JWST’s new and improved exam of Uranus shines
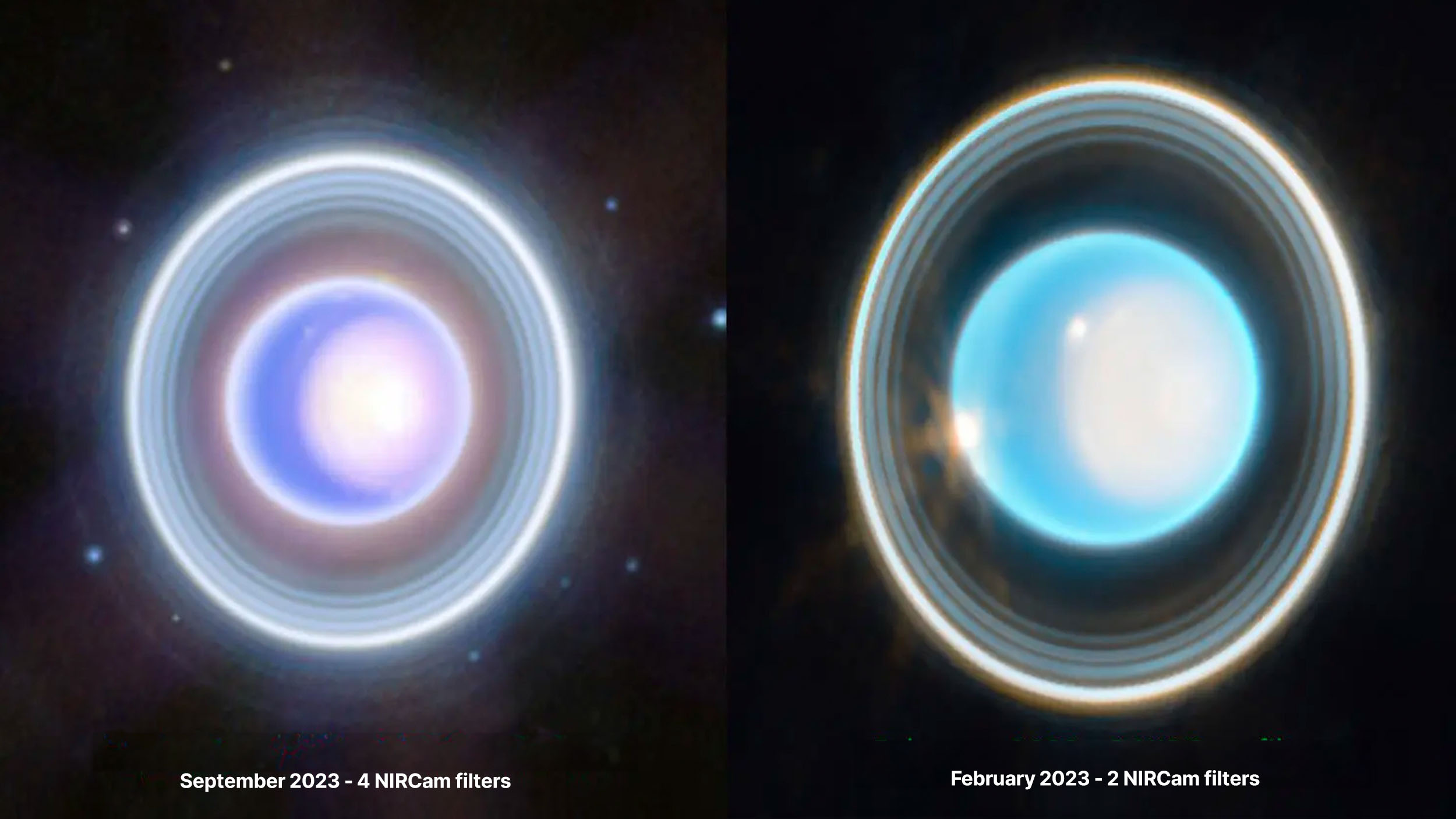
- The 7th planet in our Solar System, Uranus, was only discovered in 1781, and was only photographed up close once: when NASA’s Voyager 2 flew by it back in 1986.
- With an 84 year orbit, 1986 just happened to correspond to Uranian solstice, where one pole of this highly-tilted planet points directly toward the Sun.
- Now, as 2028 approaches, solstice is on the cusp of returning for Uranus at last. With ground-based images from Uranian equinox and JWST’s eyes to view it now, the planet comes into better view than ever before.
