Where do James Webb’s unique “spikes” come from?

- Since its launch in December 2021, NASA’s James Webb Space Telescope has successfully reached and surpassed a number of important milestones in launch, arrival, and configuration.
- The mirrors and instruments are being cooled and calibrated over the telescope’s first six months in space in preparation for science operations, and it’s either right on or even ahead of schedule for everything.
- But when you look at the first aligned image, you might notice an unusual pattern of spikes coming from their very first star. Here’s why at least six of those spikes will always persist.
Less than three months after its initial launch, it finally happened: the James Webb Space Telescope revealed its first telescope alignment evaluation image. For the first time, humanity’s latest, greatest flagship observatory has successfully aligned, focused, and finely phased the light from all 18 of its primary mirror segments to successfully produce a single image of a “test star.” The results are so good that even in this preliminary image, faint stars and galaxies located in the distant background can also be seen, resolved, and even examined in detail. It’s a remarkable triumph, and as NASA themselves announced, “Every optical parameter that has been checked and tested is performing at, or above, expectations.”
Still, what you’ll notice, even on a cursory glance at the image, is that the main “star” imaged has a series of spikes coming out of it: six large ones and two smaller ones, all visible even zoomed out and at low resolution. For comparison, the Hubble Space Telescope only had four spikes attached to every star, and an upcoming 25-meter ground-based telescope, the Giant Magellan Telescope, will be the first observatory of its kind to have no spikes on its stars at all. Here’s what we can expect, as far as spikes go, from the James Webb Space Telescope, even at full, maximum power.
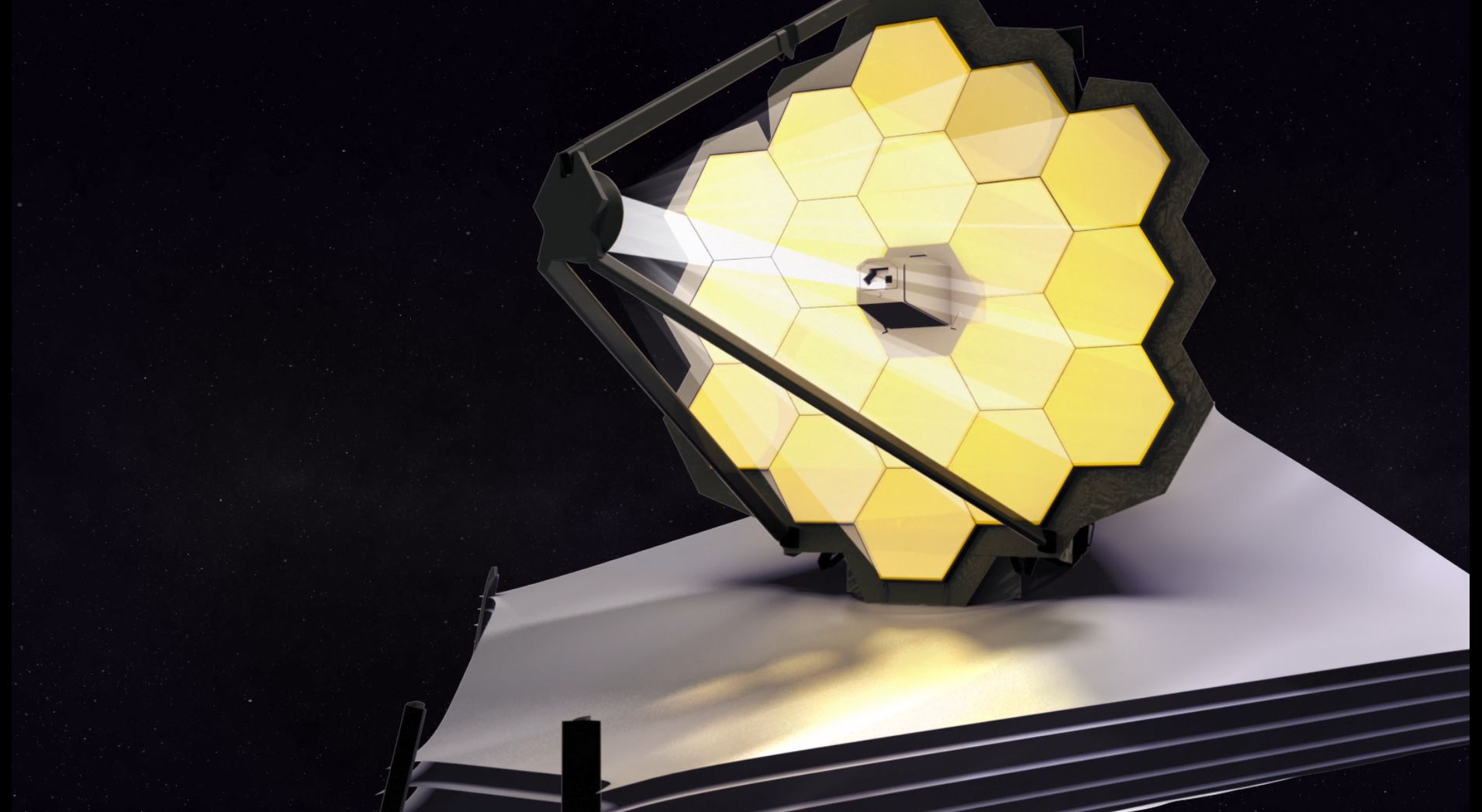
The first thing you have to realize about the James Webb Space Telescope is that, unlike Hubble, it is not a single-dish mirror. Instead, there are 18 individual segments to its primary mirror, and the goal of an optimally-configured James Webb will be to have all 18 of those segments function as though they are a single mirror, in a single plane, with optical perfection.
What does “optical perfection” mean in this instance?
It means that each one of the 18 segments will make up a section of a perfect mirror, all designed to have the cumulative light striking it from a distant, observed target focus down to a single point precisely in the telescope’s instruments. This is a tremendously ambitious task, requiring that we compensate for:
- the spacing in between each segment,
- the edges, and in particular, the sharp corners, of each segment,
- the optical imperfections induced by the trusses that hold the secondary mirror in front of the primary mirror,
- and the individual variations both across each segment and from segment-to-segment.
