Ask Ethan: How Can A Nation Have Nuclear Power Without The Danger Of Nuclear Weapons?
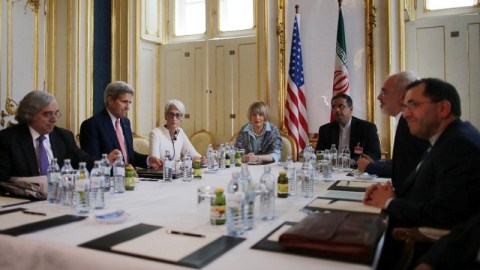
It worked for Iran two years ago, and it can work again with the right negotiations.
“We have to understand the ubiquity of energy in everything we do. Energy is core to our economy and it brings with it environmental challenges, and it’s core to our security challenges.” –Ernest Moniz
In 2015, John Kerry, then the Secretary of State, brought nuclear physicist and Secretary of Energy Ernest Moniz with him to Iran, to try and negotiate a nuclear deal. The hope was that Iran would have the freedom and ability to create energy using nuclear power, but in such a way that creating a nuclear weapon would be impossible on timescales shorter than a year. Is such a peaceful, nuclear dream scientifically possible? And if so, what would it look like? That’s what Patreon supporter Patrick Dennis wants to know:
Could you elaborate some of the scientific background on which Dr. Moniz must have briefed Kerry for those talks? Among issues that are sometimes mentioned with little or no explanation are uranium vs plutonium; materials and technology suitable for peacetime energy production vs those suitable only for weapons; breeder reactors; and illegal technology transfer.
By many metrics, nuclear power is a winner that other energy sources can’t hope to touch.
Every other energy source we have relies on either mechanical, chemical, or electromagnetic (including solar and geothermal) energy to power it. Wind power is a great example of mechanical energy: the moving wind catches the blades, which cause an internal turbine to spin, converting mechanical energy into electrical energy. Fossil fuels — including coal, oil, and natural gas — involve the combustion of carbon-containing compounds, which release chemical energy (by rearranging electron/atomic configurations) and convert it into electrical power in a variety of ways. Electromagnetic energy has an advantage that it can be converted directly into electrical energy under the right conditions, albeit in the form of direct (rather than alternating) current. But nuclear power has the advantage here.
Unlike wind, solar, or hydroelectric power, it isn’t subject to hourly, daily, or seasonal variation: you supply the fuel and the right conditions and nuclear delivers the power you need on demand. Unlike coal, oil, or natural gas, it doesn’t produce greenhouse gas emissions (because it doesn’t combust carbon), and we’re not in any danger of running out of nuclear fuel for tens of thousands of years. Instead of relying on chemical transitions, where the configurations of electrons in atoms and molecules are changed to release energy, nuclear power relies on the process of nuclear fission, where heavy elements are split apart, releasing energy via Einstein’s E = mc2. The nuclear transitions are some 100,000 times more efficient, meaning the same amount of fuel that can power a city for a day via chemical reactions can, with nuclear reactions, last for centuries.
But there’s an insidious downside to nuclear power that goes far beyond the fear of environmental and ecological catastrophe: the fact that the by-products of these nuclear reactions produce material that could be used to build an atomic bomb. With recent nuclear tests by North Koreahaving just occurred, the lingering fears of the Cold War still remaining, and many people still alive who remember the effects of the 1945 bombing of Hiroshima and Nagasaki, the fear of nuclear proliferation is real and valid, and a concern that must be addressed.
In its most basic form, nuclear fission arises from uranium ore, which is a mix of fissionable U-235 and the non-fissionable U-238. After that fuel is spent, where most of the U-235 was split apart, there are a slew of additional products. These include lower elements on the periodic table, from zinc on up, along with some highly radioactive, heavy elements not found in nature. These include:
- U-236, which is a surefire “fingerprint” of spent nuclear fuel,
- four different isotopes of plutonium: Pu-238, Pu-239, Pu-240, and Pu-241,
- and some Curium: Cu-245.
