Strongest “missing link” in black hole physics discovered at last
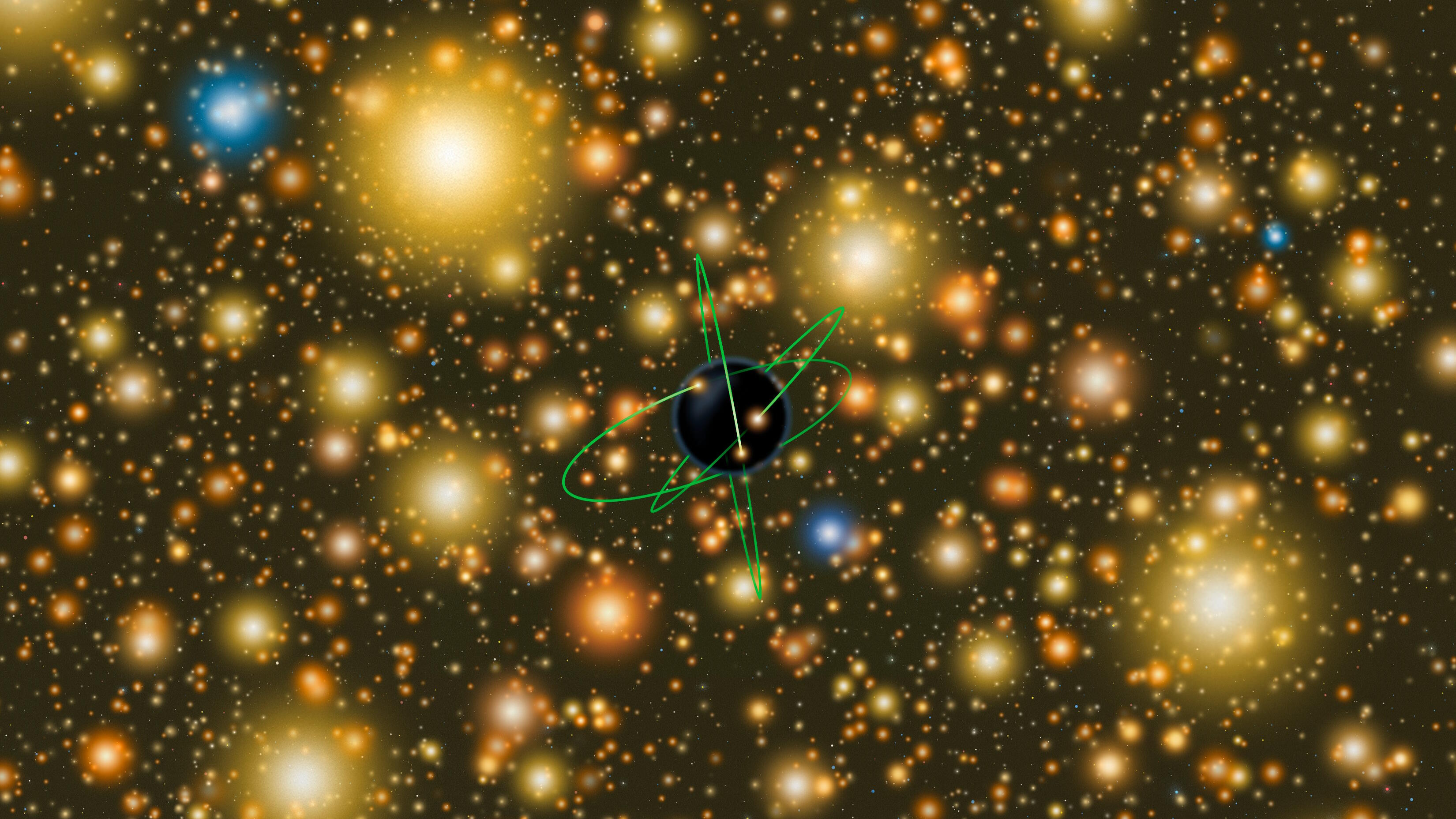
- Wherever massive stars form, black holes inevitably arise, ranging from a few solar masses up to 100 solar masses or even a little bit greater.
- At the cores of galaxies, supermassive black holes abound, with masses ranging from several hundred thousand to many billions of times the mass of our Sun.
- However, intermediate mass black holes have long remained elusive. A new discovery claims a black hole of 8200 solar masses at the center of globular cluster Omega Centauri, changing the game forever.
All throughout the Universe, black holes abound in great numbers and with impressive masses. On the one hand, black holes are known to arise from the corpses of stars, and are seen with orbiting companions, often emitting X-rays, and have also been discovered by inspiraling and merging through their gravitational wave emissions. Over 100 such black holes are known, with masses between ~3 and ~200 solar masses. On the other hand, there are supermassive black holes, predominantly found at the cores of galaxies, with much greater masses: from hundreds of thousands of times the mass of our Sun up to tens of billions.
But an in-between population of intermediate-mass black holes has long been suspected to exist, but has proven extremely difficult to unveil directly. One of the leading places to search for them has been near the centers of globular clusters: dense collections of anywhere between hundreds of thousands to several million stars, all bound together in a volume just a few tens of light-years in size. These globular clusters abound nearby, with more than 100 of them bound to our Milky Way and with over 1000 within the Local Group.
