like stars. However, owing to the dual limitations of instrument design and the small aperture (including both low-resolution and relatively low amounts of light-gathering power) of the Roman Telescope, Earth-sized worlds will still be well out of reach.
But that’s okay; the goal of the Roman Telescope isn’t to find and characterize living worlds, but rather to measure large numbers of stars, galaxies, galaxy clusters, and supernova to try and better understand the Universe. It’s the next flagship telescope in the arsenal — presently unnamed but currently called LUVex, as a portmanteau of two different NASA flagship proposals — will be the one that gets us there. The basic idea is:
- to take a large, segmented telescope,
- with a slightly larger diameter than JWST (so that you can inscribe a complete 6.0-meter diameter circle inside of it),
- sensitive to the same set of wavelengths as Hubble (possibly including a little farther into the near-infrared than Hubble),
- with a next-generation coronagraph compared to the one that will be aboard Roman,
and use it to measure a series of Earth-sized planets around the nearest stars to Earth.

Given that there are, beyond our own Solar System:
- 9 star systems within 10 light-years of Earth,
- 22 star systems within 12 light-years of Earth,
- 40 star systems within 15 light-years of Earth,
- and 95 star systems within 20 light-years of Earth,
building a slightly larger telescope, and/or a slightly more efficient coronagraph, can increase the chances of finding an inhabited Earth-sized planet significantly. It’s part of why astronomers need to fight for every fraction-of-an-inch (or centimeter) that they can; when it comes to our discovery potential with an observatory such as this, light-gathering power and resolution are everything. As long as we can measure the light from any planet that orbits the star, rather than imaging the star itself, there are all sorts of vital pieces of information we can learn.
If we can image the planet, directly, over time, then simply being able to detect things like color and brightness over time will teach us tremendous amounts of information. We’ll be able to measure the planet’s albedo (or reflectivity), as well as how and whether that albedo changes over time. If there are icecaps that grow-and-shrink with the seasons, clouds in the atmosphere that vary with time, continents and oceans on a planet that rotates, and/or life forms that cover the land masses that change color (i.e., from green to brown and back again) with the seasons, simply measuring the color and brightness of the planet, over time, will reveal that. This remains true even if all we can see is a single pixel!
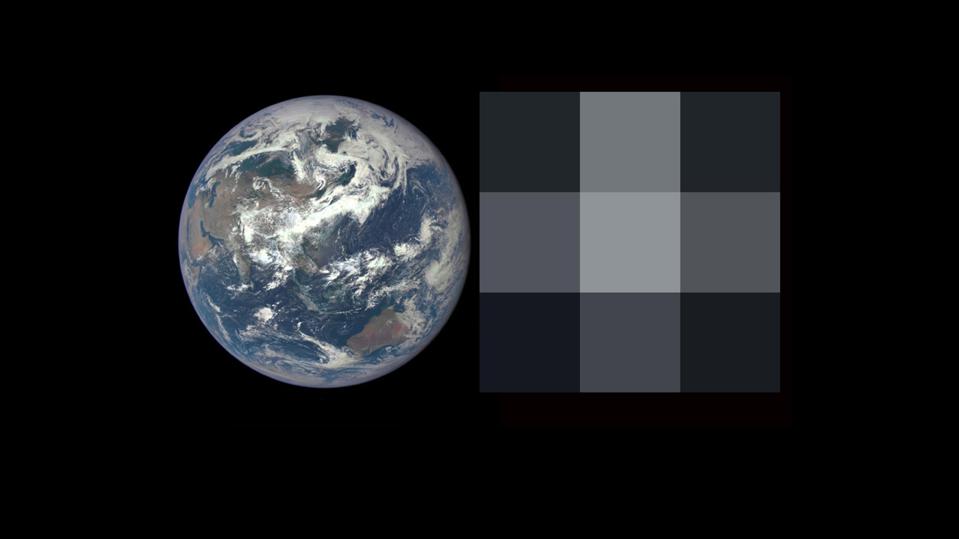
But the true power of direct imaging comes when we gather enough light to perform spectroscopy: to break the light emitted from the planet up into the individual wavelengths that make up this light. If there are molecular species in the atmosphere, they will emit light if they’re excited by the sunlight, and they will absorb light if they’re found along the line-of-sight from the planet’s surface (or oceans, or clouds) before that light reaches our eyes.
Every atom and molecule in the Universe emits and absorbs light at its own unique set of wavelengths, and that’s what makes spectroscopy such a powerful technique. We already know what types and ratios of atoms and molecules we expect the planets that form around stars to possess from the moment of their birth. This information comes from many lines of evidence: from examining the nebulae that planets form from (such as protoplanetary disks), from direct imaging of the atmospheres of the largest exoplanets (already visible today), from transit spectroscopy (already available for smaller, gaseous worlds), and from the planets and moons and other bodies in our Solar System.