If you examine a planet like Earth over the course of a year, you’ll notice a great many changes. Day to day, the most significant changes will come from cloud cover and weather patterns, as the motion of storms, fronts, and water throughout Earth’s atmosphere are all variable. On longer timescales, the changing of the seasons will lead to the greening and browning of continents, the advance and retreat of glaciers, ice sheets, and polar caps. And these changes will be punctuated by singular events: geomagnetic storms, blackouts, and severe weather events at various times. All of these changes affect our planet’s appearance, dependent on when we take our particular snapshot.
But for Uranus, the story is far more dramatic. Unlike Earth, with its ~23° axial tilt, Uranus rotates almost perfectly on its side, with a ~98° axial tilt: just 8° off from perfect sideways rotation. Instead of a single calendar year, Uranus takes 84 Earth years to complete a revolution around the Sun. And this means that every 21 years, it transitions from Uranian solstice, where one pole points directly at the Sun and the other point directly away, to Uranian equinox, where each part of that world receives equal night and daylight, and then back again in the next 21 years. With its second view of Uranus, the true power of JWST for investigating this outer Solar System world has come into focus, and what we’re finding is already blowing scientists away.
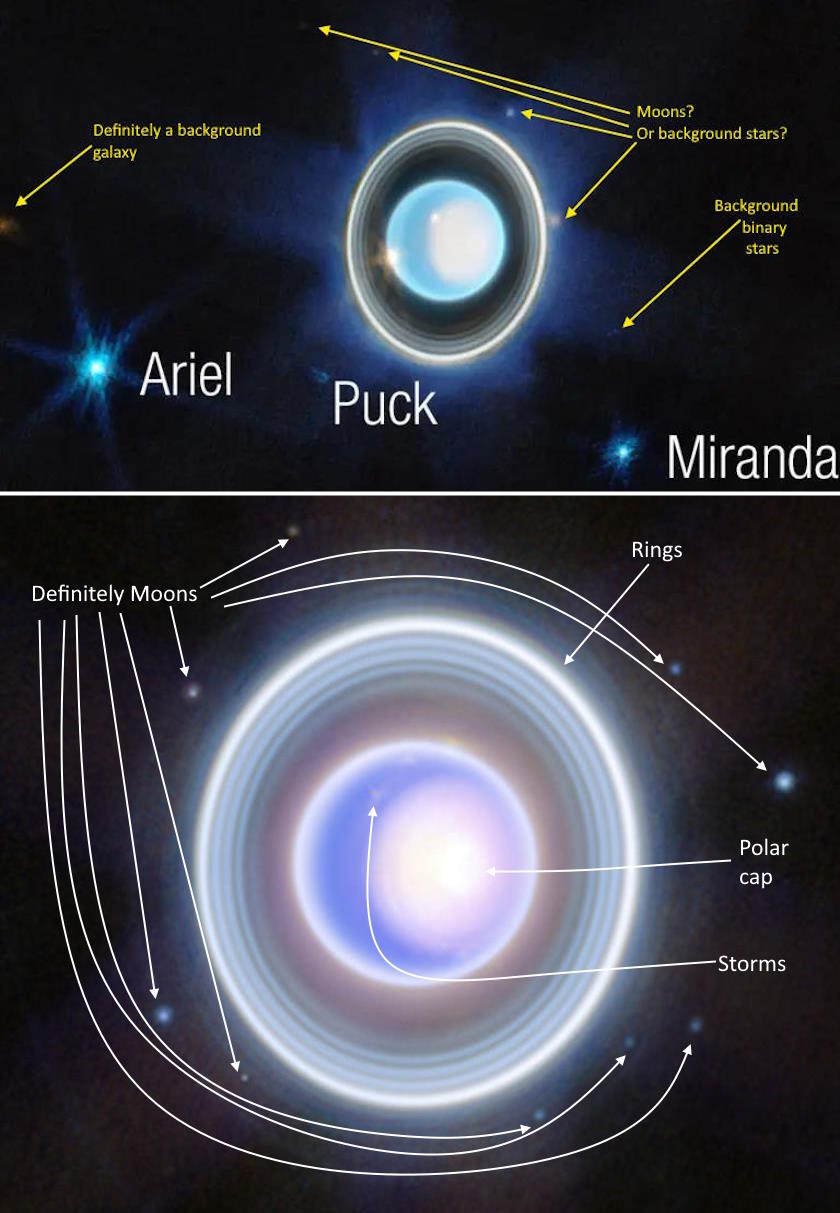
Let’s work our way from the inside out. First, in this ultra-close-up view of our 7th planet, you can clearly see the planet itself has a bright, highly reflective feature on the right side of this image. It appears to be densest in one small, roughly circular region: that is the polar cap on Uranus’s south pole. Whereas in visible light, Uranus appears as just a monochrome bluish ball at this point in time, the large amount of high-altitude ice and clouds in its atmosphere still persist, as the southern hemisphere is only now approaching its next solstice, which will arrive in 2028.