In a landmark new study, astronomers have just announced solid evidence for a central black hole in the globular cluster Omega Centauri, with a mass of at least 8200 solar masses. It’s the strongest evidence yet for an intermediate mass black hole, and may hold key implications for finding these objects all throughout the Universe.
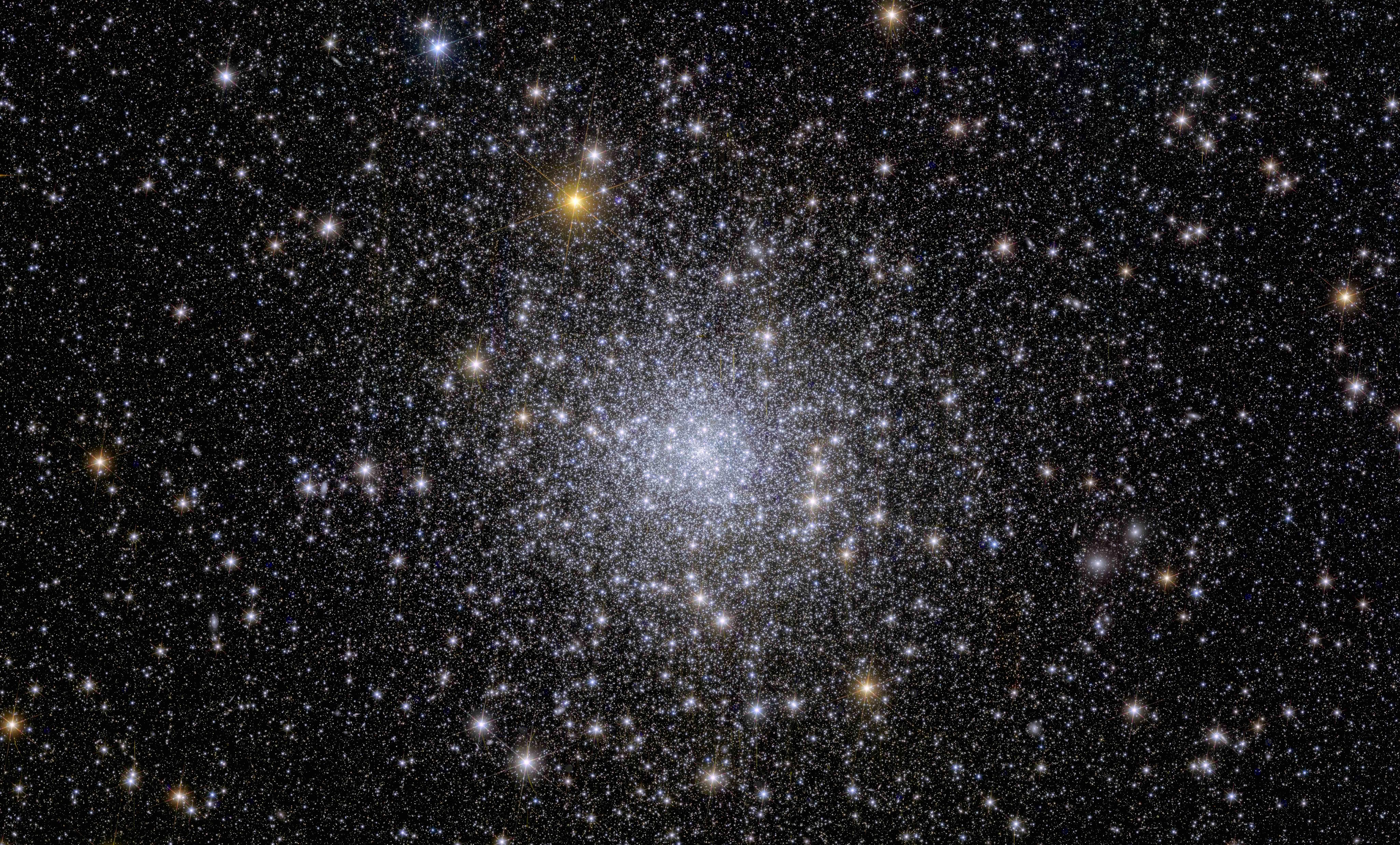
There are only a few pathways that the Universe has for enabling the formation of black holes in the first place. The most common mechanism is thought to come directly from massive stars that reach the ends of their life cycles. Whenever a star is fusing light elements into heavier ones, it releases energy in the form of radiation in its core, and that radiation is what holds the star up against gravitational collapse. Whenever the star’s supply of those key elements starts to run low, the radiation pressure decreases, and the star’s core contracts and heats up. If the new, high temperatures are sufficient to begin fusing the next heavier set of elements, the star’s life cycle continues; if not, the central core can collapse into either a neutron star or a black hole, dependent on mass.
- Some stars die in spectacular core-collapse supernovae, leaving black hole remnants behind from their cores.
- Other stars simply directly collapse, converting up to the entire mass of the progenitor star into a black hole.
- It’s also possible, perhaps even likely, that converging cold streams of gas can create even more massive black holes, of perhaps a few thousand or tens of thousands of solar masses, particularly in the early Universe.