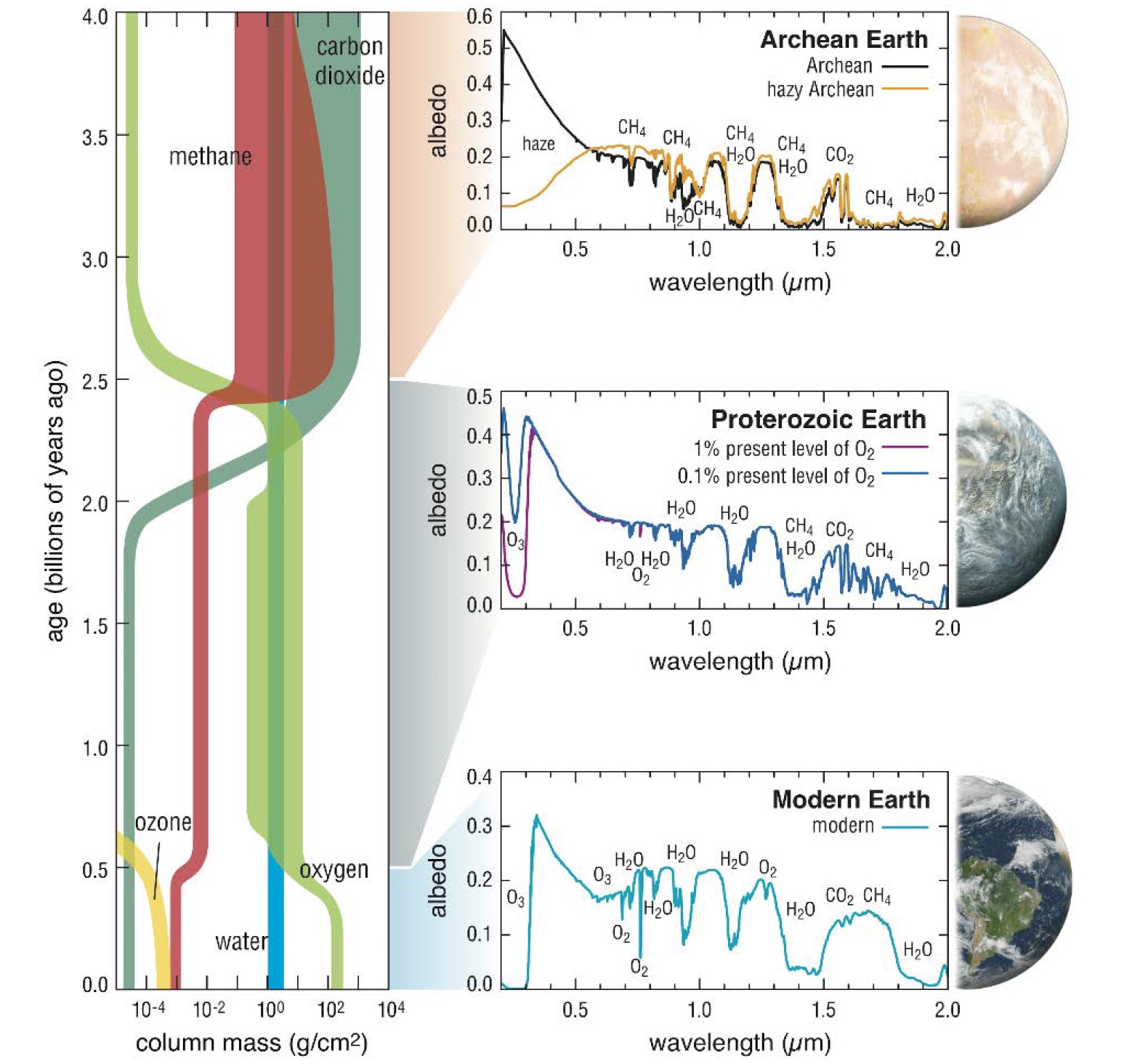
But we also know something remarkable about planet Earth: it possessed life from very early on in its history, and that life very rapidly — within the first 1-2 billion years of our Solar System’s existence — completely modified and altered the planet’s biosphere. If life can “saturate” the planet, as it did on Earth and as it’s expected to on any planet where life survives and thrives for any substantial amount of time, that signal will serve as the most unambiguous biomarker we could ask for.
If any of the nearest planets, and we’ll have between dozens and hundreds to examine, dependent on how ambitiously we design-and-build this upcoming observatory, have life on it that’s even as successful as Earth was back when our most complex organism was a single cell only capable of:
- anaerobic respiration,
- reproducing via mitosis,
- and lacking the ability to photosynthesize light into sugars and/or energy,
we’ll easily be capable of detecting such a success story. When you consider how much more successful life on Earth has been than this simple scenario presents, it makes you wonder just what we could discover if we invested in the right tools for the job.