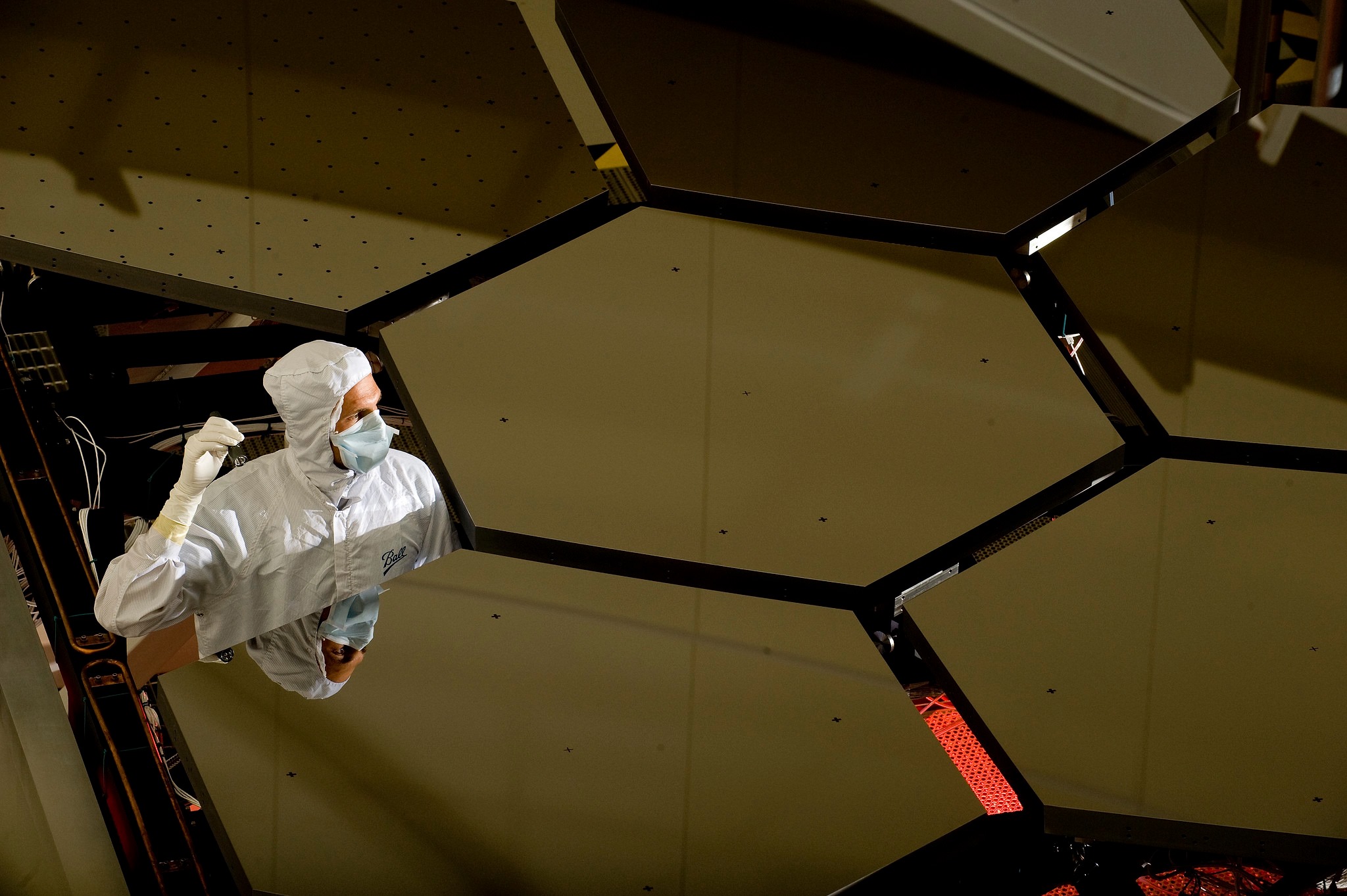
Each one of the individual segments themselves, back at various points in the 20th century, would have made a spectacular, cutting-edge observatory all by itself. If you want to focus your light properly, you need to avoid the problem that initially plagued the Hubble Space Telescope: spherical aberration. Spherical shapes, for both lenses and mirrors, are easier to manufacture than other curves, like parabolas, and the shapes of large lenses and mirrors can be distorted by gravity.
As a result, when Webb, which was built on Earth but operates in space, was launched, there was a worry that everything might not fall properly into place. Even though the mirrors were checked and re-checked and re-re-checked again and again, there was always the worry that something would be off with the optics. If that was the case, then the mirrors would be unable to focus the distant starlight into the single image that was desired, and we’d have to find some way to compensate for the blurriness that would arise.

For the 18 mirror segments that James Webb possesses, there are three individual designs that needed to be manufactured six times each:
- the “A” segments, which are for the interior segments, where five of the six hexagonal edges will border another mirror segment, but the innermost one will leave a gap for light to be reflected inside, onto the instruments,
- the “B” segments, which are at the outside corners of the hexagon-shaped honeycomb, each have three edges that border another mirror segment, but three edges that comprise the outside border of the primary mirror,
- and the “C” segments, which go between the “B” segments and possess four edges that border another mirror segment, but two edges that, along with the “B” segments, define the outside border of the primary mirror.
As a result, the shape of the James Webb Space Telescope’s primary mirror makes a shape known as a tricontagon, or a 30-sided polygon. This is a very, very complicated geometrical shape to deal with, and the technical achievements needed to produce a quality data product are literally astronomical.
