Ask Ethan: Can black holes really cause dark energy?
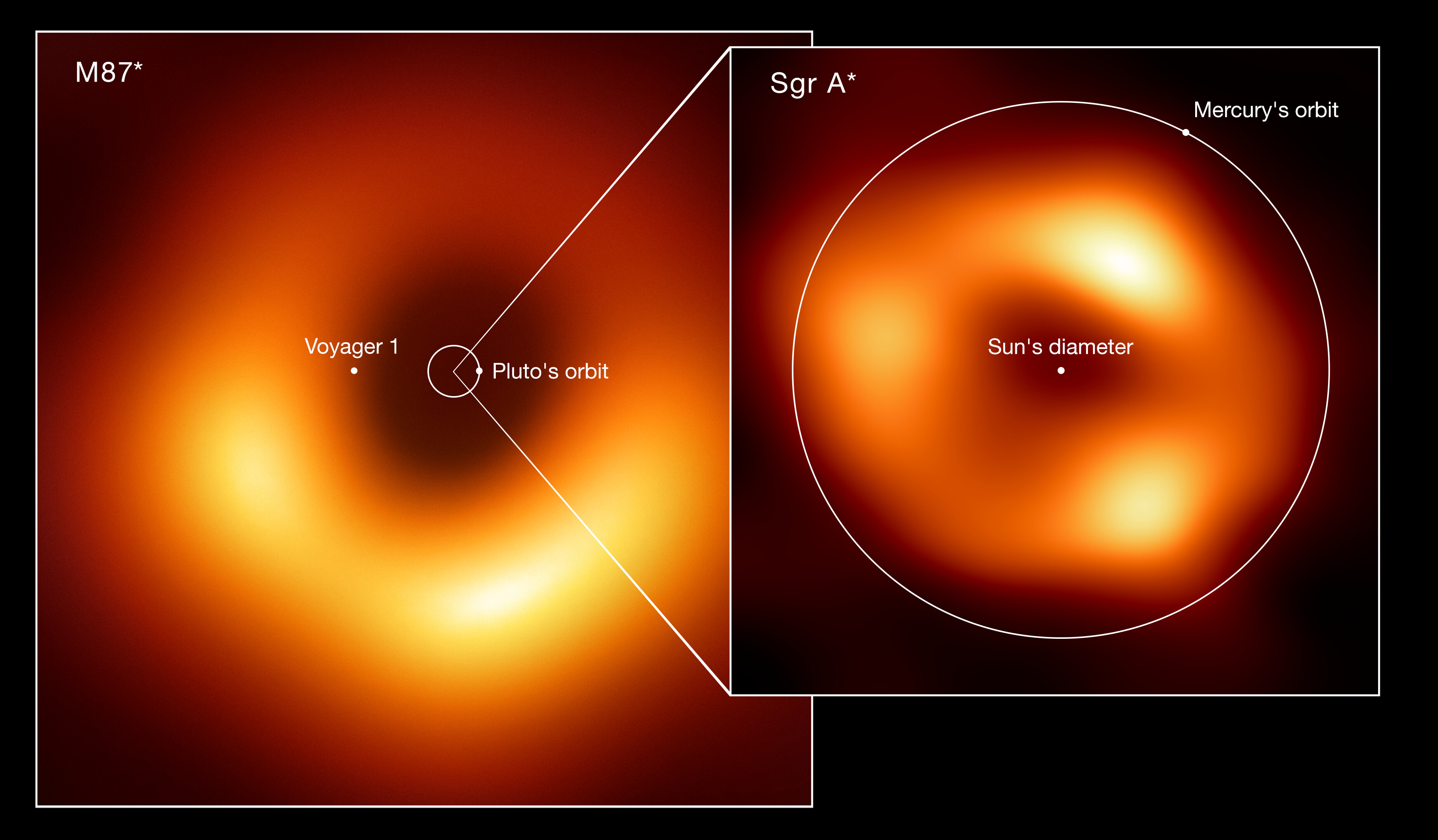
- One of the greatest mysteries in the Universe is that of the accelerated expansion of the cosmos, often described as an unknown form of energy dubbed “dark energy.”
- While many potential explanations have been offered for why dark energy exists, no one has yet been able to calculate its value, or offer a compelling reason for why it possesses the value it does.