- And finally, two neutron stars of sufficient masses can merge together, giving rise to a black hole post-merger.
All of these mechanisms are thought to lead to the production of black holes within our Universe.

Moreover, over time, black holes don’t just simply remain with the properties they had when they were created; they interact, merge, and devour matter that falls into them, leading to the potential for mass growth over time. Most of the black holes we know about were identified through one of two means:
- through the inspiral and merger of two lower-mass black holes, creating a heavier, higher mass black hole at the end,
- or because a black hole is actively feeding on matter, either from a transient source (like devouring a gas cloud or star) or from an ongoing source (like siphoning matter off of a binary companion).
In other words, most of the black holes that we actually find are discovered when they’re in the process of growing and gaining mass.
At the centers of galaxies, we’re learning that supermassive black holes reside there. Early on in the Universe, as shown by observatories like JWST, Chandra, Hubble, and ALMA, supermassive black holes were nearly as massive as the cumulative total amount of mass in stars in those galaxies, but by the present day, they’re usually only about ~0.1% as heavy as the total stellar mass of a galaxy. That gives us an interesting set of places to look for these in-between black holes masses, that are more massive than black holes arising from individual stars but less massive than the supermassive behemoths: either at the centers of small, low-mass galaxies, or deep inside the central cores of globular clusters.
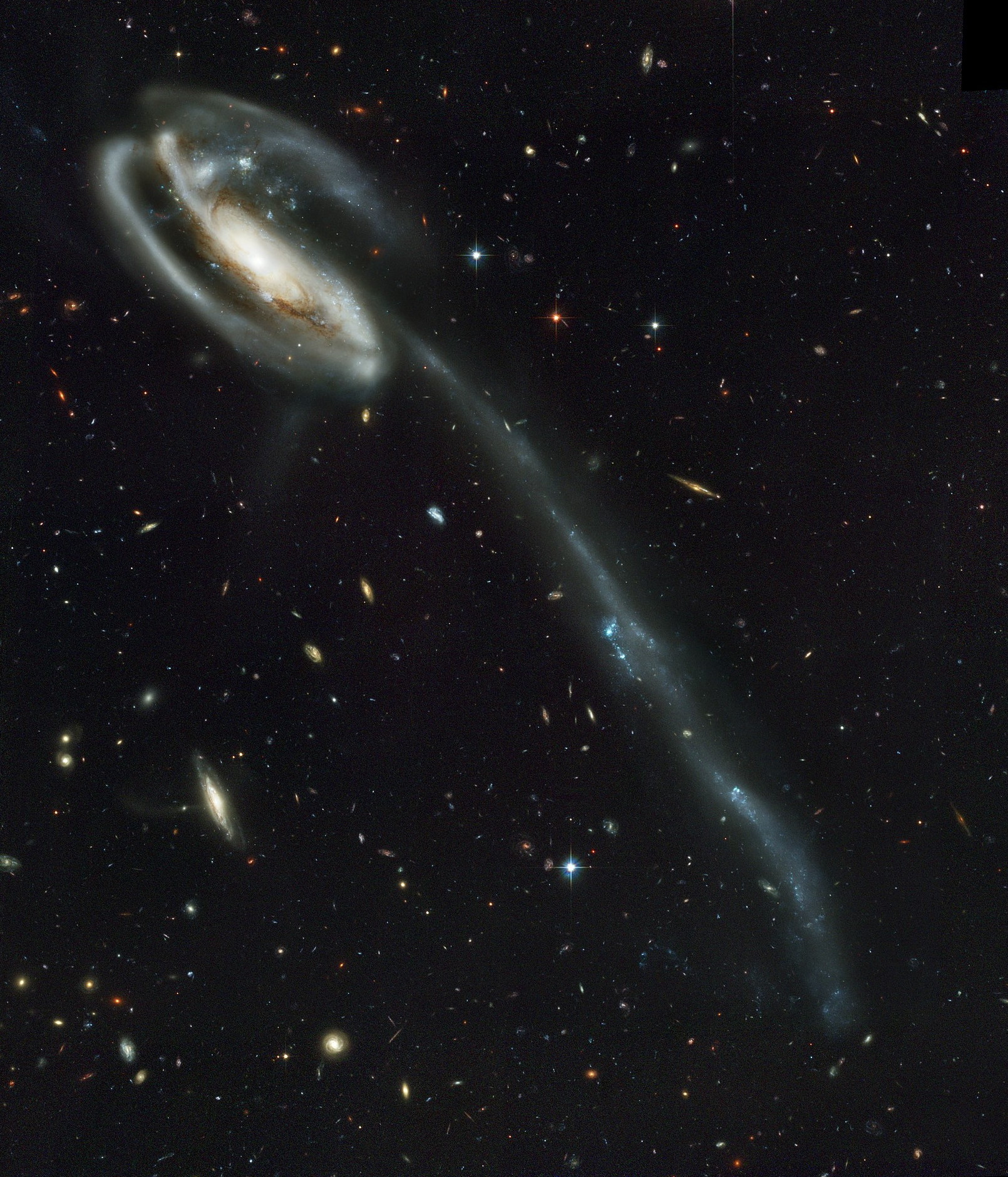
This latter option, of looking inside globular clusters for intermediate mass black holes, has been the subject matter of many searches over recent decades by astronomers. Globular clusters have many advantages in this quest.