Surrounding the dense polar cap is a less dense region around it, where the polar cap still persists but is far less dense. As we look farther away from the pole and move toward equatorial latitudes, not only does the density of the cap decrease, as one might expect to find warmer regions down toward more equatorial latitudes, but dark lanes appearing toward the edge of that polar cap: evidence that the cap is evaporating as the seasons change. Finally, below the southern border of the polar cap, additional bright features — storms, likely due to a combination of seasonal and meteorological effects — can be seen at still closer-to-equatorial latitudes.
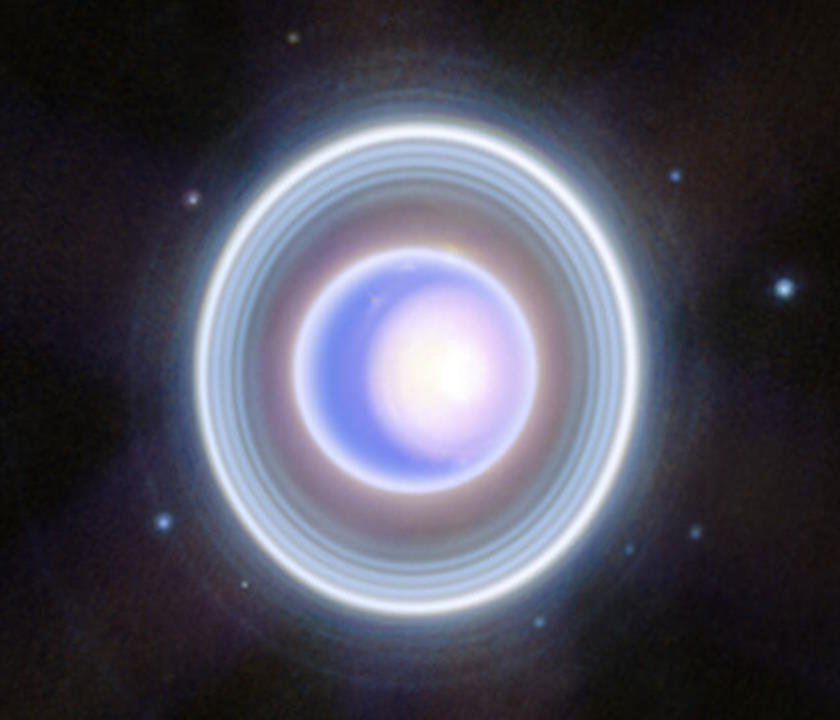
There appears to be a bright, reflective aura at the edge of Uranus, as seen by JWST’s eyes. Many have wondered at seeing this phenomenon: what is it?
Is there a ring that encircles the planet right at the top of its atmosphere, rendering invisible except where seen edge-on? That’s not quite right; observations with other instruments and up-close from Voyager 2 discount that notion.
Is there a ringed system orbiting it that’s simply located right at the upper edge of Uranus’s atmosphere, interior to the known, identified rings but identifiable to JWST’s eyes? Unlikely as well, as both Voyager 2 observations and space-based observations with Hubble, which have found previously unidentified rings around Uranus, show no evidence for such a feature.
Instead, it’s most likely to be due to an upper layer of haze: above the three layers of clouds (water-ice, ammonia, and hydrogen sulfide clouds) found at high pressures, and still above the methane cloud layers lying at higher altitudes. Instead, above the tropopause, there are likely layers of hydrocarbon haze, and where the planetary atmosphere becomes thin, those hazes are more heavily reflective, resulting in Uranus’s bright appearance to JWST’s infrared views.
