The biggest holes in NASA’s suite of observatories
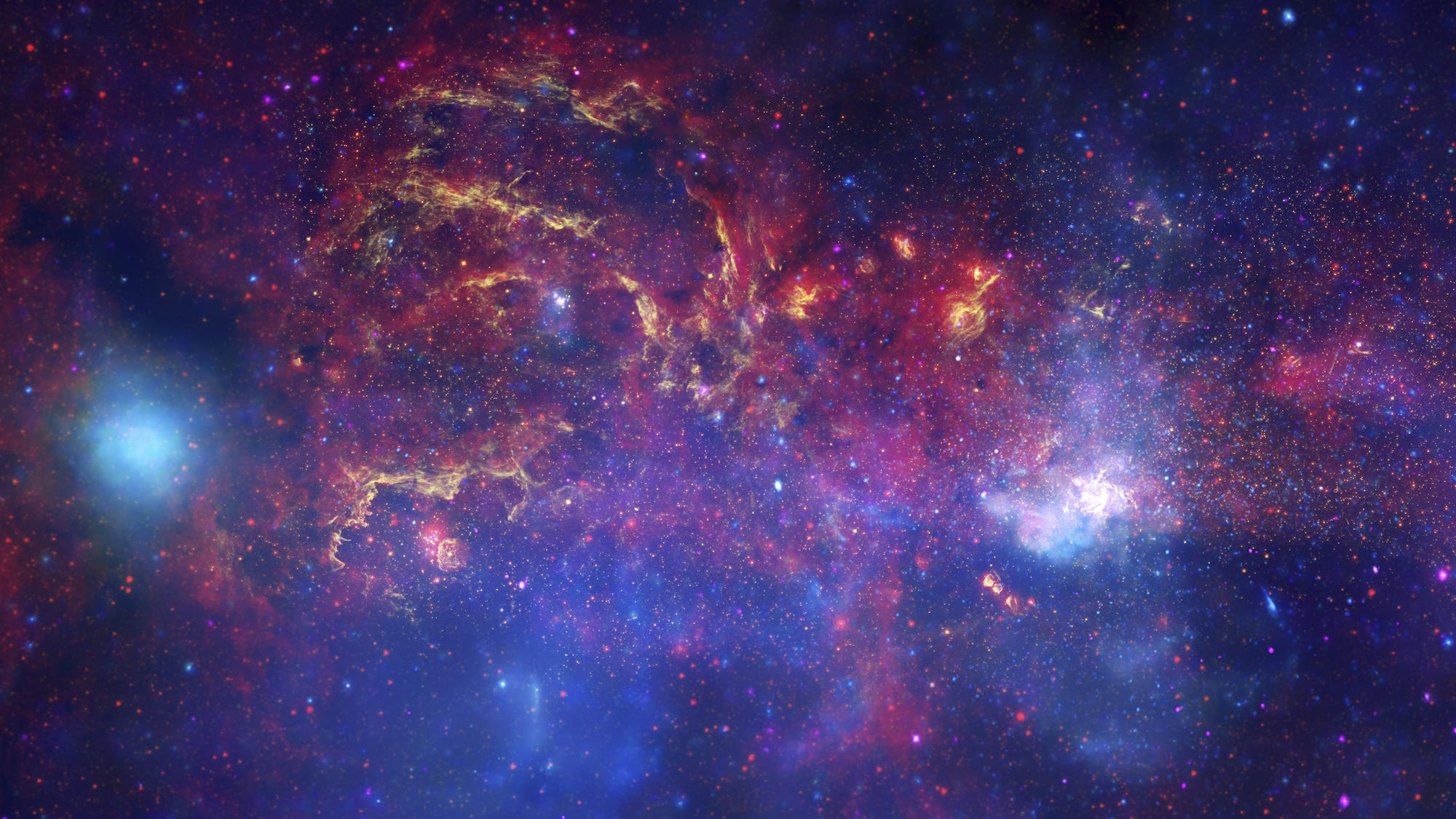
- Over the past several decades, NASA has launched observatories that have spanned the full gamut of electromagnetic wavelengths: from high energy gamma-rays all the way down to radio waves.
- With famous flagship missions like the Chandra X-ray observatory, the optical Hubble space telescope, and the near-infrared and mid-infrared JWST, NASA’s eyes on the sky are impressive and informative.