- In a brand new study put forth in February of 2023, a team of scientists put forth the idea, backed by some very suggestive evidence, that black holes might be the culprit. How does the idea stack up?
Despite all we’ve learned about the Universe over the 20th and 21st centuries, a few important phenomena have yet to be sufficiently explained. We know there’s more matter than antimatter in the Universe, but we don’t know how this cosmic asymmetry came to be. We know that for every gram of matter in the Universe, there are ~5 grams of dark matter, but we don’t know what dark matter is or what its properties are. And we know that the Universe’s expansion is accelerating, but we don’t understand what’s causing that phenomenon. We’ve given it a name — dark energy — but lack an understanding of why it exists at all, and how it came to have the value it possesses today.
In a fascinating new study that’s taking the internet by storm, a team of scientists believe they’ve found a connection between the interiors of supermassive black holes and the dark energy that permeates the Universe. Already, five separate people — Jeremy Parker, Cameron Sowards, Dario Gnani, Jeremy Forsythe, and Patreon supporter Pedro Teixeira — have written in to ask about it, stating:
“Help!”
“Is there any validity to this theory?”
“[N]eed your take on this for sure!”
“[T]his sounds amazing (kind of next Nobel prize stuff) and would love for you to weigh in on the matter.”
If you’re here, you’re in the right place for a deep dive. Let’s start with the basics and go from there!
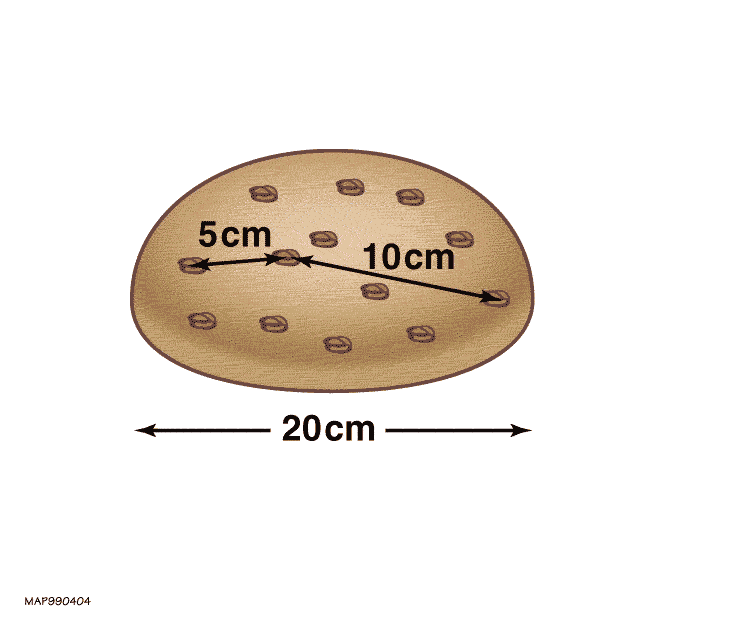
For about a full century now, ever since the 1923 observations that allowed us to first measure the distances to galaxies beyond the Milky Way, we’ve noticed an important relationship: the farther away a galaxy is from us, the faster it appears to be receding from us. When we made the connection of these observations to Einstein’s General Relativity, we discovered that the Universe was expanding. It was like a great cosmic race was taking place — between the initial expansion driving everything apart and the gravitational effects of all the matter and energy in the Universe attempting to pull everything back together — where the Big Bang was the starting gun.
For many generations, physicists and astronomers considered three main possibilities for how this race would end.
- A Big Crunch. After a period of rapid expansion, there could be enough matter-and-energy in the Universe to cause the expansion to slow, stop, reverse direction, and recollapse, ending in a Big Crunch.
- A Big Freeze. In this scenario, the Universe begins expanding rapidly, but now there isn’t enough matter-and-energy to cause the expansion to stop and reverse. Instead, it expands forever, with all the matter structures within the Universe eventually coasting away from all the others.
- A “Goldilocks” ending. Or, quite possibly, the Universe comes perfectly balanced between the two: where the Universe would recollapse if there were a single extra atom in it, but instead, the expansion rate only approaches zero, never quite stopping or reversing.
And yet, when the definitive data arrived in the 1990s, it indicated that the Universe was actually doing none of these.