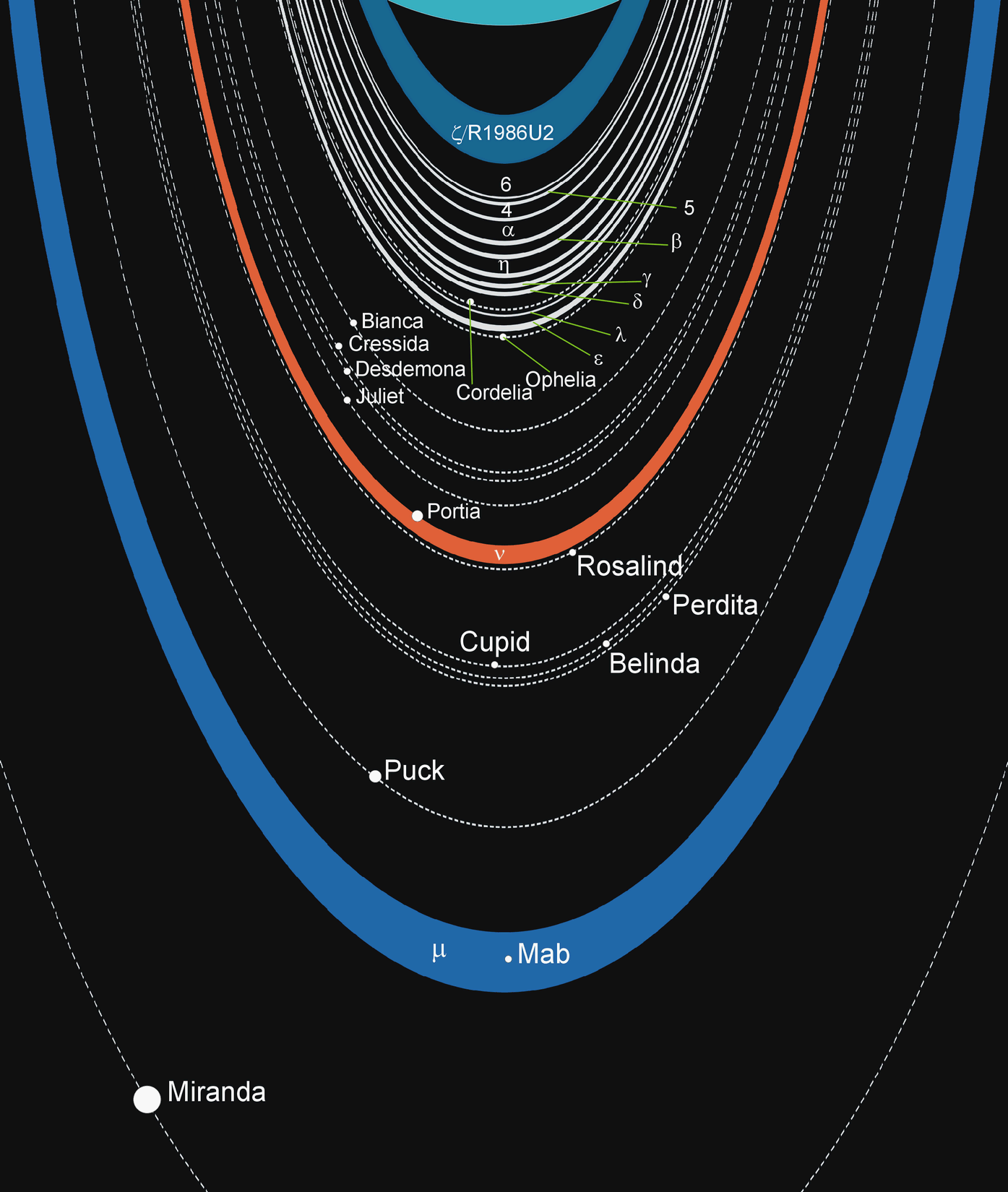
Moving farther outward, the Uranian rings shine brilliantly. The innermost ring is Uranus’s Zeta (ζ) ring: elusive to most instruments but thoroughly revealed by JWST’s NIRCam imager. Exterior to the Zeta ring are a series of additional, relatively bright rings:
- the α and β (Alpha and Beta) rings, which are closely spaced together and are both relatively broad and deep, located about 3-4000 km outside of the Zeta ring,
- the η (Eta) ring, which has a bright outer component, and is about 6000 km greater in radius (about one planet Earth radius) than the inner Zeta ring,
- the δ (Delta) ring, which has a bright inner component and is a little more than 1000 kilometers farther outward than the Eta ring,
- and the thick ε (Epsilon) ring, shepherded by Uranus’s moons Cordelia and Ophelia (not captured by JWST), which represents the thick, bright, outermost of the five clearly visible Uranian rings captured by JWST.
There are several other rings of Uranus, but beyond the Epsilon rings are what appear to be a faint series of concentric rings: these are the wider and more distant ν (Nu) and μ (Mu) rings, which are the wide, outermost, but thin and tenuous rings of Uranus, with many moons found in their vicinities.
