Ask Ethan: What Is Spacetime?
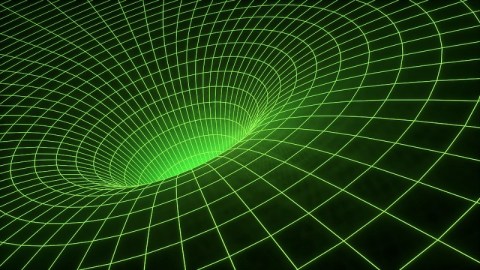
It’s the most fundamental fabric of the Universe itself. But how does it work?
“‘Space-time’ — that hideous hybrid whose very hyphen looks phoney.”
–Vladimir Nabokov
When it comes to understanding the Universe, there are a few things everyone’s heard of: Schrödinger’s cat, the Twin Paradox and E = mc². But despite being around for over 100 years now, General Relativity — Einstein’s greatest achievement — is largely mysterious to everyone from the general public to undergraduate and graduate students in physics. For this week’s Ask Ethan, Katia Moskovitch wants that cleared up:
Could you one day write a story explaining to a lay person what the metric is in GR?
Before we get to “the metric,” let’s start at the beginning, and talk about how we conceptualize the Universe in the first place.
At a fundamental level, the Universe is made up of quanta — entities with physical properties like mass, charge, momentum, etc. — that can interact with each other. A quantum can be a particle, a wave, or anything in some weird in-between state, depending on how you look at it. Two or more quanta can bind together, building up complex structures like protons, atoms, molecules or human beings, and all of that is fine. Quantum physics might be relatively new, having been founded in mostly the 20th century, but the idea that the Universe was made of indivisible entities that interacted with each other goes back more than 2000 years, to at least Democritus of Abdera.
But no matter what the Universe is made of, the things it’s composed of need a stage to move on if they’re going to interact.
In Newton’s Universe, that stage was flat, empty, absolute space. Space itself was a fixed entity, sort of like a Cartesian grid: a 3D structure with an x, y and z axis. Time always passed at the same rate, and was absolute as well. To any observer, particle, wave or quantum anywhere, they should experience space and time exactly the same as one another. But by the end of the 19th century, it was clear that Newton’s conception was flawed. Particles that moved close to the speed of light experienced time differently (it dilates) and space differently (it contracts) compared to a particle that was either slow-moving or at rest. A particle’s energy or momentum was suddenly frame-dependent, meaning that space and time weren’t absolute quantities; the way you experienced the Universe was dependent on your motion through it.
That was where the notion of Einstein’s theory of special relativity came from: some things were invariant, like a particle’s rest mass or the speed of light, but others transformed depending on how you moved through space and time. In 1907, Einstein’s former professor, Hermann Minkowski, made a brilliant breakthrough: he showed that you could conceive of space and time in a single formulation. In one fell swoop, he had developed the formalism of spacetime. This provided a stage for particles to move through the Universe (relative to one another) and interact with one another, but it didn’t include gravity. The spacetime he had developed — still today known as Minkowski space — describes all of special relativity, and also provides the backdrop for the vast majority of the quantum field theory calculations we do.
If there were no such thing as the gravitational force, Minkowski spacetime would do everything we needed. Spacetime would be simple, uncurved, and would simply provide a stage for matter to move through and interact. The only way you’d ever accelerate would be through an interaction with another particle. But in our Universe, we do have the gravitational force, and it was Einstein’s principle of equivalence that told us that so long as you can’t see what’s accelerating you, gravitation treats you the same as any other acceleration.
It was this revelation, and the development to link this, mathematically, to the Minkowski-an concept of spacetime, that led to general relativity. The major difference between special relativity’s Minkowski space and the curved space that appears in general relativity is the mathematical formalism known as the Metric Tensor, sometimes called Einstein’s Metric Tensor or the Riemann Metric. Riemann was a pure mathematician in the 19th century (and a former student of Gauss, perhaps the greatest mathematician of them all), and he gave a formalism for how any fields, lines, arcs, distances, etc., can exist and be well-defined in an arbitrarily curved space of any number of dimensions. It took Einstein (and a number of collaborators) nearly a decade to cope with the complexities of the math, but all was said and done, we had general relativity: a theory that described our three-space-and-one-time dimensional Universe, where gravitation existed.
Conceptually, the metric tensor defines how spacetime itself is curved. Its curvature is dependent on the matter, energy and stresses present within it; the contents of your Universe define its spacetime curvature. By the same token, how your Universe is curved tells you how the matter and energy is going to move through it. We like to think that an object in motion will continue in motion: Newton’s first law. We conceptualize that as a straight line, but what curved space tells us is that instead an object in motion continuing in motion follows a geodesic, which is a particularly-curved line that corresponds to unaccelerated motion. Ironically, it’s a geodesic, not necessarily a straight line, that is the shortest distance between two points. This shows up even on cosmic scales, where the curved spacetime due to the presence of extraordinary masses can curve the background light from behind it, sometimes into multiple images.
Physically, there are a number of different pieces that contribute to the Metric Tensor in general relativity. We think of gravity as due to masses: the locations and magnitudes of different masses determine the gravitational force. In general relativity, this corresponds to the mass density and does contribute, but it’s one of only 16 components of the Metric Tensor! There are also pressure components (such as radiation pressure, vacuum pressure or pressures created by fast-moving particles) that contribute, which are three additional contributors (one for each of the three spatial directions) to the Metric Tensor. And finally, there are six other components that tell us how volumes change and deform in the presence of masses and tidal forces, along with how the shape of a moving body is distorted by those forces. This applies to everything from a planet like Earth to a neutron star to a massless wave moving through space: gravitational radiation.
You might have noticed that 1 + 3 + 6 ≠ 16, but 10, and if you did, good eye! The Metric Tensor may be a 4 × 4 entity, but it’s symmetric, meaning that there are four “diagonal” components (the density and the pressure components), and six off-diagonal components (the volume/deformation components) that are independent; the other six off-diagonal components are then uniquely determined by symmetry. The metric tells us the relationship between all the matter/energy in the Universe and the curvature of spacetime itself. In fact, the unique power of general relativity tells us that if you knew where all the matter/energy in the Universe was and what it was doing at any instant, you could determine the entire evolutionary history of the Universe — past, present and future — for all eternity.
This is how my sub-field of theoretical physics, cosmology, got its start! The discovery of the expanding Universe, its emergence from the Big Bang and the dark energy-domination that will lead to a cold, empty fate are all only understandable in the context of general relativity, and that means understanding this key relationship: between matter/energy and spacetime. The Universe is a play, unfolding every time a particle interacts with another, and spacetime is the stage on which it all takes place. The one key counterintuitive thing you’ve got to keep in mind? The stage isn’t a constant backdrop for everyone, but it, too, evolves along with the Universe itself.
Have a question for Ask Ethan? Submit it to startswithabang at gmail dot com!
This post first appeared at Forbes, and is brought to you ad-free by our Patreon supporters. Comment on our forum, & buy our first book: Beyond The Galaxy!