- However, as our technological capabilities advance, so does the potential for new discoveries. If we don’t build new observatories to take advantage of such capabilities, that science just never gets done.
From here on Earth, our views of the Universe are impressive and expansive, but are also fundamentally limited. We can build observatories as large as we like — even on the highest, driest mountaintops our planet has to offer — with instruments that are as up-to-date and sophisticated as our budgets allow. However, even with those capabilities, there are only a few narrow “windows” in the electromagnetic spectrum where light can get through unimpeded by the atmosphere. While the longest wavelengths, at radio frequencies, are largely unaffected by the atmosphere, the same cannot be said for all other wavelengths.
At microwave, far-infrared, and most mid-infrared wavelengths, the atmosphere is completely opaque to light coming from the Universe beyond our planet. The same is true for gamma-rays and X-rays; one must go to space to observe them. Most of the ultraviolet and near-infrared is opaque, and — just like the optical — the parts that aren’t are severely distorted by Earth’s atmosphere. Even with the impressive advances brought by modern adaptive optics systems, sending your observatories to space offers advantages unachievable from the ground.
With the unprecedented deluge of new information brought to humanity by JWST, it’s time to remember what NASA astrophysics is all about. With a new vision and a new focus, a suite of great observatories could be exactly what humanity needs to advance further than ever before in the 21st century.
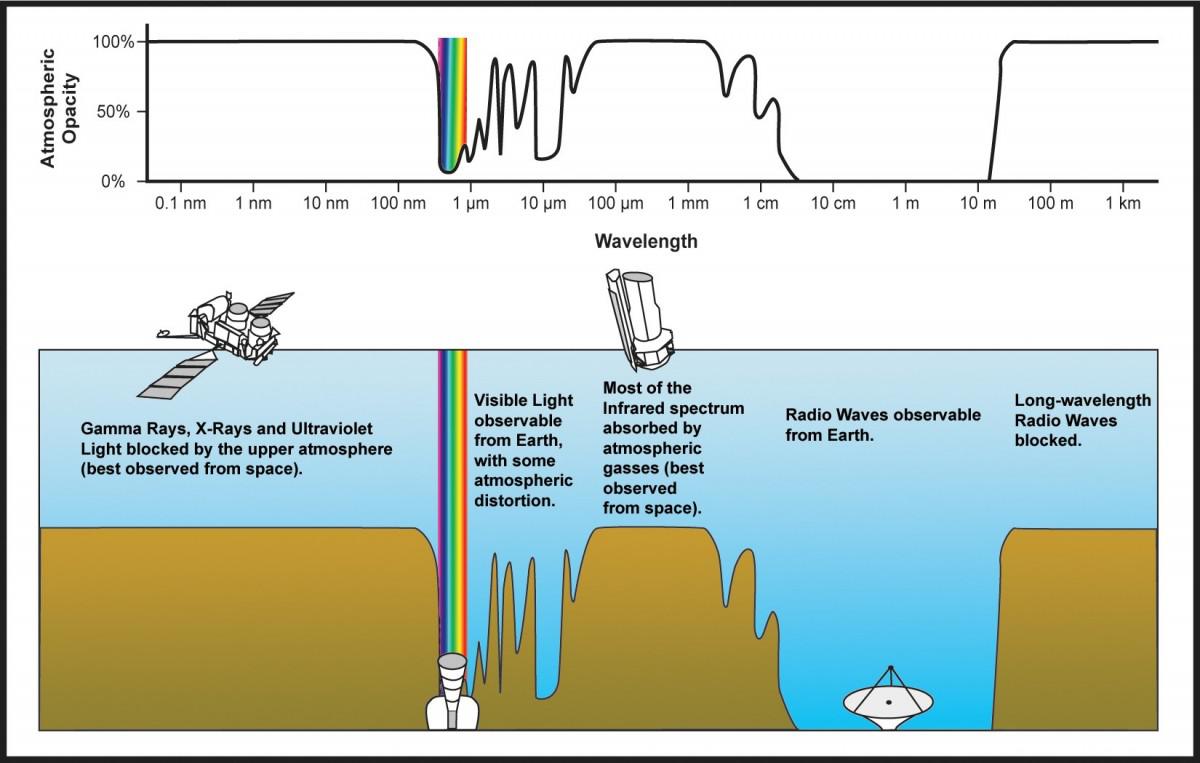
A lot of people are presently marveling at what JWST has brought to the table, and rightly so. Our largest, most precise, and arguably most ambitious space telescope of all-time has shown us details about the Universe that most of us never could have imagined. Sure, we fully expected that JWST would break a whole slew of cosmic records, and it already has, including for the:
- most distant galaxy,
- earliest proto-cluster of galaxies,
- most distant gravitational lens,
- and most distant red supergiant star,
among many others. It’s also helped show that the Hubble tension is more robust than we had previously realized, and that we appear to truly have an epic puzzle on our hands with that one.