Instead, after looking like it was on track for a “Goldilocks” ending for several billion years, distant galaxies all of a sudden started speeding up in their recession from one another. The expansion of the Universe was accelerating, and that required a new type of energy that was different from all the known forms of matter and radiation: something we named, for better or worse, dark energy. As our measurements of the distant, expanding Universe improved, we found that dark energy behaved in a specific manner: as though it were a form of energy inherent to space itself, behaving equivalently to Einstein’s “cosmological constant” in General Relativity.
This was beyond puzzling: it was baffling. If there were a cosmological constant present, it comes without explanation for why it’s non-zero or how it got to have the value that it does. If we attempt to calculate the zero-point energy of space using quantum field theory, we get nonsense answers that are ~10120 times too large. Many have assumed that this is simply evidence that we don’t know how to calculate the zero-point energy, and that it must all cancel away: equaling zero after all.
But then what causes dark energy? Why does it behave as though it’s some form of energy inherent to space itself, rather than diluting like matter or radiation does? Although many new hypotheses have emerged — a new field, a new parameter, or some other type of new physics — no evidence has emerged supporting any of them.

One interesting idea that was explored at length in the mid-2000s was the idea that dark energy could have arisen due to the (negative) binding energy that resulted from the formation of stars, galaxies, galaxy clusters, and the great cosmic web: the structure in the Universe. The equations that we use to calculate how the Universe expands assume that the Universe obeys the cosmological principle: that it’s isotropic (the same in all directions) and homogeneous (the same in all locations) everywhere. This is accurate if you “smear out” the cosmic web — the average density in a region of space a billion or more light-years in radius is pretty much the same everywhere — but on smaller scales, it’s very clear this assumption is invalid.
A fun calculation that one can do, and that I myself did in a paper back at that time, is to actually quantify the effects of this “inhomogeneity energy” and see how it behaves. It turns out that you can quantify:
- the gravitational potential energy (long-dashed line, below),
- the inhomogeneity energy (short-dashed line, below),
- and the kinetic energy (solid line, below),
arising from gravitational imperfections, or the departure from perfect smoothness, at all times in the Universe. Those curves not only never rise above the ~0.1% level in terms of how they affect the Universe, but even irrespective of that, they never behave as dark energy does: as a cosmological constant or some other form of energy inherent to space itself.
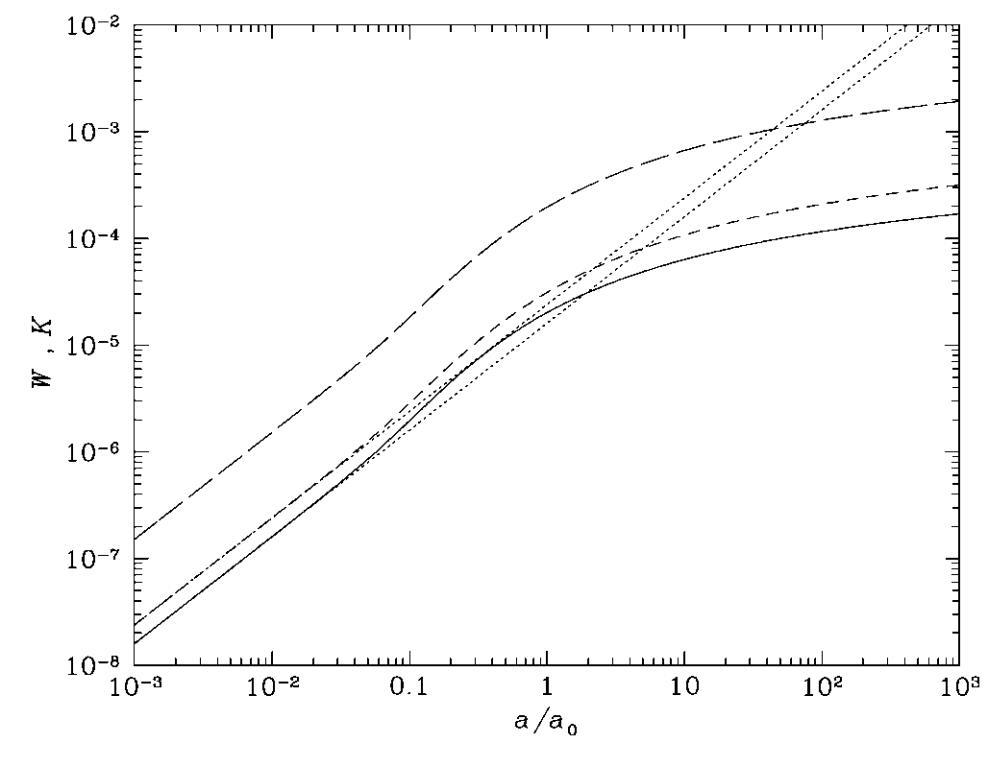
The only place left where there was any “wiggle room” for this sort of effect to occur — what we call a “backreaction” effect, because it would be an effect that arises as the Universe responds in an oppositional way to something that occurs within it — would occur where singularities arose: in the interiors of black holes. That kind of treatment went beyond what anyone knew how to calculate, but it was difficult to imagine that black holes would matter very much for three reasons.