- They’re of modest masses themselves: between ~105 and ~107 solar masses, indicating that black holes in the range of ~thousands of solar masses should be present within them.
- Globular clusters are densely packed, with all of their stars located within just a few tens of light-years of one another.
- Globular clusters are predominantly old, meaning that they’ve persisted for many billions of years, with many of the known globulars having most of their stellar populations dated at ~12 billion years or more.
- Globular clusters are ubiquitous and close by, with over 100 of them bound to the Milky Way and located within only a few thousand or ten thousands of light-years from us.
- And globular clusters also exhibit a property known as mass segregation, which happens to astronomical systems that are gravitationally bound over time. On long enough timescales, the heaviest masses sink toward the center, leaving the lighter-mass objects more loosely bound and located preferentially toward the outskirts.
All of that, put together, provides an excellent set of motivations for scouring globular clusters, and in particular, the centers of globular clusters, for the possibility of housing intermediate mass black holes.
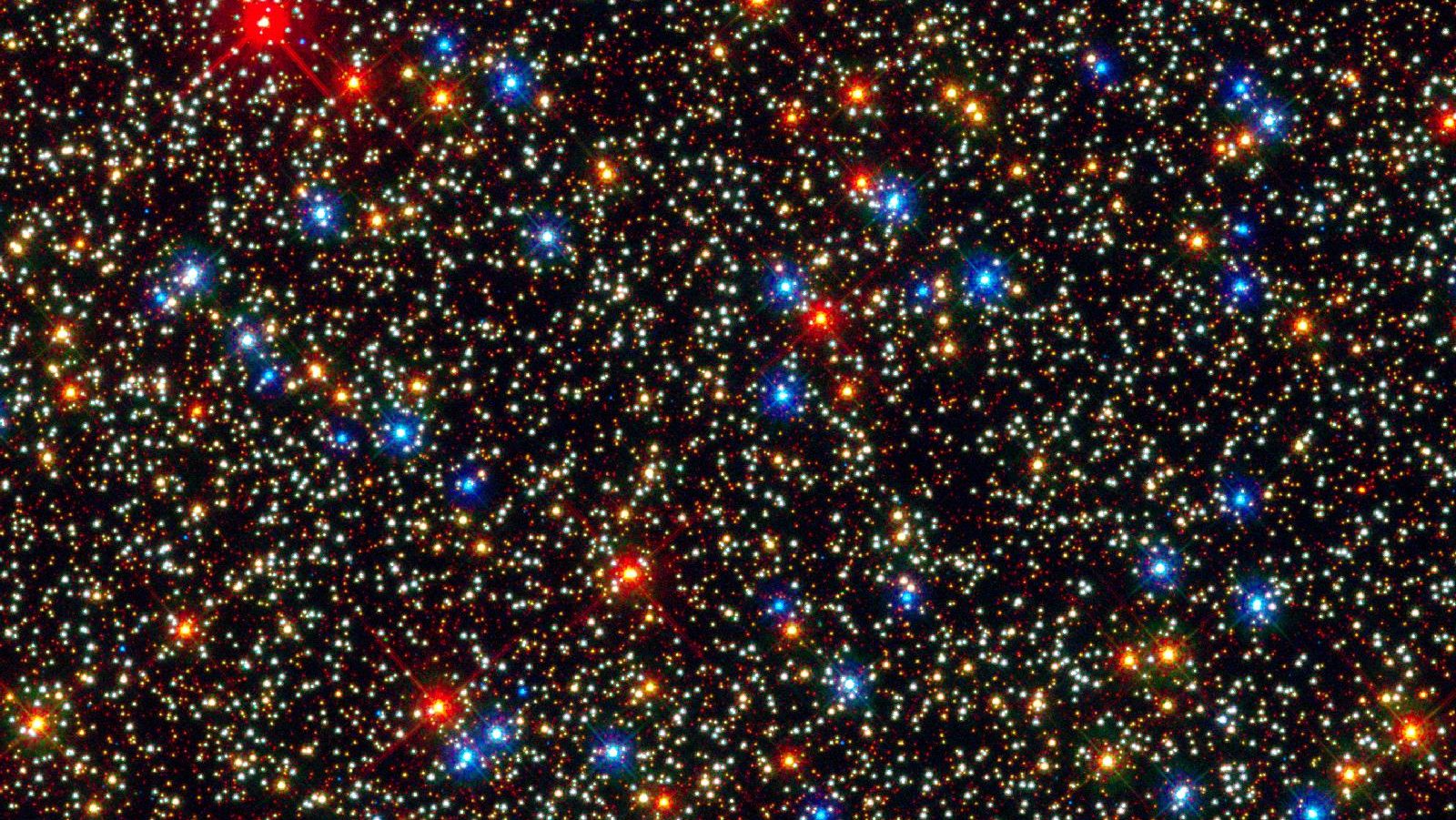
Searches have been conducted using millisecond pulsars as tools, noting that populations of these pulsars, which are very accurate clocks, would experience jerks and jounces (first and second derivatives of their acceleration) in their timing. Simulations predict that intermediate mass black holes should indeed form in globular clusters, with masses greater than 100 solar masses but below 100,000 solar masses. Using a proposed but not-yet-built tool such as the next-generation Very Large Array (ngVLA), scientists could be sensitive to intermediate mass black holes in globular clusters around galaxies located up to ~80 million light-years away. And globular clusters Messier 62 and Messier 22 were both shown to have black holes within them, but of the stellar mass, rather than intermediate mass, variety.