But those aren’t even the greatest findings that the telescope has made! Sure, they’re examples of what it was designed to do, but JWST’s greatest discoveries were things that were entirely unexpected. By teaming up with NASA’s Chandra, it found low-mass, faint early galaxies that house supermassive black holes that are about as massive as all of the stars within those galaxies combined: a mass ratio that had never even been approached before JWST. It implies that supermassive black holes cannot form from stars alone, but require some other mechanism that means many of these black holes predate the stars in the galaxies that house them.
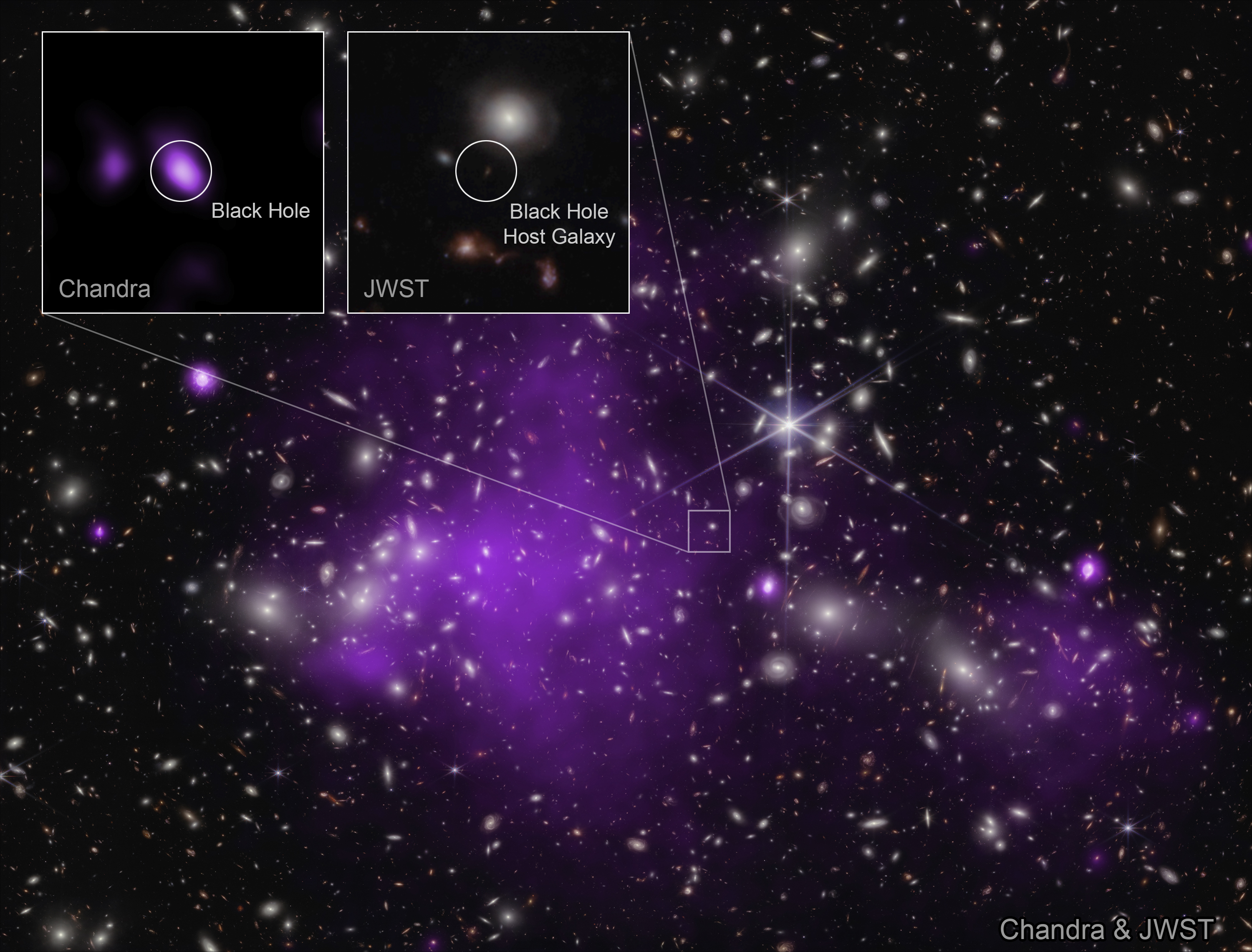
It also peered into the Orion Nebula, expecting to see new details about the birth of stars and the properties of young stellar systems with its novel capabilities, and it, in fact, did. But what it also found in that nebula was impressive and unexpected: a large number of Jupiter-mass planets, floating freely in these nebulous regions without any parent star of their own. In fact, a full 9% of those Jupiter-mass planets have a binary companion of another Jupiter-mass planet that they orbit, making these Jupiter Mass Binary Objects (or JuMBOs) another unexpected find of this observatory.