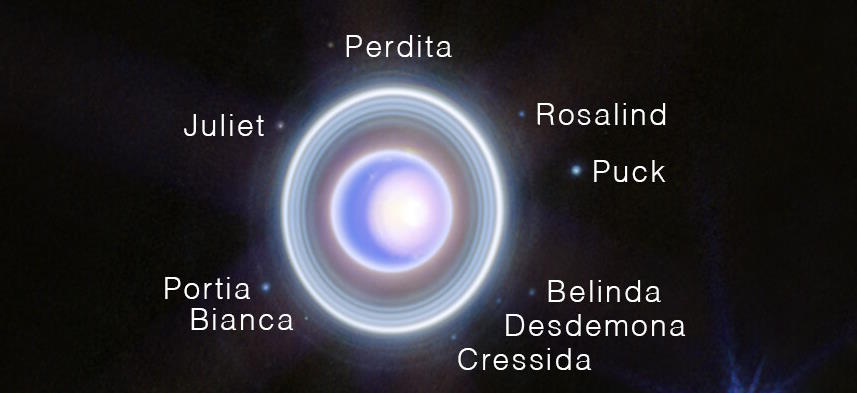
Beyond the rings, which have a few small moons that are not-quite-visible to JWST’s eyes, lie the prominent innermost moons of Uranus. These include:
- Bianca, the third-most-inner moon,
- Cressida, the fourth,
- Desdemona, the fifth,
- Juliet, the sixth,
- Portia, the seventh,
- Rosalind, the eighth,
- Belinda, the tenth (sorry, Cupid fans, it’s too small to show up here),
- Perdita, the eleventh,
- and Puck, the twelfth and largest of the inner moons of Uranus.
There’s another moon known outside of Puck, Mab, which is also too faint to be seen by JWST.
This is an incredibly impressive feat; we have known about all but three of the inner moons of Uranus since the time of Voyager 2, and JWST was able to reveal all of those except for Cordelia and Ophelia (the innermost two, likely lost in the Uranian rings). Additionally, while it was unable to find Cupid and Mab, the smallest known Uranian moons, it was able to find Perdita, the next smallest and one that was not found in Voyager 2 data. It turns out that JWST is outstanding for finding Uranus’s moons, and that’s before we even move beyond Mab: to where Uranus’s five largest and most prominent moons can be found.
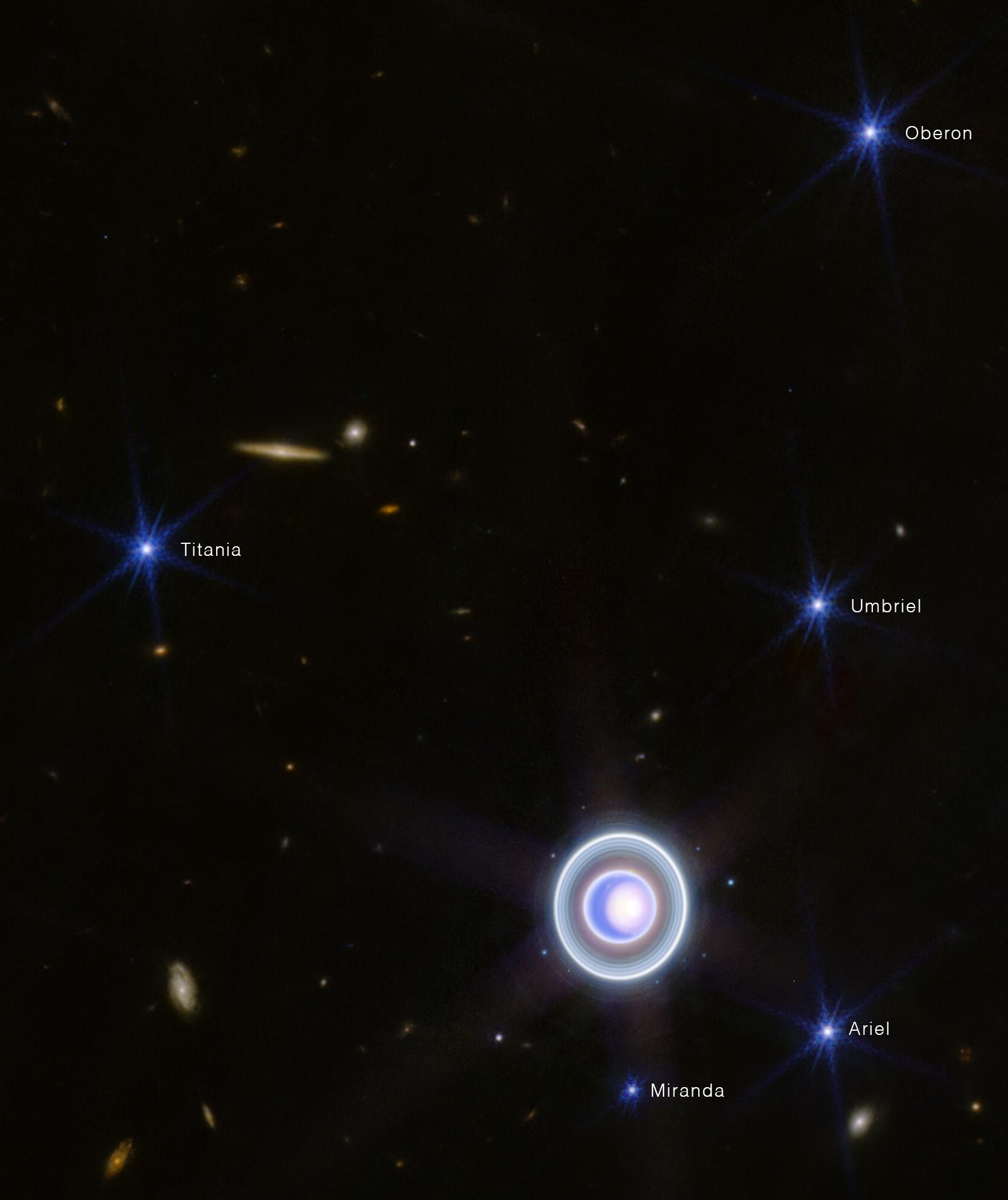
However, as we move farther outward, they spectacularly appear. The innermost of Uranus’s large moons is Miranda, which was discovered only in 1948, by a very famous astronomer whom you may better know for the belt of cometary material named after him: Gerard Kuiper. Whereas all of Uranus’s inner and the other four large moons are inclined at less than 1° to the planet’s orbital plane, Miranda is inclined at more than 4°, making it unique.
Beyond Miranda, the two larger moons Ariel and Umbriel can be located: more than 1000 km in diameter apiece. These moons were known for much longer, as both were discovered in 1851 by England’s William Lassell, who also discovered Saturn’s moon: Hyperion and Neptune’s largest moon: Triton.