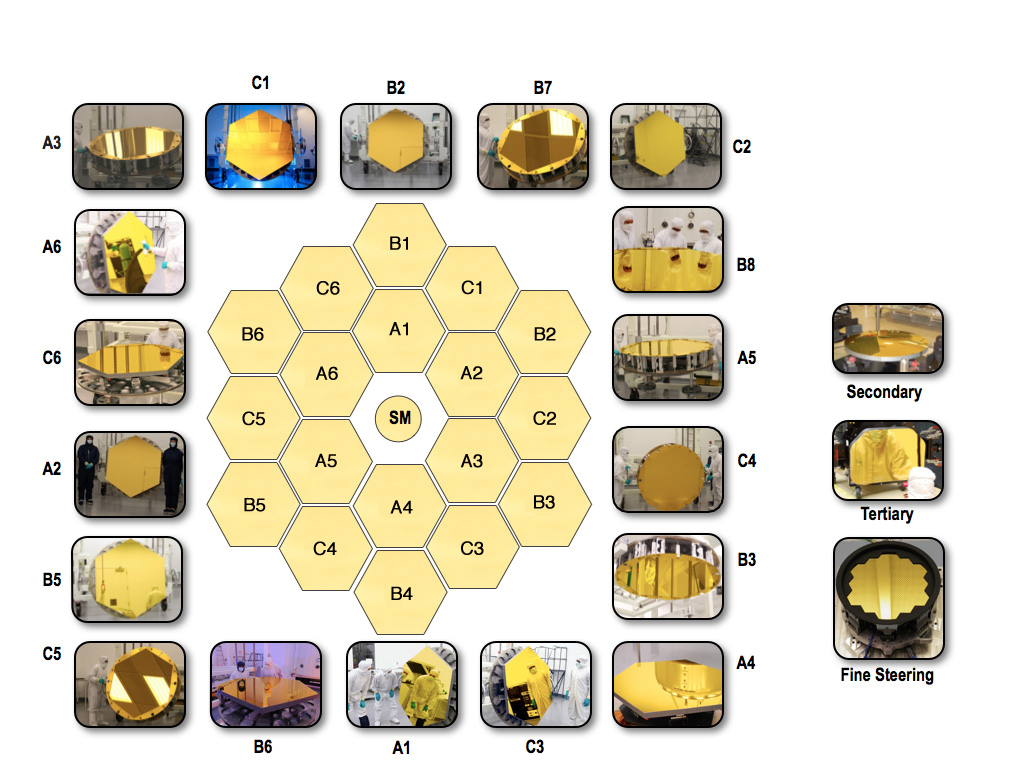
In its unfocused state, the James Webb Space Telescope would simply be made up of 18 individual mirrors, each with their own shape, their own plane of focus, and each one would produce their own image for whatever object we were attempting to observe.
The goal is to have each of these 18 segments form a single plane, together, that has a parabolic shape. At some 6.5 meters (around 21 feet) across, the variations in the plane, both across each segment and from segment-to-segment, should be right around ~20 nanometers for optimal performance. That’s an incredible precision, by the way; if the surface of the entire Earth were as smooth as Webb’s precision needs to be for its optics, then the highest mountain and the deepest ocean trench would only depart from sea level by about 2 centimeters (less than one inch), total.
When Webb took its very first image of a star, attempting to see what sort of image the 18 segments produced, it was clear that a lot of work remained ahead of the team.
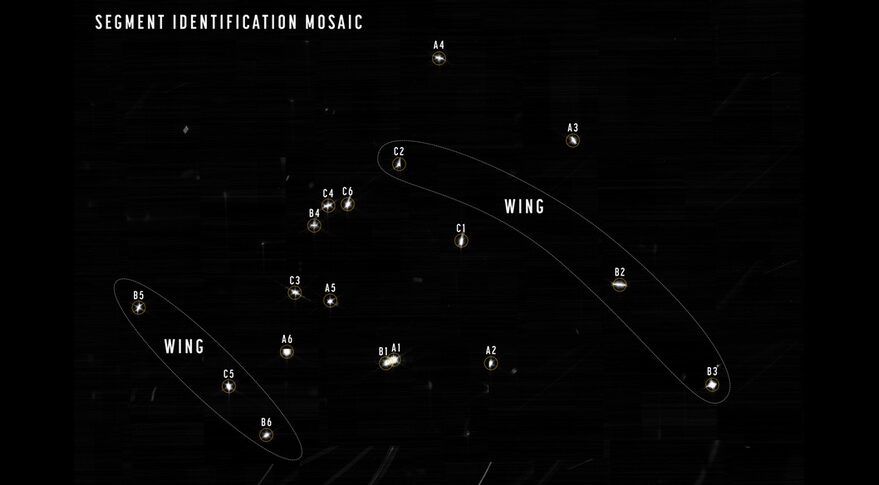
You’ll notice, when you view the initial (above) image, you see 18 different sources of light: one corresponding to each segment of the primary mirror. You’ll also notice that these sources appear all over the place, rather than in the desired “honeycomb” configuration that the mirror segments themselves take on. Finally, you’ll notice that each one of these sources doesn’t correspond to a single point-like source, which is what you’d expect for a star, but that each one appears distorted and spread out over a volume of space.
When you take a true point source of light and image it through any sort of optical system, you’re not going to get a “point” back again. Instead, you’ll get a shape unique to your equipment, which can be described by a mathematical equation known as a point-spread function. We can know that we’re looking at a star, and that the star ought to appear as a single point of light, but that’s not what we see. For James Webb, with its 18 hexagonal segments configured to make a tricontagon overall, it results in an incredibly complicated point-spread function that astronomers working on the telescope simply call, “the nightmare snowflake.”
Even with the substantial progress that was made to focus and align the individual mirror segments into a single plane, and then to combine those 18 individual images into a single one that best represents the true point source we’re observing, the “nightmare snowflake” clearly rears its ugly head.