However, it was a globular cluster located on the outskirts of our galaxy and instead bound to the Sagittarius Dwarf Galaxy, Messier 54, that gave us our first indication of an intermediate mass black hole at the center of a globular cluster. By noting the motions of many stars near the globular center’s core, astronomers concluded there was a strong likelihood of a black hole of around 9400 solar masses: suggesting that these “missing link” black holes did indeed exist. However, much like other intermediate mass black hole candidates, like:
- HLX-1 found near galaxy ESO 243-49,
- a candidate tidal disruption event, where a possible intermediate mass black hole shredded a star,
- the center of globular cluster B023-G078 found around the Andromeda galaxy,
- central measurements of stellar motions within Omega Centauri,
- or within Messier 4,
the evidence has always been fairly inconclusive. The definitive evidence ruling out alternative explanations has thus far been lacking.
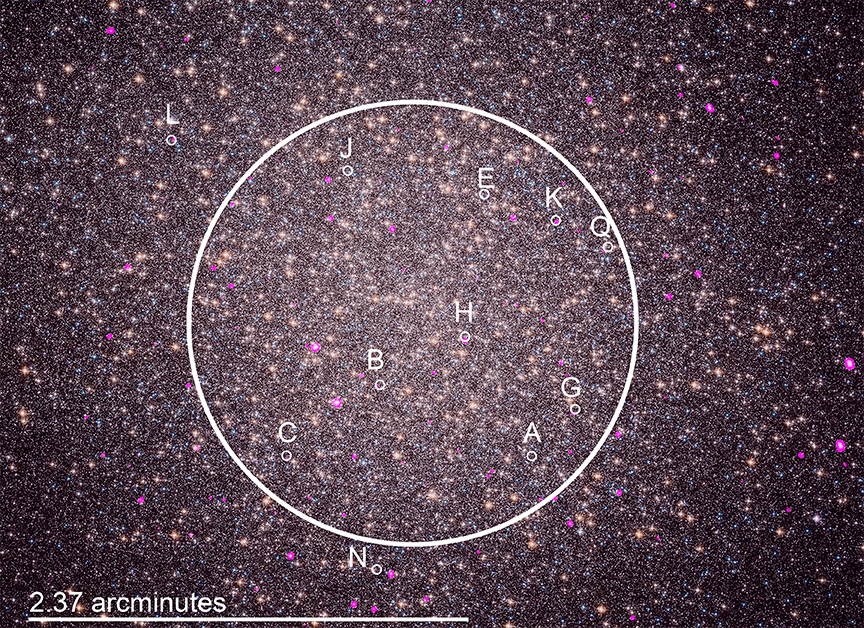
However, two new studies published on July 10, 2024 finally give us the evidence we need to declare “discovery!” when it comes to intermediate mass black holes. The key object is indeed globular cluster Omega Centauri: the most massive globular cluster known within the Milky Way galaxy and one long suspected to contain an intermediate mass black hole. Located a meager 17,000 light-years away, its vital stats are that:
- it has a radius of roughly 75 light-years,
- has a total mass of around 4 million solar masses,
- and is estimated to contain around 10 million stars.
Unlike most globular clusters, which are thought to have originated from a major episode of star formation from a much more massive cloud of gas than typically creates open star clusters — something akin to what we’re seeing occurring right now in the Tarantula Nebula — there’s substantial evidence that Omega Centauri took a much more uncommon path toward becoming a globular cluster: that it instead is the remnant core of a largely-destroyed dwarf galaxy that was gobbled up by the Milky Way long ago. Unlike globulars formed from super star clusters, Omega Centauri’s low (but not absurdly low) abundance of heavy elements, its motion through the Milky Way, and its somewhat young age of “only” 11.5 billion years (for a globular cluster), combined with a complex variety of stellar populations found within it, all suggest this unusual origin for this object.