And lastly, the final Uranian moons imaged by JWST are also its two largest: Titania (at 1577 km in diameter) and Oberon (at 1523 km in diameter), both discovered by William Herschel, the discoverer of Uranus itself, just 6 years after finding the Solar System’s 7th planet at all. Unlike the innermore moons that only appear as points or blobs, all five of these Uranian moons are so bright and reflective that they possess their own diffraction spikes.
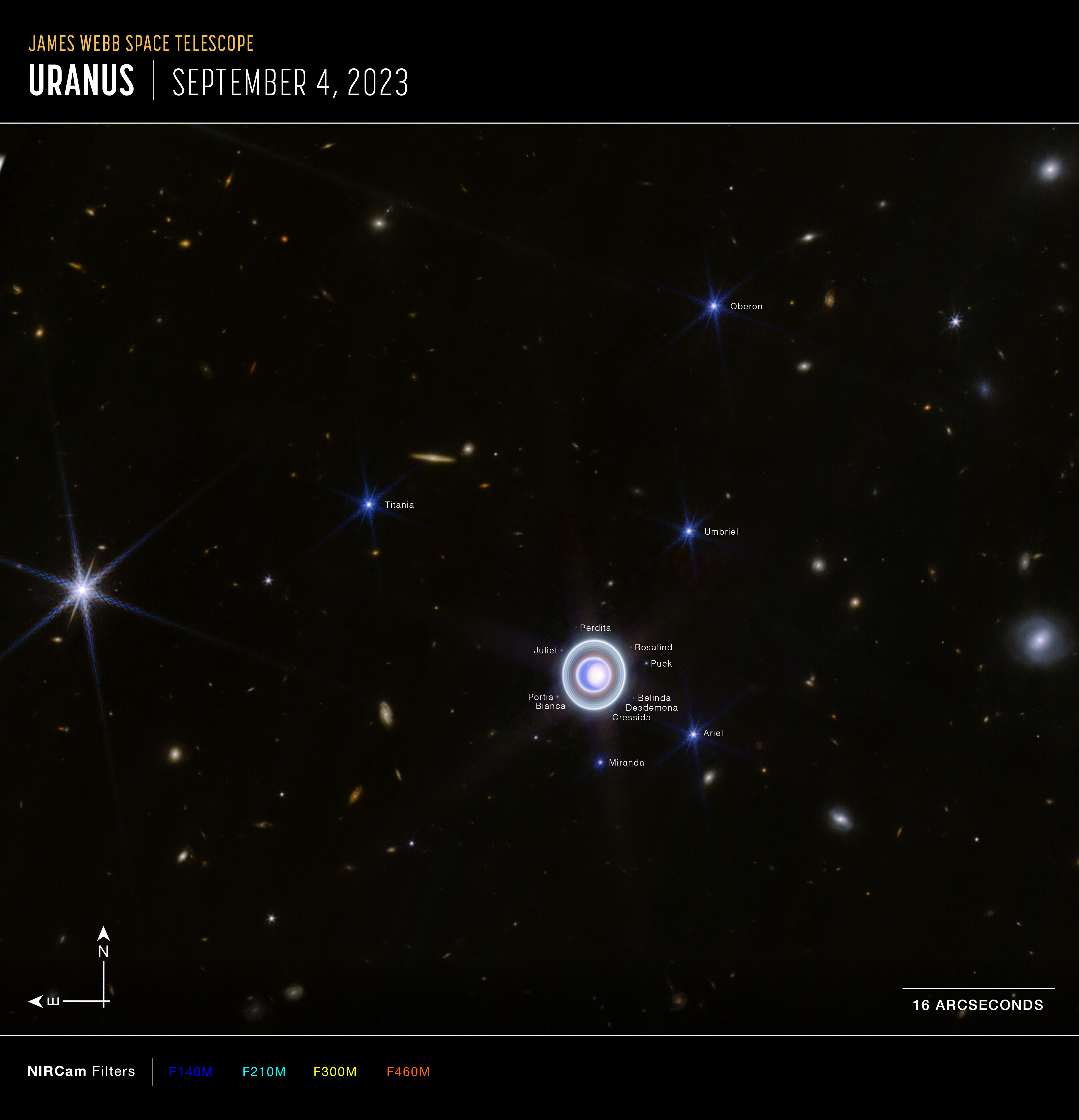
But that’s not all. In this same field of view, even though it was viewed at just a single “snapshot” in time on the date of September 4, 2023, a huge amount of additional features can be found. At the left of the image, a brighter object than Uranus or any of its moons, at least in infrared light, can be seen: that is a relatively bright star that just happens to be close by Uranus: too faint to be seen with the naked eye. To the top right of the image, a fainter star also within the Milky Way, identifiable by its diffraction spikes as well, represents the only other star in the Milky Way visible in this field.
Beyond the Solar System and the stars in our Milky Way, an enormous number of other faint points and smudges of light can be seen: these are galaxies located tens, hundreds, or even thousands of millions of light-years away. These galaxies can be found everywhere: where Uranus and its rings and moons both are and are not; the only reasons some of them are obscured is:
- because there are closer, bright, foreground objects (like Uranus, its rings, moons, or Milky Way stars) in front of them,
- or because they are too faint to be seen in this limited-time exposure, as the Uranian system’s features are bright enough to all be taken in a relatively short period of time.
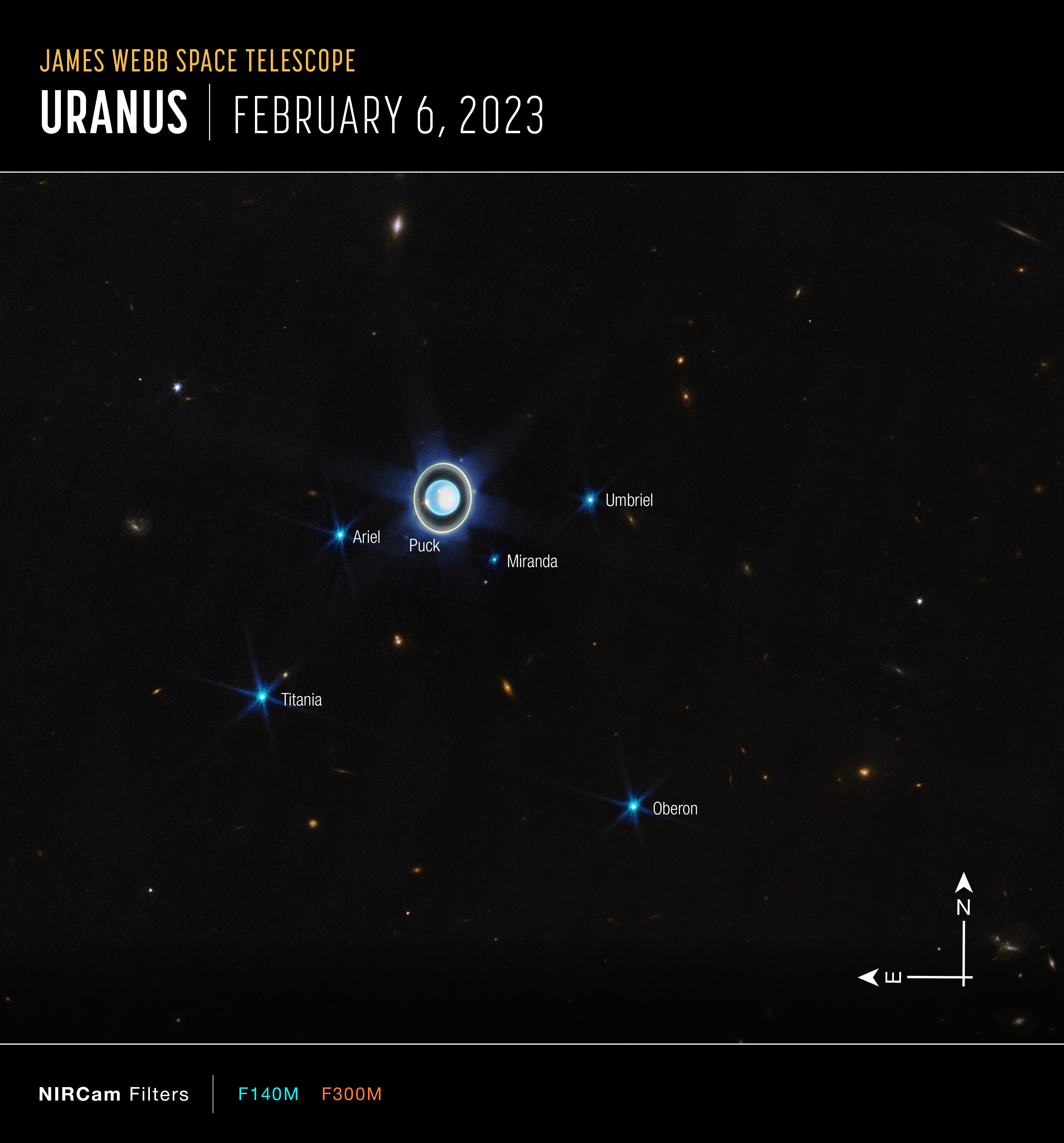