- For one, we can quantify how much gravitational binding energy there is in black holes, and it’s only about 0.01% of the needed amount of energy to explain dark energy.
- For another, the dark energy density needs to remain constant over time, but the number density and mass density of black holes decreases over time, especially at very late times.
- And for yet another, individual black holes actually grow over time and new black holes continuously form, but this growth occurs much more slowly than the rate at which the Universe expands.
Although no one had been able to do a full calculation for “How does the energy in black holes contribute to the expansion of the Universe?” the way we’ve been able to do it for other contributions, it didn’t seem like a very compelling pathway.
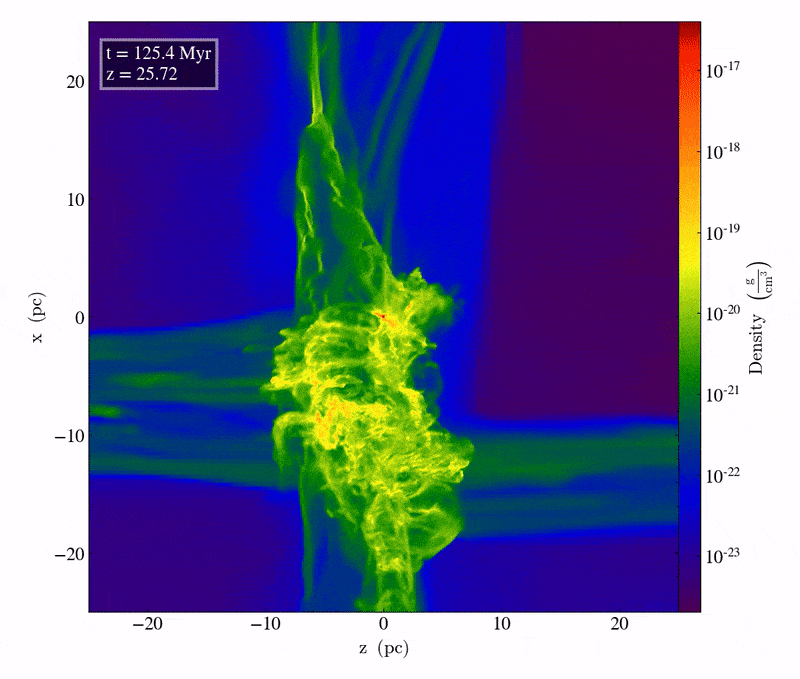
Which is why it was an absolute shock to see the headline appear, just days ago, that “Black holes are the source of dark energy.” Even more surprisingly — at least, to me — was that when I went into the scientific paper itself, this was based not on a theoretical calculation, but rather on observational evidence, which was absolutely shocking to see. The overall claim is that black holes, and specifically, supermassive black holes, couple to the Universe’s expansion on the largest cosmic scales, and that the specific way that they must couple could potentially explain some or even all of the dark energy effects we observe.
But is that a true claim? And what leads them to make such a claim? What did they observe, what does it imply, and how does the black hole-dark energy connection work? (Can you tell that I’m skeptical?)
They start by reminding us that based on what we’ve seen from black holes on all scales, including:
- from stellar mass black holes that merge and emit gravitational waves,
- from the direct observations of the photons bent around a black hole’s event horizon,
- and from hot plasma, gas, and stars seen in orbit (and the light they emit) around supermassive black holes at the centers of galaxies,
we know that the realistic black holes we have in the Universe typically rotate extremely rapidly: close to the speed of light. This means that their singularities are not just “point masses” on the inside, but they have spin/angular momentum as well, which means they have complex interior geometries in General Relativity: given by the Kerr solution.