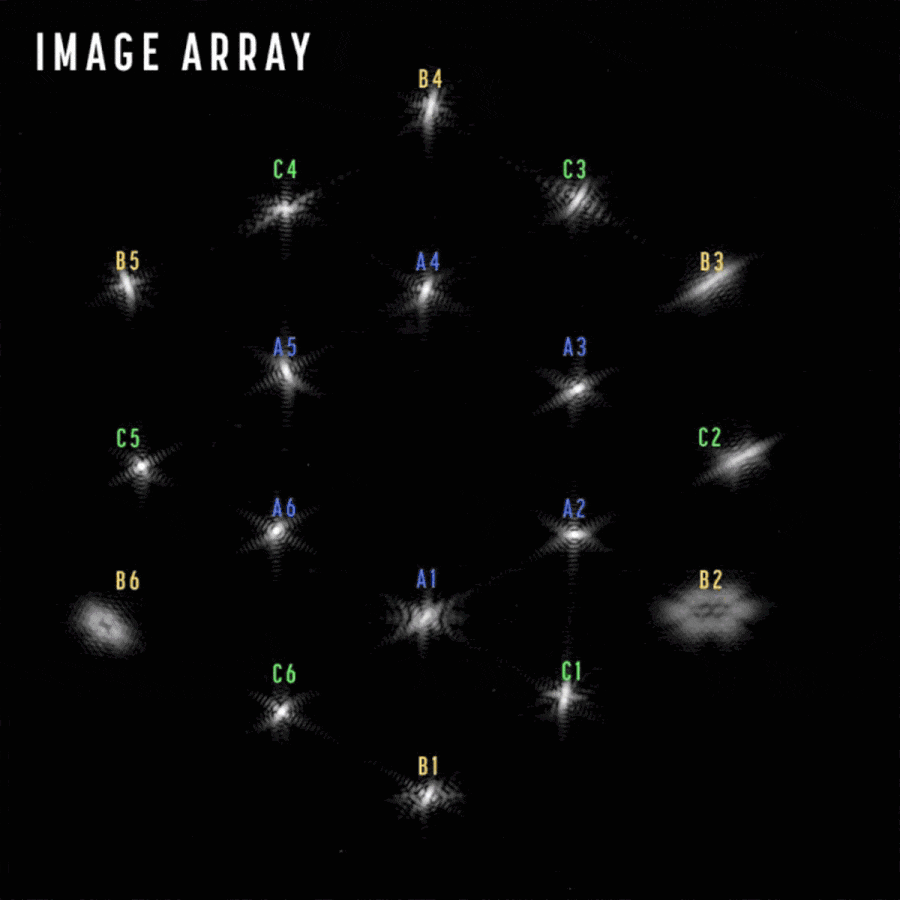
The individual star you see at the end, above, represents what happens when all of the mirrors are focused and phased together. But everywhere you have an edge, a gap, or something that blocks a portion of the light from coming into your primary mirror, you’re going to get an image artifact, and that’s something we must be able to successfully correct for.
That involves tweaking the shape of each individual mirror through the use of actuators, and doing so in a wavelength-dependent way. It involves ensuring that each individual mirror not only makes its own perfectly parabolic shape, but that the shapes between mirrors all correspond to a different portion of the same parabola. And, even with gaps that are approximately ~4 millimeters between the individual mirror segments, that one single parabola needs to be perfect down to a tolerance of ~20 nanometers.
Even at that, there are still the edges to reckon with, and the fact that there are supports for holding the secondary mirror in place that cross the plane of the primary mirror.
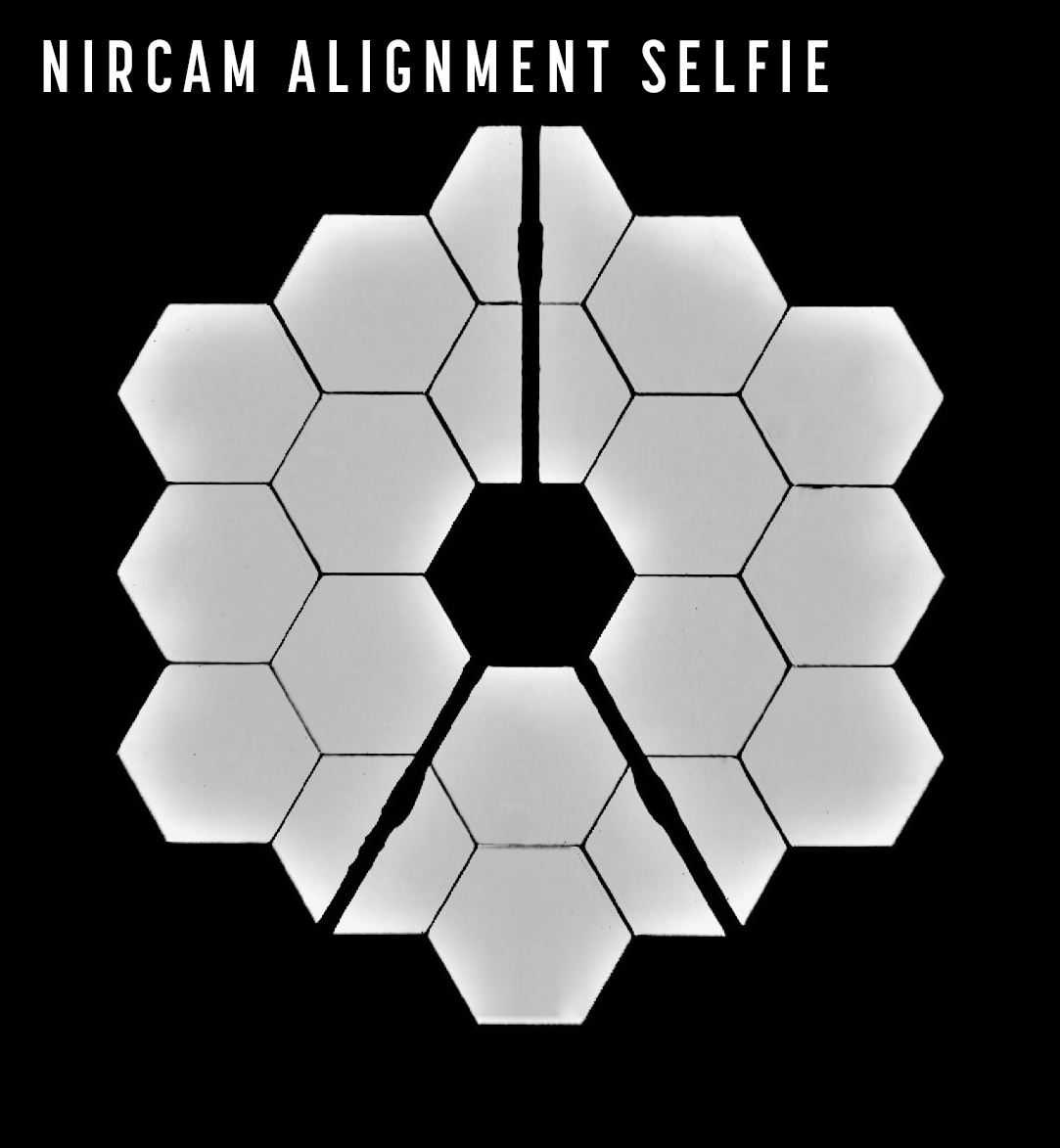
In the case of James Webb, specifically, there are three axes that the supports rest on, and they cause three sets of inevitable spikes that will always appear in images of point sources, such as stars.