Compare that JWST view to the one above: of the same system, but taken earlier this year: on February 6, 2023, just about 7 months prior to the more recent JWST photo. While some of the features appear very similar, it’s obvious that there are:
- greater amounts of detail,
- more moons,
- fainter rings,
- and a far greater number of background galaxies,
revealed in the more recent image. Why is this?
Sure, there’s a little bit more observing time, and that definitely helps. But it’s the same instrument, on the same telescope, with the same hardware and software, viewing the same set of celestial phenomena. The big difference, however, is the addition of two new observing filters. Whereas the earlier (February) image only viewed with the 1.4 micron and 3.0 micron medium-band NIRCam filters, the later (September) image also added in data from 2.1 microns and 4.6 microns, exposing details that are either faint or invisible in those other wavelengths of light.
Just as humans have far superior color vision to dogs, because we have three (or four) types of cones compared to just two, viewing the Universe in additional bands of infrared light can significantly improve the types of details you’re sensitive to.

While Uranus is interesting in its own right, and certainly deserves a second visit now that nearly four full decades have passed since our first-and-only visit to it, there’s another important reason that JWST would want to turn its infrared eyes onto this ice giant world in our outer Solar System: exoplanets. These Uranus-size worlds are very common in the Universe, and while many of the ones we know best are relatively close to their parent stars and are therefore warm, Uranus actually possesses the coldest temperatures of any planet in our Solar System during most times of the Uranian year.