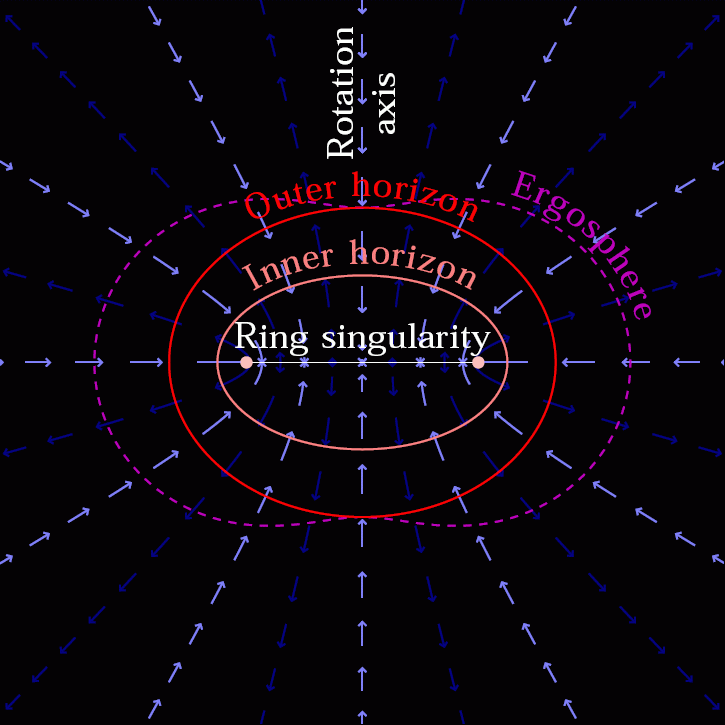
All of that is fine. But General Relativity is notoriously difficult to work with, particularly if your system is complicated in terms of what’s at play in your Universe. For example, if all you have is empty, unchanging space with no matter or energy in it, your spacetime is simply flat: the same spacetime we have in Special Relativity, with no gravitational effects. If you put down one point mass that doesn’t spin, you get the spacetime for a black hole with a spherical event horizon: a Schwarzschild black hole. If you put down a point mass that does spin, you get the aforementioned (and illustrated, above) Kerr black hole. And if you try to put down a second point mass in either the rotating or the non-rotating case, the equations become unsolvable; you can only approximate them using numerical techniques.
- The “flat, empty space” solution was discovered by Hermann Minkowski (Einstein’s physics teacher!) back in 1908.
- The “non-rotating point mass” solution was discovered by Karl Schwarzschild in 1916: just months after Einstein introduced General Relativity in its final form.
- The “rotating point mass” solution was discovered by Roy Kerr (who’s still alive in 2023!) back in 1963, and who many believe should have won a share of the Nobel Prize awarded in 2020 for black holes.
- And the fact that “two masses” cannot be solved, except via numerical approximation techniques, has been a well-known phenomenon for generations.
If it teaches you anything, it’s that General Relativity is very hard. Even today, only a couple of handfuls of exact solutions exist.

One of the exact solutions that’s been discovered is for a Universe with a cosmological constant: the equivalent of dark energy. (It’s known as the de Sitter solution.) Another is for a Universe that’s uniformly filled with matter, radiation, and any other form of energy: the generic solution for an expanding (or contracting) Universe. (It’s known as the Friedmann-Lemaître-Robertson-Walker solution.)
Now, here’s a fun (or not so fun) fact: you can combine the “point mass” and the “cosmological constant” solutions together, and get a spacetime known as Schwarzschild-de Sitter. You can also put the “rotating point mass” and the “cosmological constant” solutions together to get a Kerr-de Sitter solution.
But we live in an expanding Universe with a cosmological constant, matter, and radiation, and need the full Friedmann-Lemaître-Robertson-Walker solution, and would like to combine that with the Kerr solution by embedding a rotating point mass within it. That’s something we really don’t know how to do: we don’t have an exact solution for that at all. But this is precisely what the new paper is about: attempting to make this connection in a reasonable and consistent way, and to tie the growth of realistic (Kerr) black holes to the overall expansion of the Universe, even arbitrarily far away from the black hole itself.