This isn’t unique to the James Webb Space Telescope, by the way. Any reflecting telescope where the light is reflected back in front of the telescope and into a secondary mirror, where the secondary mirror then reflects the light into a “hole” located in the primary mirror itself (and then, into the instruments), needs that secondary mirror held in place in front of the primary mirror by something.
However those supports are configured determines the shape of the spikes, with each unique support creating its own diffraction spikes that are perpendicular to that support structure itself.
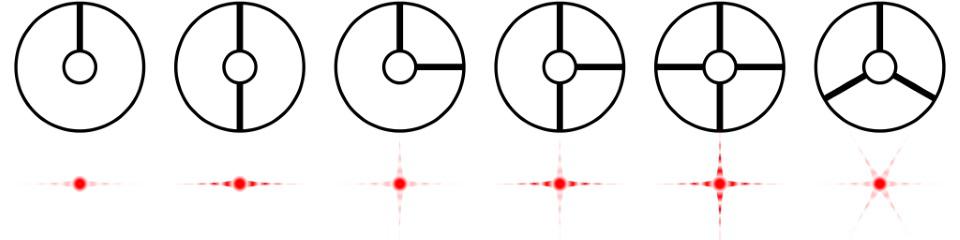
The Hubble Space Telescope, whose images are arguably the most ubiquitous and recognizable of all telescopes in human history, is configured in a typical way for a reflecting telescope: with supports for the secondary mirror shaped like the “+” sign. Its perpendicular supports ensure that there will be heavy diffraction spikes making a “+” shape coming off of every source that qualifies as point-like: the individual stars it can see.
Other sources, however, such as distant galaxies and nebulae, are what we know of as extended sources, as their light is spread out over a larger area on the sky. As a result, these spikes are non-existent, since light arrives from more than just a point, and that optical effect is effectively washed out over the large angular area that the extended object provides. In the (Hubble) image below, for example, you can easily identify the points of light that are stars contained within our own Milky Way by their diffraction spikes, whereas the fainter, more distant, extended objects definitively do not possess them.
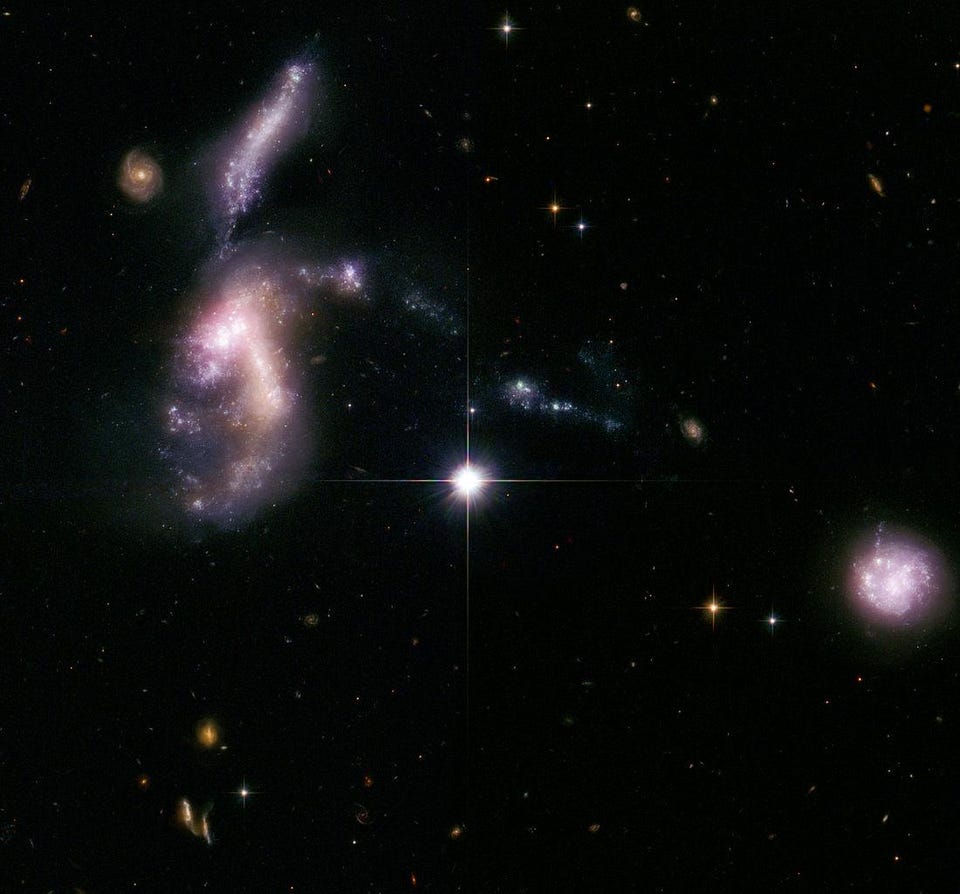
As the fine phasing of the James Webb Space Telescope continues, we’ll continue to see the “nightmare snowflake” evolve closer and closer toward its desired shape: of simply six spikes coming off of every star, and of the more distant, extended objects looking more and more pristine.