If we’re going to study exoplanets, we would be fools to not study, in great detail and with the same instruments, the “exoplanet analogues” right here in our own Solar System. How do planets of this size work? What is their meteorology like, and what types of weather phenomena appear on these planets under a variety of different conditions? By studying Uranus, especially as it makes that critical transition from equinox-to-solstice and then, afterward, back again toward the next equinox, we may learn a great amount about this planet’s atmospheric processes. And, because of that, it may help us better understand what’s going on with similarly sized (and similarly cold) planets found all throughout the Milky Way.

It also helps us prepare for the next great era in astronomy: the era of exoplanet direct imaging. In the coming years and decades, improvements in coronagraph technology, which blocks the light from a parent star but enables us to see the light coming from its orbiting planets, is expected to improve to contrasts of between one and ten billion. This means that a planet that is just one-billionth, or even one-ten billionth as bright as its parent star can be observed if the light from the parent star can be blocked, and won’t be lost in its glare. Even if the planet shows up as just a single pixel, we can learn a great deal about it, including its wind speeds, atmospheric contents, and cloud properties and variability.
What would the properties of a planet be if it possessed an extremely severe axial tilt? How does heat flow work on a planet with such extremes, and what does Uranus’s “night” side look like? Without a mission to the outer Solar System, these questions will not be answered, and it seems like these questions are of paramount importance, knowing full well the sheer extent of the variety of planets found around stars in this Universe. If we want to know more about Uranus, a mission to the outer Solar System is necessary. Until then, we can all marvel at what we’re learning just from observations with JWST!