The large number of early, bright galaxies that were found initially appeared to “break” cosmology, as what we were seeing wasn’t what was predicted. It took four independent contributions, all working together:
- JWST’s optical overperformance,
- the use of higher-resolution simulations that focus on the rarest, largest initial overdensities,
- the fact that star-formation is bursty, not continuous,
- and the contributions of active supermassive black holes to brightness,
in order to finally explain what we were observing with JWST.
And, in perhaps the greatest surprising scientific find, while looking at the nearby star Fomalhaut, the closest star to Earth with a known debris disk around it, JWST found not one, not two, but evidence for three belts within the system. It possesses an analogue to our asteroid belt and our Kuiper belt, but it also seems to possess an intermediate belt, something we didn’t even know to look for. It now makes us question how many belts are “typical” in a stellar system, and makes us ask why we “only” have two?

How did JWST make these discoveries? It wasn’t designed to make them, of course, and it wasn’t because we knew (or even imagined) that they might be out there, and we chose to look for them.
The reason we were able to find out these unexpected properties about the Universe is because we designed JWST to have a specific set of properties that endowed it with what scientists call “discovery potential.” Those properties include:
- high optical resolution, which allows us to see features in detail at small angular scales,
- high spectral (or energy) resolution, which allows us to see how objects emit energy across a variety of wavelengths,
- large amounts of light-gathering power, which allows us to see fainter objects than all previous observatories,
- observing speed, which allows us to probe the same region of sky again and again for any changes that have occurred within it,
- and high sensitivity compared to all previous observatories at the relevant wavelengths.
Although JWST has often been called the “successor to Hubble” as NASA’s big flagship observatory of the 2010s and 2020s, it only has a small overlap with Hubble in terms of wavelength ranges. Hubble was designed as an optical observatory, with limited capabilities in the ultraviolet and in the near-infrared. JWST, meanwhile, was designed to specialize in infrared wavelengths (in both the near-infrared and mid-infrared), with limited capabilities in the optical: where it overlaps with Hubble. It’s more accurate to treat JWST as the successor to other infrared telescopes, such as Spitzer or WISE, which were far smaller and less sensitive than JWST in practically every way.

We can recognize very swiftly that NASA has a large suite of observatories that have been built across an enormous variety of wavelengths.
- We have the Swift satellite as well as the Fermi mission for investigating the gamma-ray sky.
- We have the Chandra X-ray observatory, launched way back in 1999, as (still!) the most powerful X-ray observatory available to humanity.
- We had the now-defunct GALEX in the ultraviolet, with the future UVEX coming up in a few years (estimated 2030) and with NASA partnering with Israel to launch ULTRASAT in 2026.
- We have Hubble, even after all these years, still serving as our primary ultraviolet/optical/near-infrared observatory for both imaging and spectroscopy, with the future wide-field Nancy Grace Roman observatory slated for launch within the next 10 years and Habitable Worlds Observatory slated to be the ultimate successor to Hubble in terms of resolution, light-gathering power, and coronagraphic imagery.
- In the near-infrared and mid-infrared, we have JWST, which is surpassing all prior observatories in similar wavelengths by factors of 10s, 100s, or even 1000s.
- In the far-infrared, we had ESA’s Herschel mission for a time; now we have none. In fact, other than an explorer-class mission that’s still being designed (we have one in the X-ray, too), we have no plans to observe the far-IR skies.
- In the microwave, we had NASA’s COBE and WMAP, and then ESA’s Planck, to observe the Big Bang’s leftover glow in unprecedented detail; these missions have now all been completed.