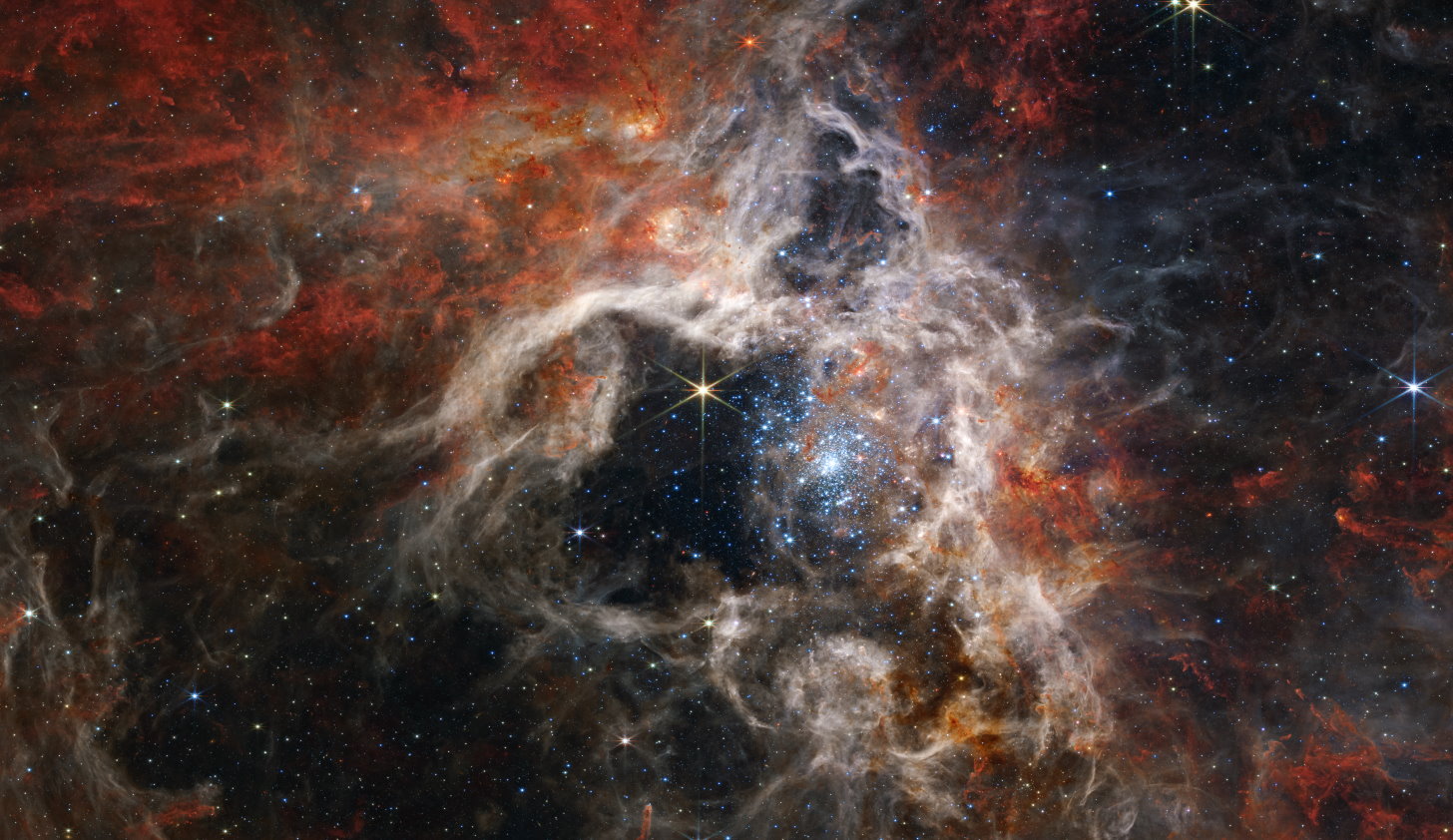
But how would we determine whether this globular cluster — or, for that matter, any nearby globular cluster — actually contains an intermediate mass black hole within it or not?
The key is to obtain tremendous amounts of high-quality data about the stars located within the globular cluster itself, including about the motion of those stars over time. Many of us remember seeing the spectacular images of the event horizons of the supermassive black holes at the center of the Milky Way, Sagittarius A*, as well as the one at the center of Messier 87: about 2000 times farther away but also about 1500 times as massive as the one at the center of our own galaxy. Although this is often treated as the “smoking gun” evidence for these supermassive black holes, there were two lines of evidence that were surefire giveaways before we actually acquired those measurements.
One line of evidence was the strong X-ray and radio emissions coming from these galactic centers: something seen emanating from supermassive black holes all across the Universe. The large angular features, owing mostly to the close proximity of these black holes to us, were joined by flares and/or jets, revealing a likely black hole. But even more compelling was the fact that we had tracked the motions of a large number of stars within the Milky Way’s center over many years, and they were all shown to be orbiting a common point from which no light was emitted. Just by understanding gravity, we could not only infer the presence of a black hole at the Milky Way’s center, but we could infer its mass as well.