On March 11, 2022, the James Webb Space Telescope team at NASA produced what’s been labeled as a Telescope Alignment Evaluation Image, and the results are immediately apparent as spectacular. Instead of a snowflake with all sorts of artifacts emanating from it, and instead of an extended point where the light is clearly spread out over a large area, the star itself looks crisp, collimated, and has six major diffraction spikes in the expected directions. Taken only with the NIRCam instrument, it’s already well-enough aligned to reveal background stars and galaxies, with many of the background stars displaying their own diffraction spikes as well.
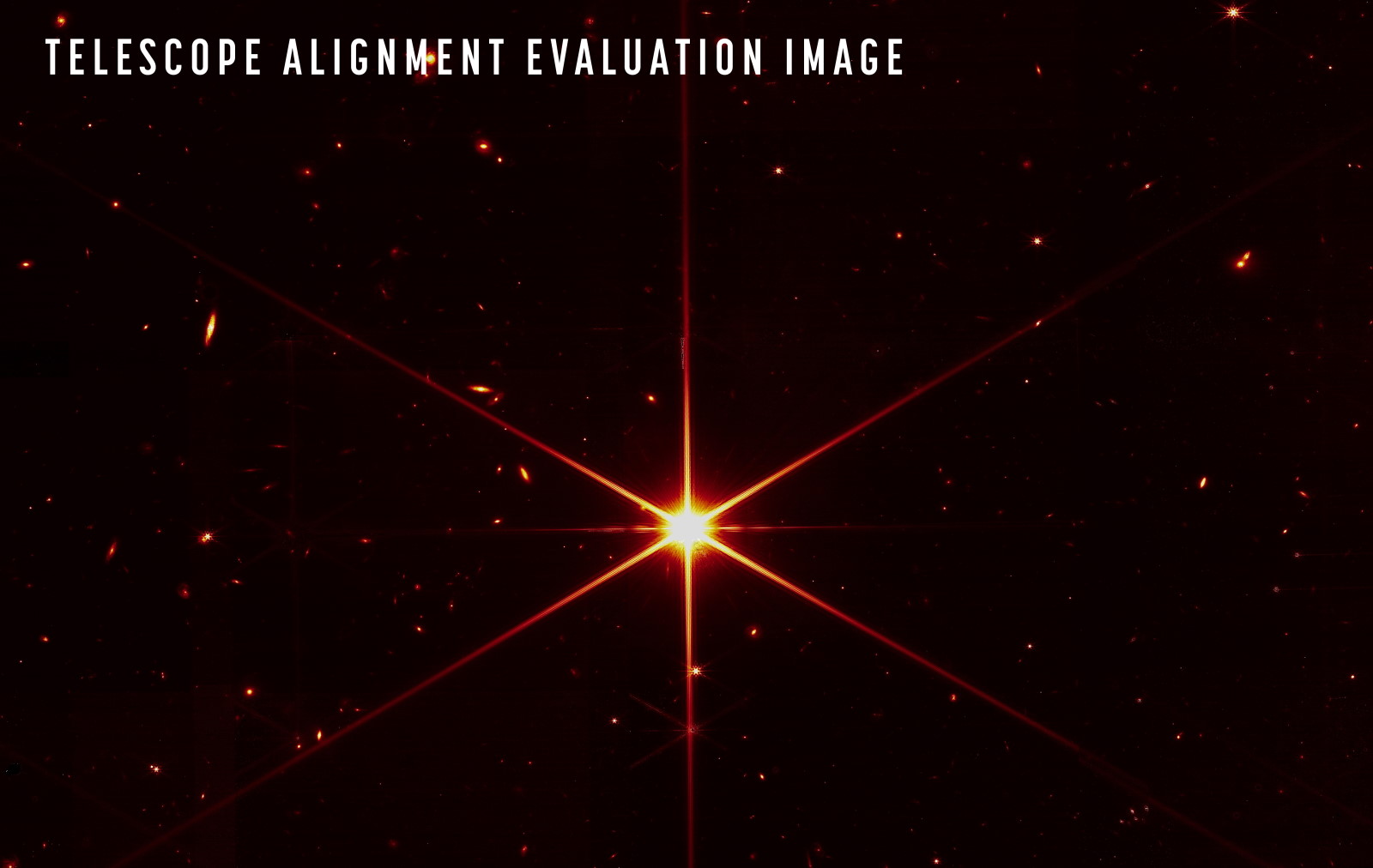
This isn’t, however, “as good as it gets” for James Webb. If you look at this with a very careful eye and pay attention to details, you’ll notice many things about this star and the rays that come off of it. For example:
- Each of the six major diffraction spikes has a set of perhaps five-to-seven major streaks, rather than all being aligned into a single spike.
- Between the spikes, there are smaller rays of light that come off and prevent us from viewing objects that are too close to the luminous star.
- In the horizontal plane, where there should be no diffraction spikes, we have an extra, fainter, but still substantial set of spikes: a 7th and 8th spike, both of which should be eliminated.
- And that if you examine the other stars or galaxies revealed in the image, you can see that they’re not pristine either, but rather have distortions consistent with Webb not yet being optimally aligned and configured.
In the image below, I’ve highlighted some of the details that the team will work to improve over the coming months. Even though progress has been spectacular over the first three months of Webb’s commissioning, you should be heartened to know that there are still a few months before science operations begin, giving the team an opportunity to iron out as many of these details as possible before we start using the observatory’s capabilities to teach us as much as possible about the Universe.