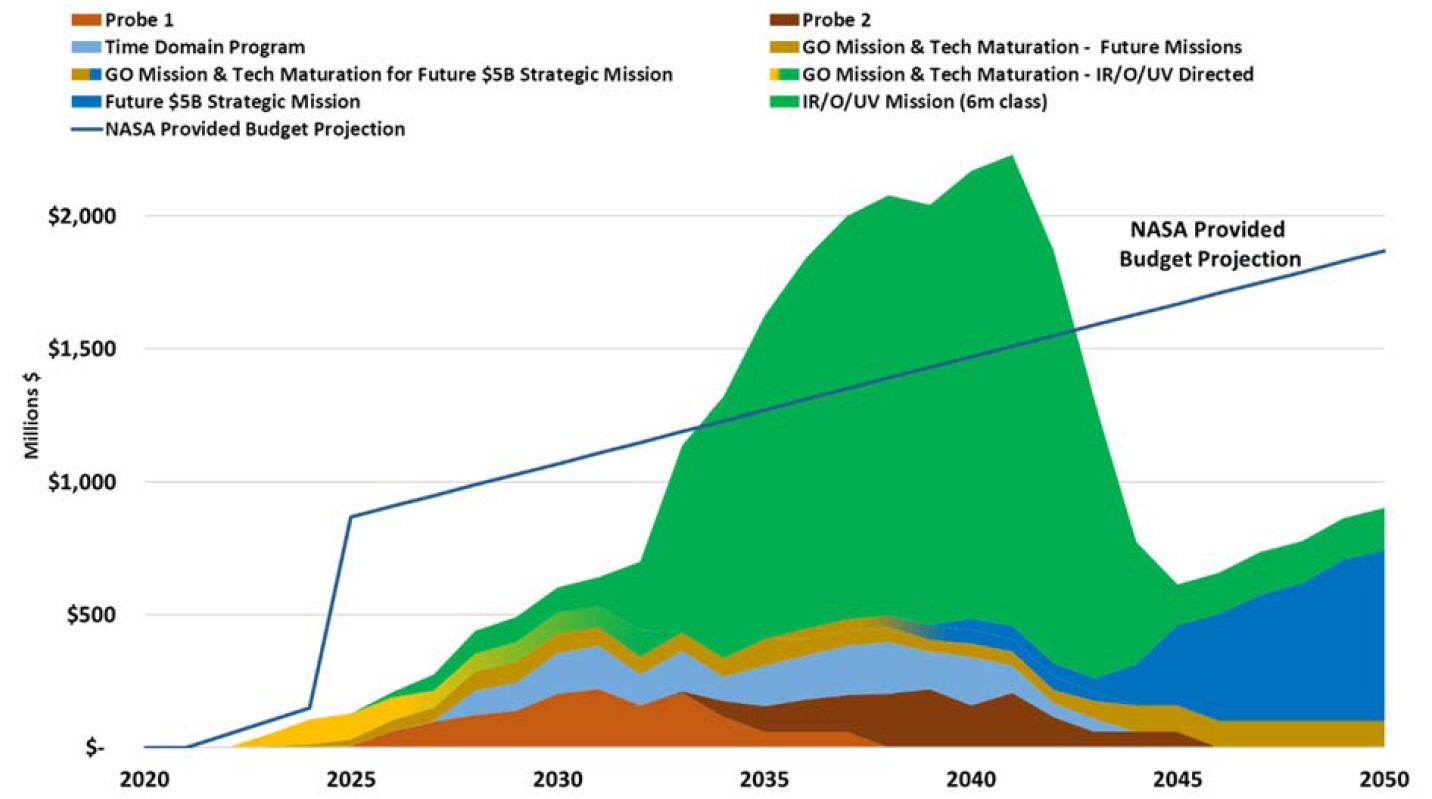
Of course, this also means something that’s truly compelling: if life isn’t rare in the Universe, and if complex, differentiated, and possibly even intelligent life arises easily and frequently once life takes hold on a planet, what we might discover could change everything. Imagine how differently we might live our lives if we knew there was an inhabited planet, teeming with life of the sort our imaginations can hardly even fathom, just in our cosmic backyard. For countless generations, we’ve wondered about whether we’re alone in the Universe or not. With a modest but sustained investment in the task ahead, we could finally give ourselves a real chance to answer that question affirmatively within the next two decades.
As exciting as this possibility is, and as world-changing as such a discovery would be, it’s completely dependent on what we choose to invest in as a society. For the cost of not quite 2 billion dollars a year — the entire budget of NASA’s astrophysics division — we could not only fund this mission and all of its prerequisites, but launch a new fleet of great observatories that open the entire Universe to unprecedented discoveries. But if we choose basic science as the arena where we pinch our pennies and cut our corners, it could be many lifetimes before we answer this existential question whose reach is finally within our grasp. Now is the time to choose wisely. If we do, the lessons we learn could impact the lives of every human to ever live from hereon out.