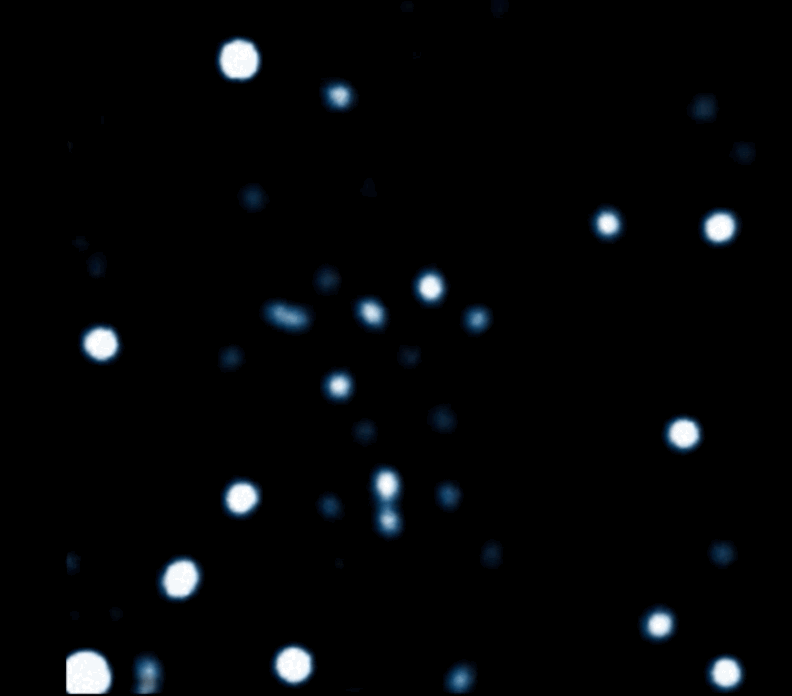
While a galaxy like Messier 87 is too far away to measure the orbits of individual stars near its center, that obstacle poses no problem for a globular cluster like Omega Centauri. At 17,000 light-years distant, it’s actually about 10,000 light-years closer than the stars orbiting the black hole, Sagittarius A*, at the Milky Way’s center. A herculean project was taken on to measure the proper motions (i.e., motions in three-dimensional space) of the stars within it, specifically the stars near the globular cluster’s center, over baselines of around 20 years, using the best data available to humanity over those timescales: from the Hubble Space Telescope.
In addition to this proper motion data, photometric data was taken of these stars as well, enabling astronomers to measure properties like their color, magnitude, and also to sort them by stellar population. More than one million stars (about 1.4 million) were measured within Omega Centauri in this fashion over those long baselines, as cataloged in the two recent studies led by Maximilian Häberle and Nadine Neumayer. Unlike previous studies, which could have been contaminated by:
- stellar mass black holes in the globular’s center,
- a lack of observed sensitivity to the cluster’s true center,
- and the lack of fast-moving hypervelocity stars found in the cluster,
these new papers, after sifting through all 1.4 million stars cataloged, identified seven “smoking gun” stars that all appear to have been kicked to speeds beyond the cluster’s projected escape velocity: something that can only be explained by being bound to a massive black hole.
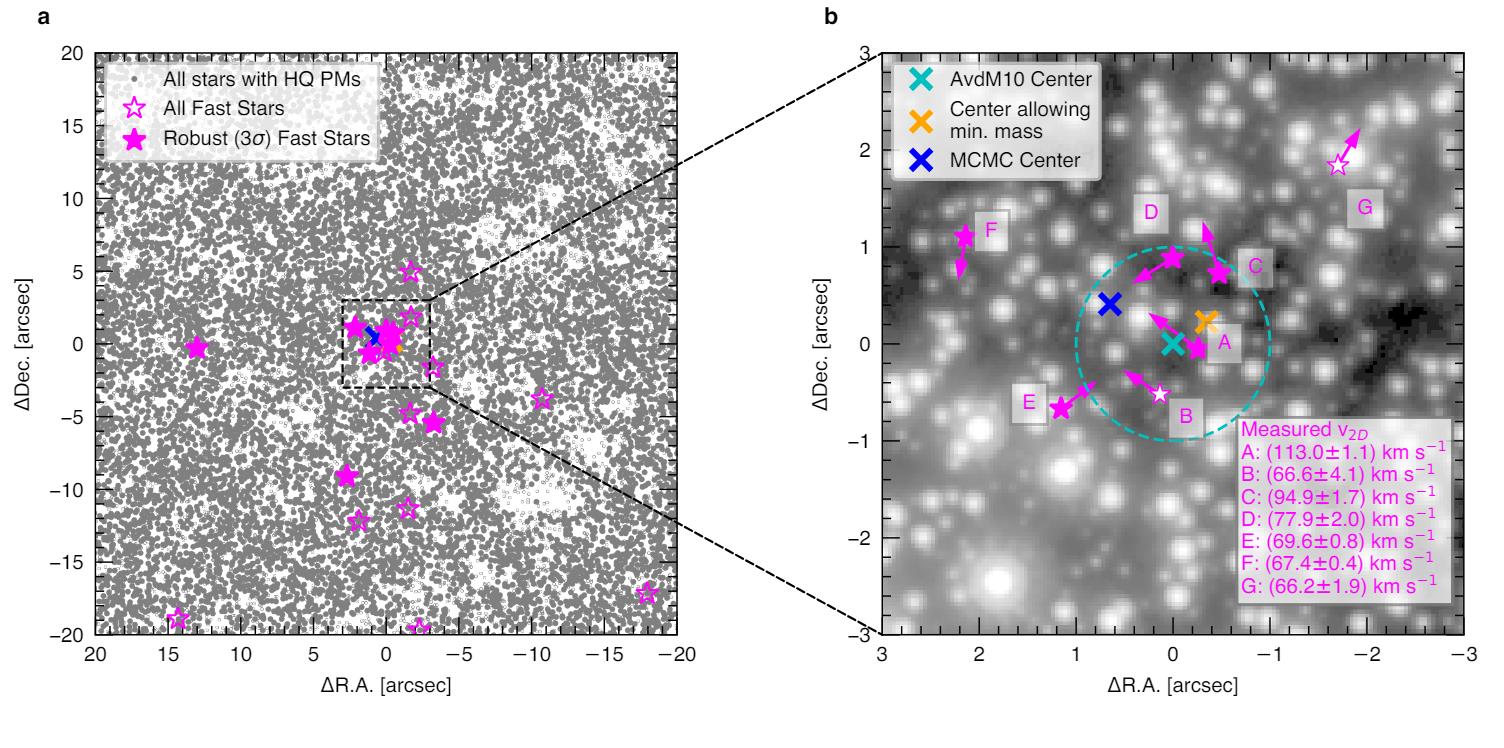
What’s remarkable about many of the images used to reconstruct the motions of these stars is that they weren’t acquired with any particular science goal in mind; they were mainly taken in order to facilitate the Hubble Space Telescope in calibrating its instruments. But just like many of the first JWST images — primarily used for calibration purposes — yielded an enormous amount of “bonus” science, the observations helped astronomers track myriads of these stars over very long periods of time. With Hubble’s remarkable resolution, especially at shorter wavelengths, even the rich star fields of Omega Centauri could be easily resolved into individual objects.