- And in the radio, we can do almost everything on Earth, but pollution from terrestrial (and satellite) signals must always be reckoned with as a source of noise.
Despite this fleet of observatories, we must recognize that a large amount of time has passed since even the most sophisticated of these missions has launched (except for the very new JWST), and that we could do a whole lot better.
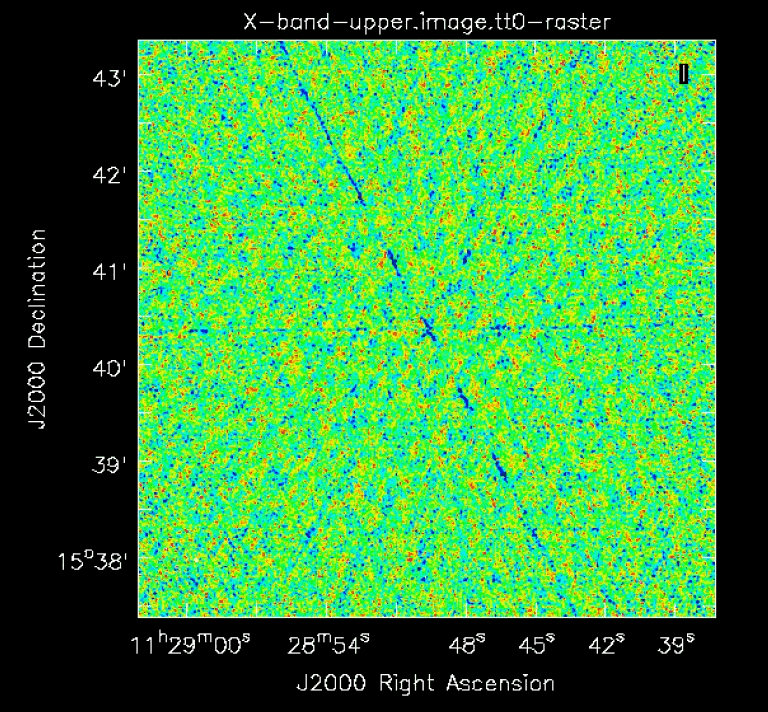
That’s why the idea of “discovery potential” is so important. Across all of these different wavelength ranges, the Universe awaits. There are objects and events, existing and occurring out there in the great cosmic beyond, that are certainly going to surprise us when we discover them and measure their properties, and we have no way to predict how that’s going to occur. What we can be certain of, however, is the following:
- when we do stumble upon these objects or events, what we learn about them will be entirely dependent on the quality of our observatories and our observations that we’re taking with them,
- and that if we don’t advance in our technological capabilities from where we are now to where we’ll be when we do make those critical observations, we won’t be able to make those discoveries.
That’s why it’s so critical, when we look to the future, to identify where the biggest gaps are: the gap between what the technical capabilities were of the previous generation of observatories that observed the Universe over a specific wavelength range, versus the technical capabilities that a new observatory would possess if it were built with cutting-edge technology within the type of budget (around $5-10 billion) that a flagship observatory can command. Although “ranking” these various priorities is going to be subjective in a great number of ways, my own professional assessment is that there are four major areas that are “no brainers” as far as planning a future suite of great observatories: something that was strongly recommended by the National Academies of Science in their recent decadal survey of the field.