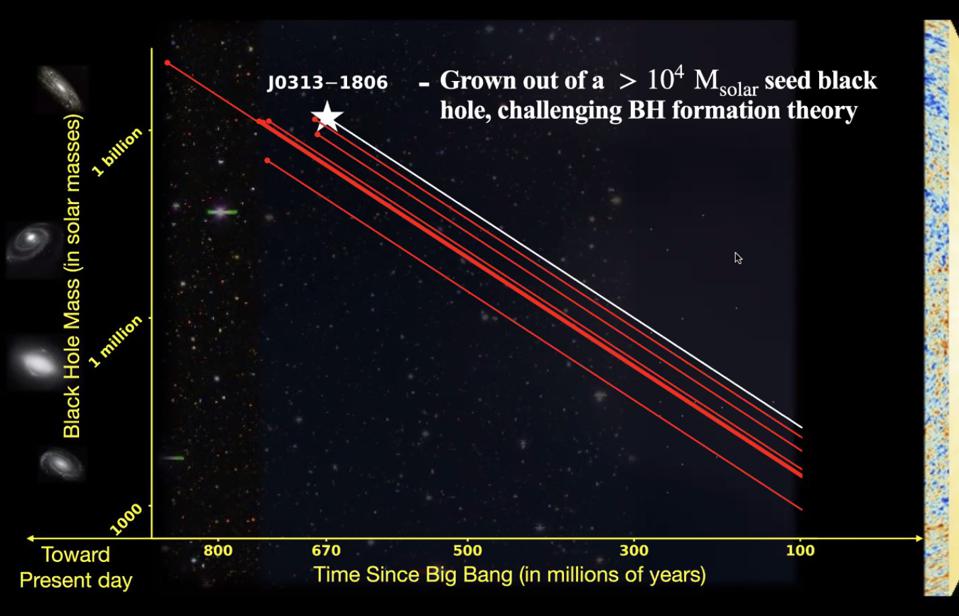
How do you do this? The approach the authors take is as follows.
- They look at multiple samples of elliptical galaxies from across cosmic time: nearby (modern) galaxies, galaxies from ~6.6 billion years ago, galaxies from ~7.2 billion years ago, and galaxies from ~9.6 billion years ago.
- They assume that there’s a universal relationship between the mass of the central black hole and the mass of the stars within a galaxy, which can evolve over cosmic time but should be universal at any particular time.
- Then, they use their model of “cosmological coupling,” assuming there exists a relationship between the mass of a black hole at any particular cosmic time (or, more accurately, redshift) and the mass of the black hole at the time it “becomes cosmically coupled” to the expansion rate, to determine whether (and, if so, how) the coupling parameter, k, has the same value across cosmic time.
If k = 0, the minimum allowable value, then there’s no coupling and the mass of the black hole you infer doesn’t change with time or affect the cosmic expansion.
If k = 3, then the coupling is at the maximum allowable value, and the mass of the black hole increases as the cube of the redshift ratio, and the black hole acts like it causes dark energy.
And if k is anywhere in between those values, then the mass of the black hole grows, but more slowly than in the maximal case, and the black hole behaves as something that contributes to the expansion of the Universe, but neither as matter nor as dark energy.
They leverage the various samples they chose and assert that if they find the same coupling ratio, k, across all of the different samples, then this picture is valid and it allows us to observationally determine how black holes contribute to the Universe’s expansion.