The fact that multiple hypervelocity stars were found indicates that they aren’t just experiencing a momentary gravitational interaction with a companion or a nearby interloper, but rather that there must be a concentrated mass nearby that they’re very likely gravitationally bound to. Just as the stars within the Milky Way’s galactic center can get up to remarkable speeds based on how tightly bound they are to the central black hole, the seven identified hypervelocity stars within the very central region of Omega Centauri reveal a black hole as well. Even with the lack of precise orbital data for these stars, the scientists involved in the study were still able to place a remarkable lower limit on the mass of the black hole inside: 8200 solar masses.
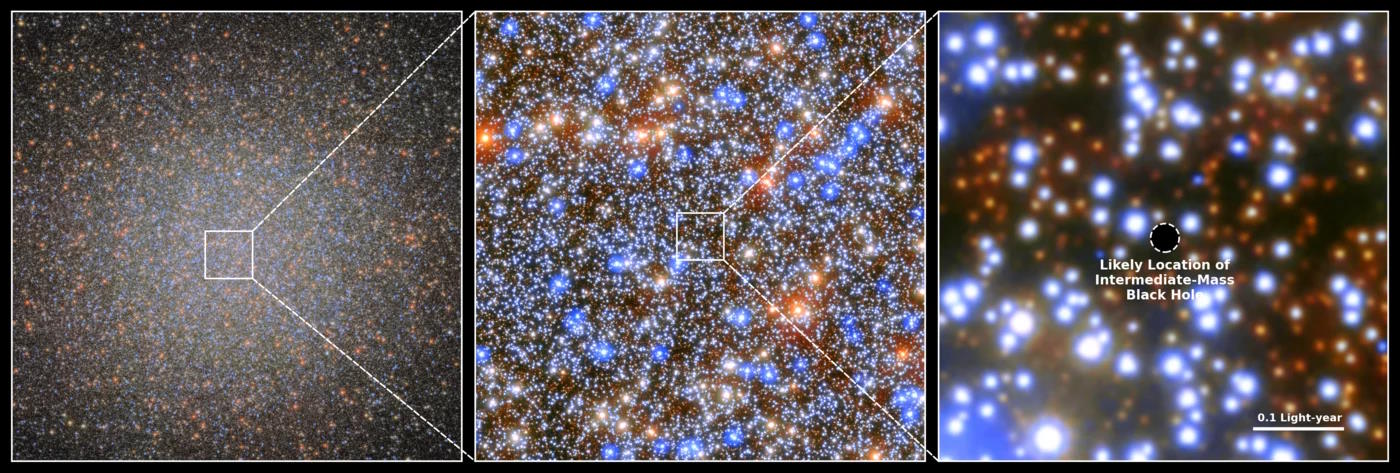
That figure, of 8200 solar masses, is more than a factor of 30 heavier than the heaviest stellar mass black hole known, and yet is more than a factor of 30 lighter than the lightest supermassive black hole known. For a long time, astronomers have wondered something like, “If there really is an intermediate mass black hole in these globular clusters, then where are the hypervelocity stars?” The discovery of not just one or two, which might be unrelated interlopers, but seven all located within the innermost arc-second of sky, is that “smoking gun” evidence we need to declare the discovery of an intermediate mass black hole. There is no other explanation that’s compatible with the known laws of physics.
However, we have to keep a limitation in mind: this black hole, at such a smaller mass than the one at the Milky Way’s center, will require very long periods of time — roughly a century, rather than roughly a decade — to map out the complete orbits of these fast-moving stars that revolve around them. Still, forthcoming JWST data should precisely measure the line-of-sight velocity of these stars to better precision than Hubble ever could, and long-term studies with ground-based facilities, including current facilities like the VLT and future ones like the GMT or ELT, could begin to determine how these stars accelerate. At long last, we’ve found our first surefire intermediate mass black hole, and now the follow-up quest to pin down its exact properties begins.