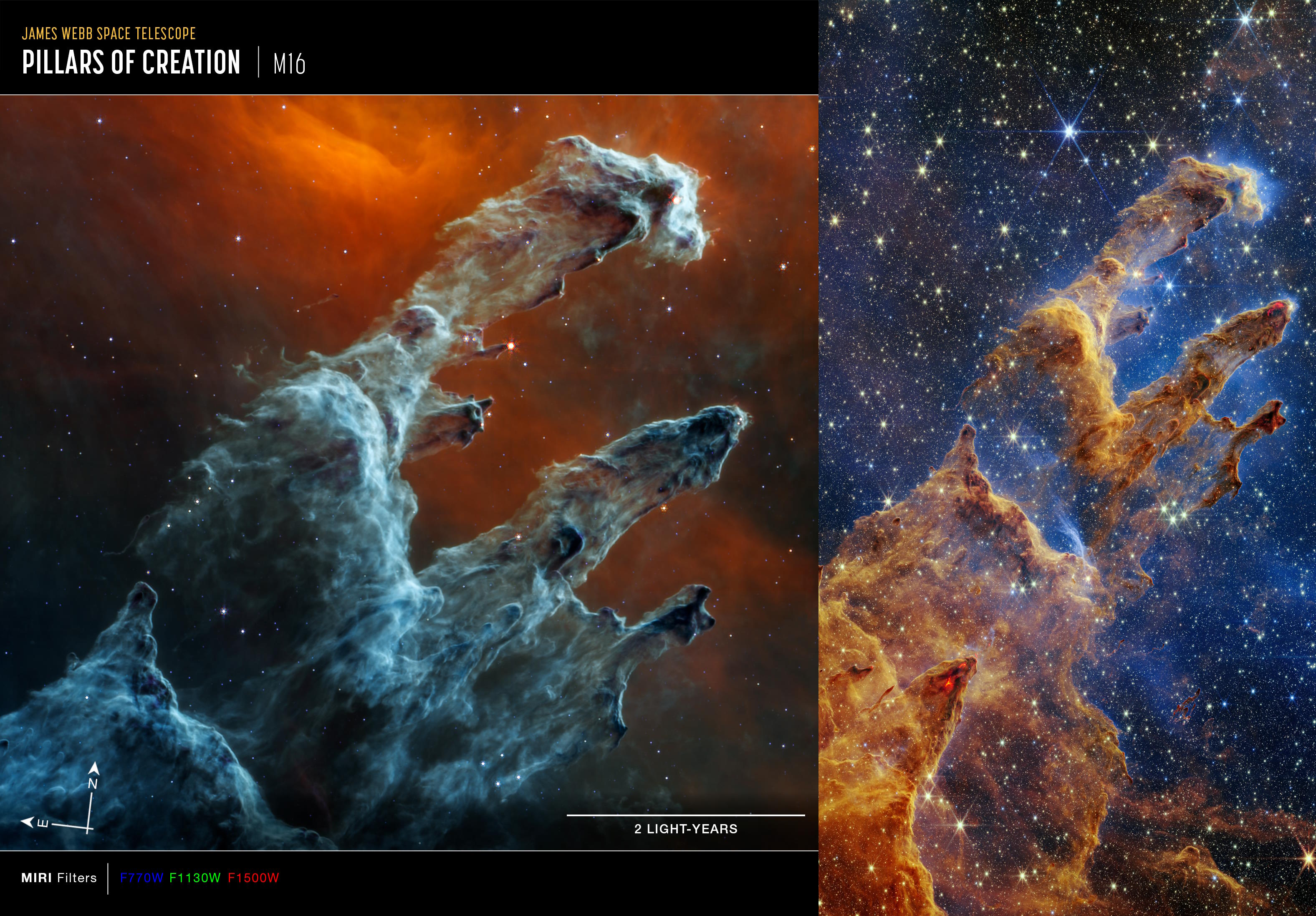
1.) The far-infrared. I know, a lot of you are going to read this and think, “Come on, man, JWST does infrared science, so why do you want another infrared observatory?” And the answer is simple: different wavelengths are sensitive to different features. Take a look at the above images, taken by JWST in the near-infrared as well as in the mid-infrared. Notice any differences? Of course you do; whereas near-infrared wavelengths are sensitive to heated gas and newborn stars, mid-infrared wavelengths are sensitive to cool dust and shrouded protostars. Far-infrared wavelengths are sensitive to features that only appear at even lower temperatures and offer an entirely different set of insights into the physical properties of the Universe where it observes.

The proposed Origins Space Telescope would be approximately the same size as JWST, but would be even colder: cooled by liquid helium. With its designed specifications, it would be more than 1000 times as sensitive as either ESA’s Herschel or NASA’s SOFIA missions were, while covering wavelength ranges that both ALMA and JWST cannot. If we want to learn about growing black holes, about planet and star formation, or about mapping out the locations of heavy elements, a far-infrared telescope is exactly what we need. It’s the great hope of many that this “forgotten wavelength” isn’t overlooked for much longer, as the unseen Universe calls to us.