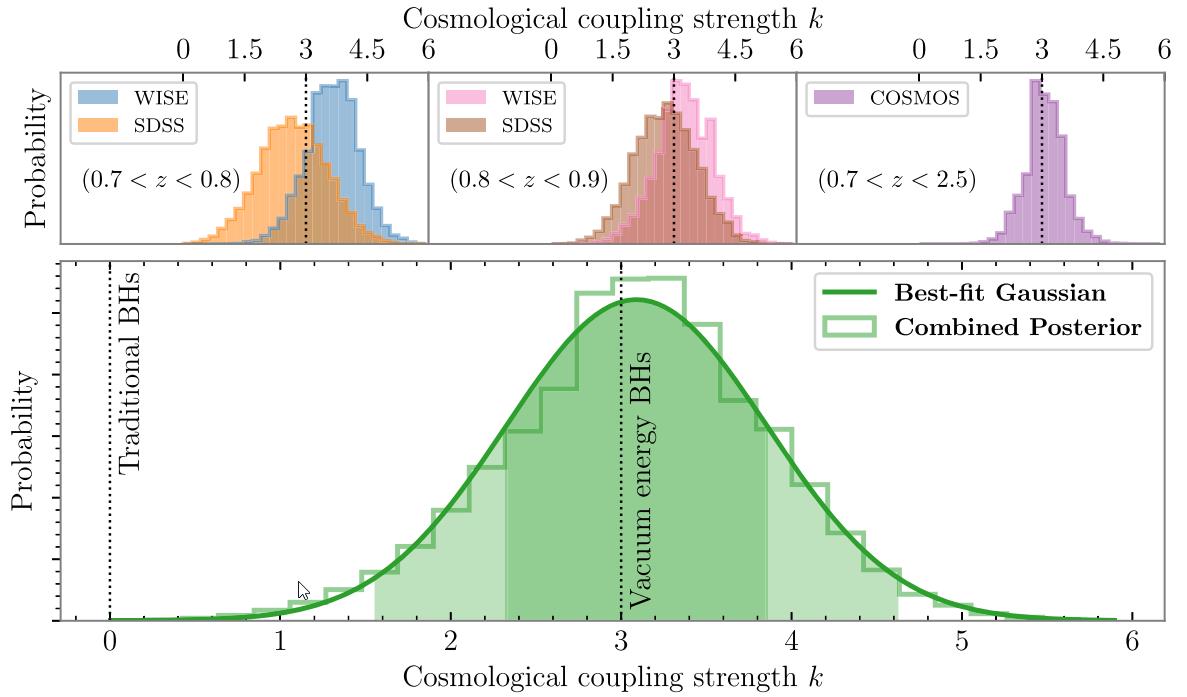
Lo and behold, as you can see above, they find that k looks like it’s 3 across all samples, and therefore black holes cosmically couple to the Universe’s expansion and behave like dark energy. The notion that the black holes aren’t cosmically coupled, which is k = 0, is disfavored at the 99.98% level, or the equivalent of 3.9-σ statistical significance. In physics and astronomy, 5-σ is the “gold standard,” so this isn’t a slam-dunk by statistical standards, but it is highly suggestive.
If, that is, you believe it. Do you?
The alternative explanation — which I’m going to put forth here for your consideration — is that this method is 100% unsound. It’s possible that k = 0, that there is no coupling, and that what’s actually happening is that these black holes are growing by purely astrophysical processes: the infall and accretion of matter over time, as well as from mergers and acts of galactic cannibalism. The authors are assuming the existence of a coupling that isn’t there and ascribing the perceived evolution of the black hole-to-stellar mass ratios to a coupling, when what’s happening is these galaxies and their black holes are evolving. Since we’re only measuring each galaxy at a “snapshot” in time, we have no way of knowing how any individual object is evolving, and this particular method is precisely how the article authors are fooling themselves, and by extension, anyone who believes them.

But I’m not here to ask you what you believe; this column is called “Ask Ethan” and (at least some of) you asked me, so I’ll tell you what I think.
This is what I call “a swing.” As in, “That’s an extraordinarily unlikely scenario, but look at this claim, going for it anyway, and if no one calls them on it, they just might get away with it.”
I think this is an avenue of research, to actually figure out whether and how black holes actually do couple to the cosmic expansion, that should continue to be explored. I think that it’s very unlikely, but not 100% impossible, that there really is a connection between black hole interiors and the external cosmic expansion.
But I think the default assumption should be that these black holes are really just behaving as any other mass in the Universe behaves, and that this empirical approach of “We’re going to measure the masses of supermassive black holes and stars in elliptical galaxies and use that to infer the cosmological coupling” totally glosses over the big astrophysics question that should be investigated: how do these black holes grow and evolve over cosmic time? Until you know that answer, you’re attributing a measured effect to what might be entirely the wrong cause.
It’s an interesting idea and one that I can’t say is definitely wrong. But despite the asserted “0.02% chance of it being a fluke,” I’d definitely bet against this as not only being the explanation for dark energy, but that any sort of significant cosmological coupling exists at all.
Send in your Ask Ethan questions to startswithabang at gmail dot com!
(Author’s note: ES would like to clarify that the authors of this study did investigate possible astrophysical mechanisms for black hole mass growth by accretions and mergers at length, and were unable to account for the observed mass growth through the channels explored.)