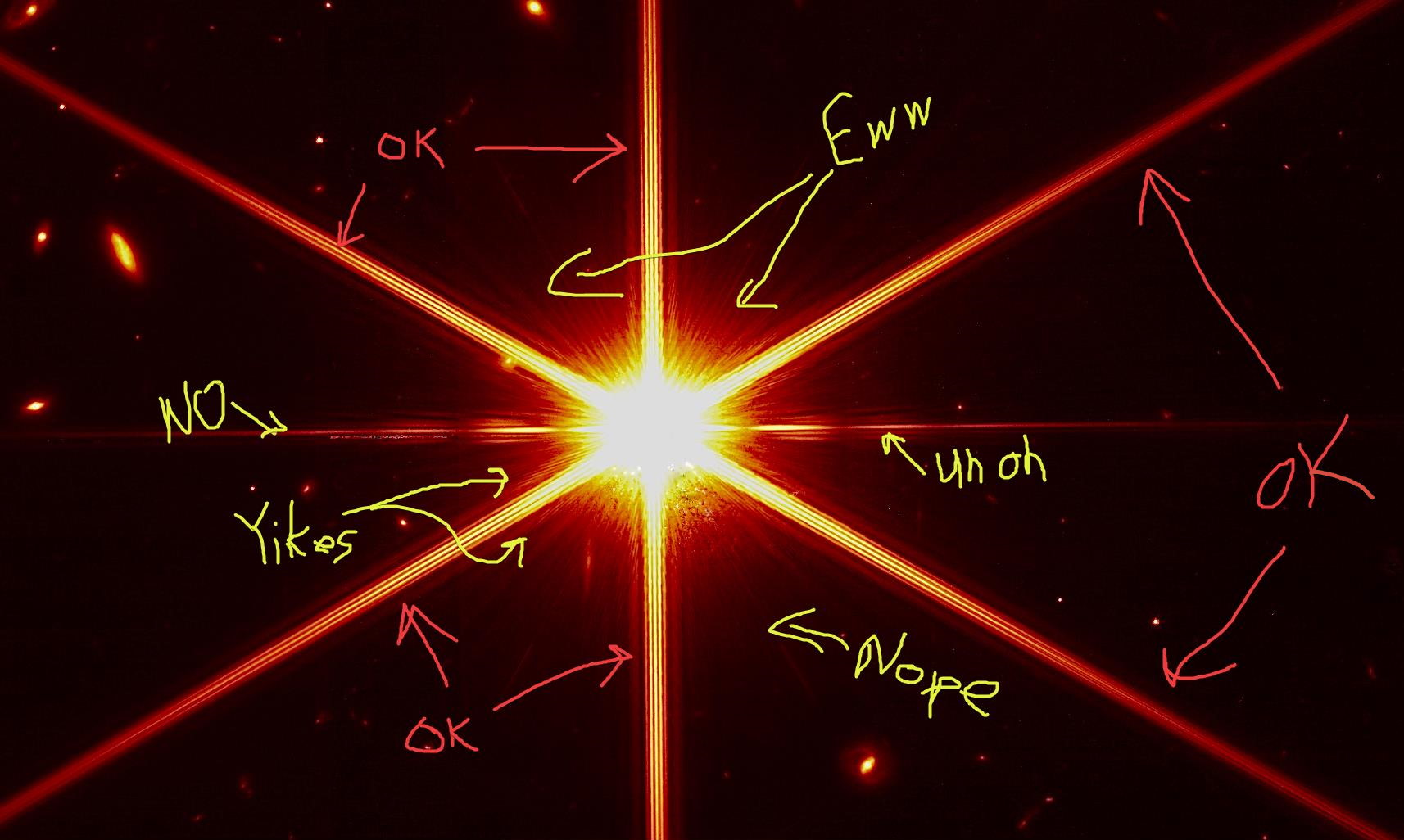
Update (3/23/2022): One of the fun things that’s available, historically, is the original prediction of what the James Webb Space Telescope should see when it looks at a very bright point source. (For reference, this “calibration star” seen in the above image is much brighter than anything that Webb will ever observe on purpose.) Way back in 2007, four scientists working on a technical report for the James Webb Space Telescope calculated the expected effects of Webb’s unique configuration on the point-spread function of a bright star that was observed with the telescope.
As you can see, from the image below, there are four major factors that create the point-spread function of the JWST. They are:
- the fact that the overall shape of the mirror is hexagonal, rather than circular,
- the fact that it’s not a single, solid mirror, but rather is a series of 18 tiled hexagons,
- the fact that there are small (about ~4 mm) gaps between each of the hexagonal tiles,
- and then, finally, the fact that the support struts exist to hold the secondary mirror in place.
It looks like, based on this analysis, that the only way to remove the six large (plus two smaller) diffraction spikes will be with software, after-the-fact.