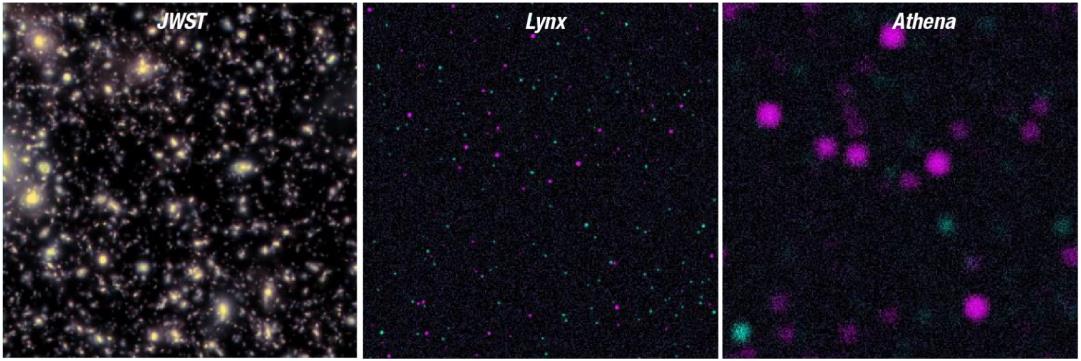
2.) The X-ray. NASA’s Chandra and the ESA’s XMM-Newton missions have been spectacular as far as what they’ve taught us about the Universe, with Chandra continuing to work hard and reveal details about even the ultra-distant cosmos. But the ESA’s proposed Athena mission is being redesigned to be smaller, cheaper, and worse in every way, and NASA’s ambitious plans for an X-ray flagship mission, Lynx, was not selected to move forward. That’s too bad! Lynx is still needed, and would be an absolute game-changer for X-ray astronomy. When you compare what a state-of-the-art, modern X-ray observatory like Lynx could deliver with what Chandra does and even with what the originally-designed Athena mission would do, you’d find that a modern observatory would have:
- a superior optical assembly, which yields better resolution, sensitivity, and a greater field-of-view,
- a better calorimeter, allowing us to more precisely determine the energy of each X-ray photon,
- a high-definition imager, which would be ideal for identifying rapid transient and variable sources,
- and the advent of a grating spectrometer, which would reveal signatures and locations of elements at high-resolution.
Lynx, if it does get built, will have 16 times the field-of-view of Chandra, an energy and flux sensitivity that’s 50-to-100 times greater than Chandra, and it would even achieve 10 times the resolution and better spectroscopic power at low-energies than the original design of Athena. In terms of discovery potential, we’re leaving it all on the table by not building a next-generation X-ray observatory.
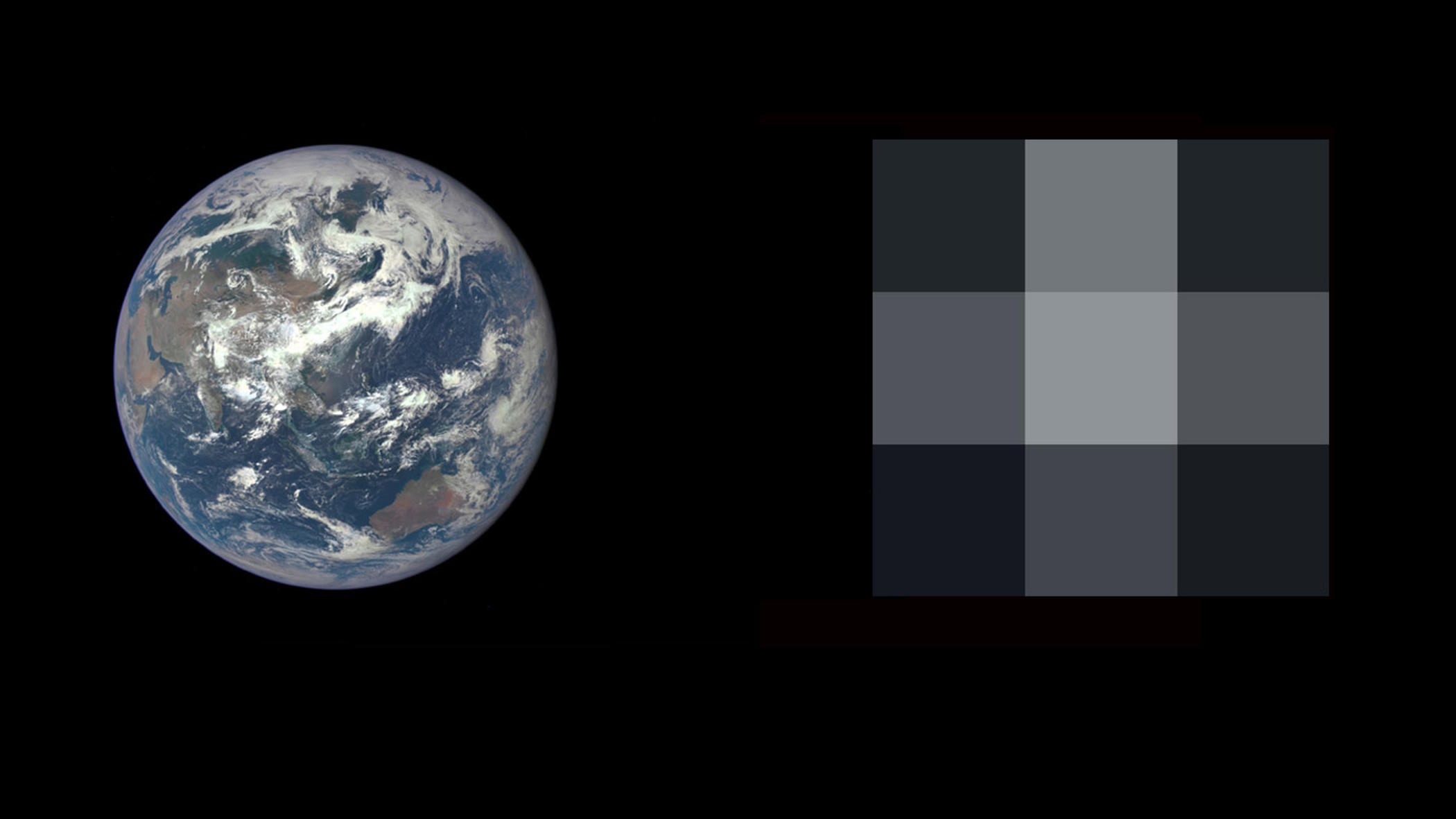
3.) The optical, as well as the near-UV and the near-infrared. This one is at least going to be addressed! Sure, humanity has had Hubble from NASA for a long time, and recently, the ESA has launched the Euclid mission, which is mapping out a large area of the Universe nearly as deeply and with nearly the resolution of Hubble. But Euclid is also a relatively inexpensive mission, and if we were to invest the proper resources, we could go much farther than even it is going to take us. For deep, wide-field views, that’s what the Nancy Grace Roman Space Telescope will do, and for narrow, deep, and high-resolution views, that’s what the Habitable Worlds Observatory is going to do.
