Ask Ethan: Could Dark Matter Not Be A Particle, At All?
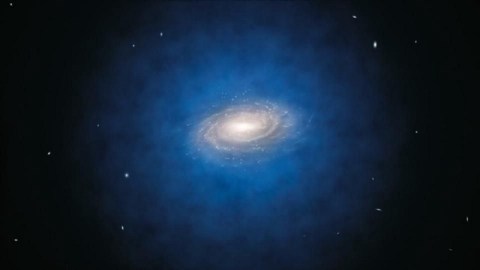
We always assume that dark matter is particle-based, and we just need to find which particle it is. But what if it isn’t so?
Everything we’ve ever detected in the Universe, from matter to radiation, can be broken down into its smallest constituents. Everything in this world is made of atoms, which are made of nuclei and electrons, where nuclei themselves are made of quarks and gluons. Light itself is made of particles: photons. Even gravitational waves, in theory, are made of gravitons: particles we may someday be able to create and detect. But what about dark matter? The indirect evidence for its existence is tremendous and overwhelming, but must it, too, be a particle? That’s what our Patreon supporter Darren Redfern wants to know, as he asks:
If dark energy can be interpreted as an energy inherent to the fabric of space itself, could it also be possible that what we perceive as “dark matter” is also an inherent function of space itself — either tightly or loosely coupled to dark energy? That is, instead of dark matter being particulate, could it permeate all of space with (homogeneous or heterogeneous) gravitational effects that would explain our observations — more of a “dark mass”?
Let’s look at the evidence, and see what it tells us about the possibilities.
One of the most remarkable features about the Universe is the one-to-one relationship between what’s in the Universe and how the expansion rate changes over time. Through a slew of careful measurements of many disparate sources — including stars, galaxies, supernovae, the cosmic microwave background, and the large-scale structure of the Universe — we’ve been able to measure both of those, determining what our Universe is made of. In principle, there are a slew of various things we can imagine that our Universe might have been made out of, all of which influence cosmic expansion differently.
Thanks to the full suite of our data, we now know that we’re made of:
- 68% dark energy, which remains at a constant energy density even as space itself expands,
- 27% dark matter, which exerts a gravitational force, dilutes as volume increases, and doesn’t measurably interact through any other known force,
- 4.9% normal matter, which exerts all the forces, dilutes as volume increases, clumps together, and is composed of particles,
- 0.1% neutrinos, which exert a gravitational and weak force, is made of particles, and clumps together only when they slow down enough to behave as matter instead of radiation,
- and 0.01% photons, which exert gravitational and electromagnetic forces, act as radiation, and dilutes as both volume increases and its wavelength gets stretched.
Over time, these various components become relatively more-or-less important, where these percentages represent what the Universe is made out of today.
Dark energy, from the best of our measurements, appears to have the same value and properties at every location in space, in all directions on the sky, and at all moments throughout our cosmic history. In other words, dark energy appears both homogeneous and isotropic: it’s the same everywhere and at all times. As well as we know it, dark energy doesn’t need to have a particle; it can easily be a property inherent to the fabric of space itself.
But dark matter is fundamentally different.
In order to form the structure that we see in the Universe, particularly on large, cosmic scales, dark matter needs to not only exist, but it needs to clump together. It cannot have the same density at every location in space; rather, it has to be concentrated in overdense regions, and needs to be below-average-density or even completely absent from underdense regions. We can actually tell how much total matter is in a variety of regions of space from a few different sets of observations. What follows are three of the most important.
1.) The Matter Power Spectrum: map out the matter in the Universe, see on what scales galaxies correlate — a measure of the likelihood of finding another galaxy a certain distance away from the one you start with — and plot it out. If you had a Universe that was made of uniform matter, the structure you’d see would be smeared out. If you had a Universe that had dark matter that didn’t clump early on, the structure on the small scales would be destroyed. This matter power spectrum teaches us that approximately 85% of the matter in the Universe is dark matter, totally distinct from protons, neutrons, and electrons, and this dark matter was born cold in temperature, or with a kinetic energy that was small compared to its rest mass.
2.) Gravitational Lensing: take a look at a massive object, like a quasar, galaxy, or cluster of galaxies, and look at how the background light gets distorted by its presence. Because we understand the laws of gravity, as governed by Einstein’s General Relativity, the way that the light bends allows us to infer how much mass is present in each object. Through a slew of other methods, we can determine the amount of mass that’s present in normal matter: stars, gas, dust, black holes, plasma, etc. Again, we find that, on average, 85% of the matter present has to be dark matter, and moreover, that it’s distributed in a more diffuse, cloud-like configuration than the normal matter is. Both weak lensing and strong lensing confirm this.
3.) The Cosmic Microwave Background: if you look at the leftover glow of radiation from the Big Bang, you’ll find that it’s roughly uniform: 2.725 K in all directions. But if you look in more granular detail, you’ll find that there are tiny imperfections on the scales of tens-to-hundreds of µK, on all sorts of angular scales. These fluctuations tell us a slew of important things, including the normal matter/dark matter/dark energy densities, but the biggest thing they tell us is how uniform the Universe was when it was just 0.003% of its current age, and the answer is that the densest region was only about 0.01% denser than the least dense region. In other words, dark matter started out uniform, and then clumped together as time went on!
Putting all of these together, we come to the conclusion that dark matter must behave like a fluid that permeates the Universe. This fluid has a negligibly small pressure and viscosity, it does respond to radiation pressure, it doesn’t collide with photons or normal matter, it was born cold and non-relativistic, and it clumps together under the force of its own gravity over time. It drives the formation of structure in the Universe on the largest scales. It is highly inhomogeneous, with the magnitude of these inhomogeneities growing over time.
That’s what we can say about it on large scales, where it’s linked to observation. On small scales, we suspect — but aren’t certain — that this is because dark matter is made up of particles with properties that cause it to behave this way on large scales. The reason we assume this is because the Universe, to the best of our knowledge, is simply composed of particles, end of story! If you’re matter, and if you have mass, you have a quantum counterpart, and that means an indivisible particle at some level. But until we directly detect this particle, there’s no way to rule out the other possibility: that this is some sort of fluidic field that is non-particle-based, but impacts spacetime the same way that an aggregate set of particles would.
That’s why attempts at direct detection are so important! As a theorist myself who wrote his Ph.D. thesis on large-scale structure formation, I’m well aware that what we can do is incredibly powerful in terms of predicting observables, particularly on large scales. But what we can’t do, theoretically, is confirm whether dark matter is a particle or not. The only way to do that is through direct detection; without it, you can have strong indirect evidence, but it won’t be bulletproof. It doesn’t seem to be coupled to dark energy in any way, since dark energy is truly uniform across space, and the predictions on large scales tell us how it interacts gravitationally and through the other forces quite accurately.
But is it a particle? Until we detect one, we can only assume the answer. The Universe has shown itself to be quantum in nature as far as every other form of matter is concerned, so it’s reasonable to assume dark matter would be as well. Keep in mind, though, that reasoning in this fashion has its limitations. After all, everything follows the same rule everything else follows, but only until they don’t anymore! We’re in uncharted territory with dark matter, and it’s important to be humble before the great unknowns in this Universe.
Send in your Ask Ethan questions to startswithabang at gmail dot com!
Ethan Siegel is the author of Beyond the Galaxy and Treknology. You can pre-order his third book, currently in development: the Encyclopaedia Cosmologica.