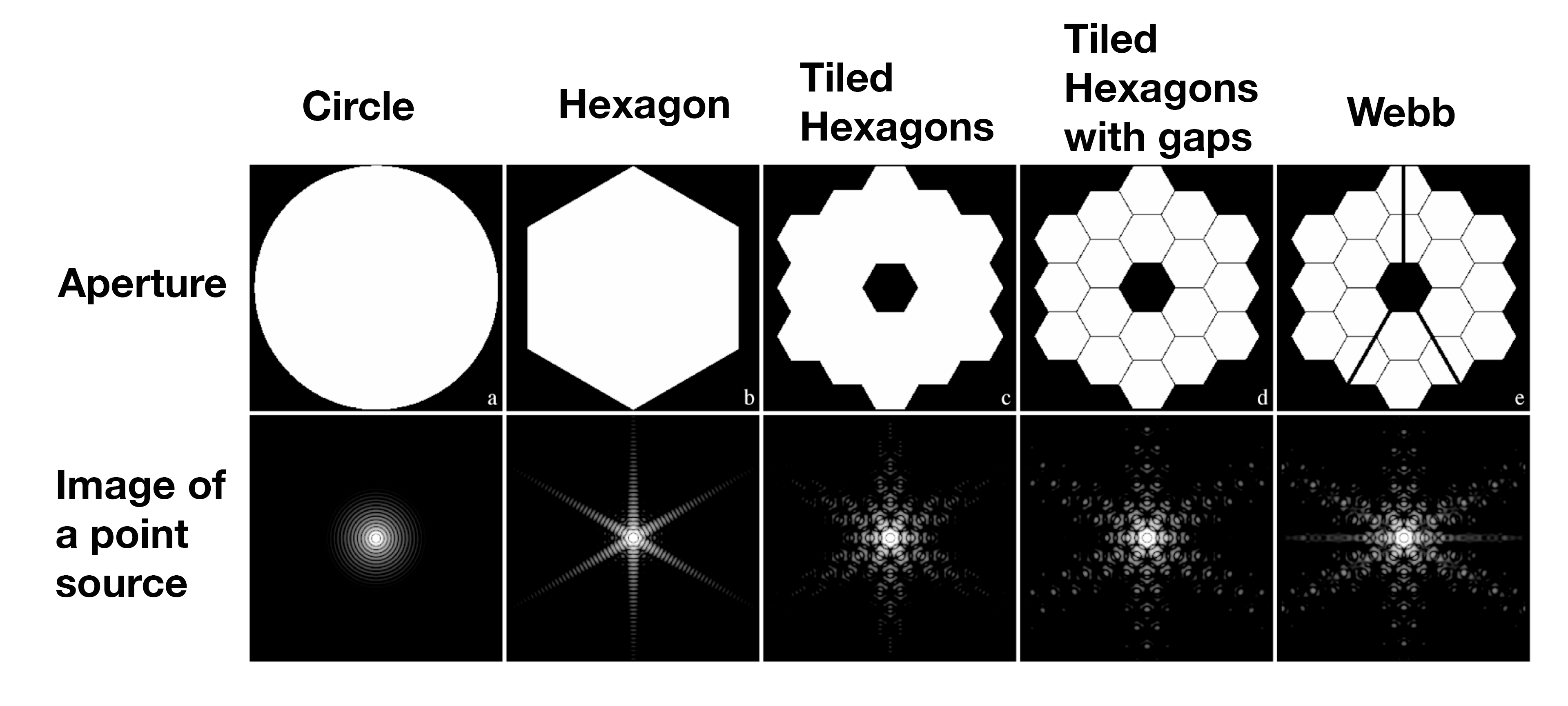
However, you shouldn’t assume that every telescope, or even every reflecting telescope, will always be stuck with this “diffraction spike” problem. Right now, on the first sets of images we’re seeing from James Webb, there are many more spikes and features than we should see when calibration is complete. At that point, there should be only the six major spikes and nothing else; the additional features should be absolutely minimized. The only reason a star should appear larger than a single point, excepting the spikes, should be if it’s bright enough to saturate the detector itself.
Moreover, there’s already a world-class telescope under construction that should be the first of its kind to produce images without any diffraction spikes. The Giant Magellan Telescope, slated for completion perhaps toward the end of the 2020s, is going to be approximately 25 meters in diameter, making it the second-largest optical telescope in the world behind the (also under construction) 39 meter European Extremely Large Telescope. But unlike its larger counterpart, which, like Webb, will be made up of large numbers of hexagonal segments tiled together, the Giant Magellan Telescope will only be made up of seven large, circular mirrors, all installed upon the same telescope mount.
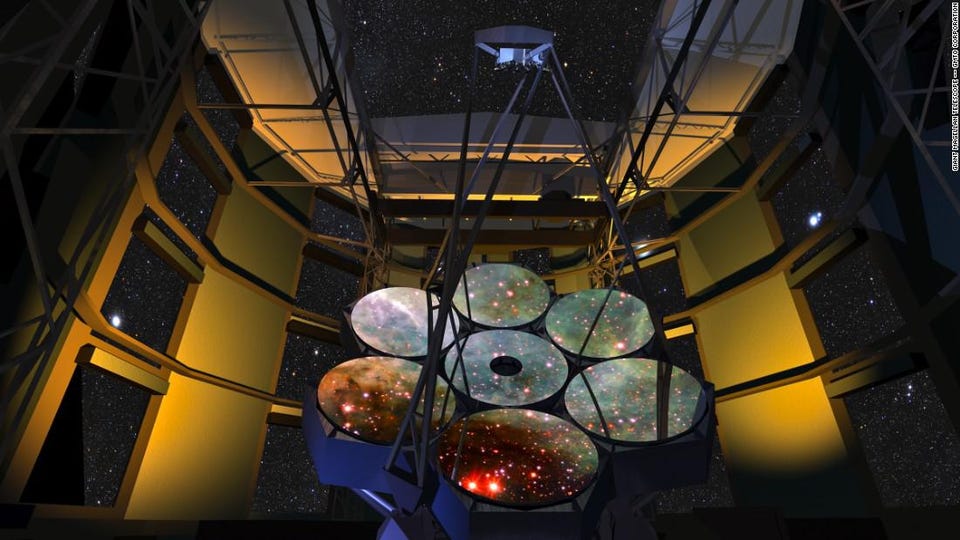
As a result of the Giant Magellan Telescope’s unique configuration, the three support struts that will hold the secondary mirrors in place will exist in the gaps between the primary mirror segments; they will not obstruct the light that reaches and reflects off of the telescope mirrors at all! Although there will be other image artifacts that arise, in particular a set of circular beads that appear along ring-like paths (Airy rings), simply observing the same object for about 15 minutes or longer will fill those beads in, creating our first cutting-edge images of stars using a reflecting telescope without any diffraction spikes at all.
The six spikes coming off of James Webb’s best configuration image to date will improve and narrow with time, and the remaining spikes and image artifacts should be improved upon in the coming months. Although Webb has often been called the successor to Hubble, it will be observing primarily in the infrared: the same sets of wavelengths previously viewed by another six-spiked space observatory: NASA’s Spitzer. Sure, Webb will always possess these spikes, but thanks to clever engineering, there will be other telescopes that won’t have them at all. We’re going to be getting not only a whole new view of the Universe, but an entirely novel experience in visualizing it. With each passing day, the potential of science and discovery with James Webb only gets more and more exciting.