And they’re both on track to get built, which is extremely exciting. Nancy Roman has similar features to Hubble, but has 50-100 times the field-of-view, meaning it can probe enormous areas of the sky to record depth in record time: accomplishing in hours what it took Hubble weeks of observing time to accomplish previously. Habitable Worlds Observatory, meanwhile, will be the highest-resolution imager ever launched in the optical, and with upgraded coronagraphic capabilities, will be the first space telescope to capture a direct image of an Earth-sized world at Earth-like distances from Sun-like stars. As impressive as this science is going to be, it’s important to recognize that there are still greater leaps, in terms of discovery potential, to be gained in both the far-infrared and in the X-ray than what these two flagship missions will bring to the optical.

4.) The radio, if we go to the Moon’s far side. This is one aspect that’s generally underappreciated: here on Earth, even though the atmosphere is transparent to radio light, human activity — both on Earth and in the space surrounding the Earth — means that our planet is never completely “radio-quiet” as we work to investigate the Universe. But there is one location relatively nearby that is pristine as far as being radio quiet goes: the far side of the Moon. The Moon itself serves as an excellent shield from the radio signals coming from Earth and the satellites that orbit it, and a radio telescope (or array of radio telescopes) on the Moon’s far side would be capable of new types of science that cannot be conducted on Earth at present.
If we want to explore the Universe, we need only build and operate the observatories that would permit this type of exploration. With the bombshell news that NASA boosts the economy by about $3 for every $1 that we spend on it, it seems like building all of these observatories would be a no-brainer, especially knowing that it would benefit all of us and society as a whole by triple the amount it costs to construct and operate them. But what we should do and what we’ll actually do don’t always line up. The fact is there are many excellent justifications for building a new suite of great observatories. From a scientific point of view, looking to “discovery potential” is precisely why we should all be excited about exploring the unknown: not because of what we know we’re going to find, but because of the unbridled potential for nature, ultimately, to surprise us.