At the simplest level, the plutonium produced by burning this fissile uranium is what’s key to the possibility of producing a nuclear weapon.
About 1% of the mass of spent nuclear fuel will turn out to be plutonium. In general, there are three classifications for the “grade” of plutonium, because there’s no good, cheap, efficient way to separate out the different isotopes. Instead, the classifications are as follows:
- Super weapons grade plutonium contains less than 3% Pu-240,
- Weapons grade plutonium contains less than 7% Pu-240, and
- Reactor grade plutonium contains 7% or more Pu-240.
It’s the Pu-239 that’s the key to building a nuclear weapon in most cases, so the key to preventing nuclear proliferation, in the simplest case, is to ensure that any plutonium that’s produced is neither weapons-grade nor super weapons-grade.
When a reactor is run “normally,” which means for a long period of time and until the U-235 fuel is spent, you have no danger of producing weapons-grade plutonium. In fact, under those conditions, less than 80% of your plutonium will be the fissile Pu-239, with 19% or more becoming Pu-240. The reason for this is straightforward: nuclear fission produces neutrons, bigger nuclei have a bigger cross-section for absorbing neutrons, so while U-238 can easily absorb a neutron to become Pu-239 (after some radioactive decays), that Pu-239 can also easily absorb a neutron to become Pu-240.
The key, then, to making weapons-grade plutonium, is to irradiate that U-238 for only short periods of time: enough time to produce Pu-239, but not for long enough to create Pu-240. It’s pretty easy, by performing this short-period irradiation, to produce weapons-grade plutonium, where up to 93% of the plutonium created is the fissile Pu-239, with somewhere between 6% and 7% Pu-240. Since one of the main concerns is preventing nations other than the established nuclear weapons-having states from obtaining them — the whole point of non-proliferation — it’s very likely that the primary concern Ernest Moniz had back in the 2015 talks with Ali Akbar Salehi (the physicist overseeing Iran’s nuclear programme) was in ensuring that any plutonium that was created was not weapons-grade.
The other main concern would be the separation of U-235 from U-238. Normal uranium ore only contains a few percent of U-235, with more than 95% of naturally-occurring uranium existing as U-238. However, it isn’t just plutonium that gets used to make fission bombs, but fissile uranium, which is heavily enriched with U-235 well above the naturally occurring levels. Nuclear physicists often talk about SWU, which stands for separative work units, or the amount of work needed to create enriched uranium. Part of the negotiations is each side estimating the efficiency and capabilities of the non-nuclear state to create that enriched uranium, with the USA having the goal of requiring at least a year of efforts for the non-nuclear state in question to create bomb-worthy materials.
Those two issues, involving the creation of enriched uranium and weapons-grade plutonium, are likely going to be at the center of any talks concerning nuclear proliferation among non-nuclear states, with extraordinary expertise required to perform the estimates and calculations accurately. If we get it right, and all sides act relatively responsibly, we could live in a world where many nations have access to the tremendous benefits that nuclear power brings, while still maintaining a level of global security that relies on those same nations not having access to nuclear bombs.
While many think this is too dangerous of a plan for planet Earth, that ship sailed way back in 1953, with Eisenhower’s Atoms For Peace plan. Ever since, part of the role of the Department of Energy has been to work with the State Department to work to prevent nuclear proliferation, which is why so many of our Energy Secretaries have been Ph.D. nuclear physicists. While the present one isn’t, that doesn’t doom us to failure in this realm; it simply means we need to ensure that the right expertise is available to do the proper calculations and make the proper policies. With the political issues affecting our nation and our world today, it’s never been more vital to do this responsibly, and to get things right. The safety and security of the world depends on it.
Send in your Ask Ethan questions to startswithabang at gmail dot com!
Ethan Siegel is the author of Beyond the Galaxy and Treknology. You can pre-order his third book, currently in development: the Encyclopaedia Cosmologica.