Ask Ethan: Could an atmosphere slow down a runaway spaceship?
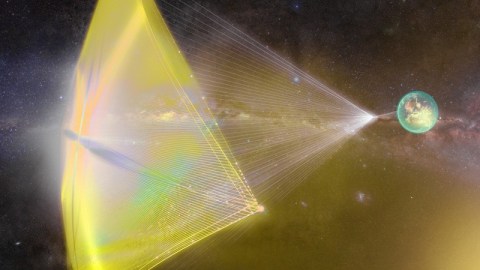
If we’re going to send a probe to another star at 20% the speed of light, what hope do we have of slowing it down?
“Yes, now there is this technological path. But it’s just starting.” –Mae Jemison
Earlier this month, Yuri Milner and Stephen Hawking teamed up to announce the Breakthrough Starshot. The plan is for a giant laser array to accelerate a very light laser sail with a spaceship-on-a-chip attached to it to more than 20% the speed of light, aimed towards one of the nearest stars to us. At these speeds, the spacecraft should arrive within a single human lifetime of its launch, an amazing feat! While there are an incredible number of technological and economic challenges to face to make this project a reality, Alex Stockton plans for success, but questions the arrival:
[M]y dad and I have been discussing the possibilities of spacecraft like the ones Yuri Milner and Steven Hawking are proposing. My dad speculated on using atmospheric drag to slow the spaceship down once it arrived at a planet. I attest that it would have no chance of slowing down appreciably and would surely result in a giant explosion. Which one is it?
After all, presumably the goal of a spacecraft that travels light years to a nearby planetary system isn’t simply to send our space junk throughout the galaxy.
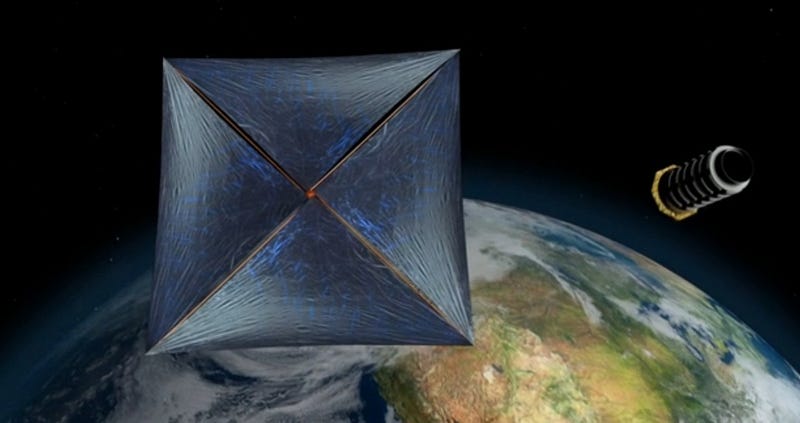
Instead, we’d like to reach a system rife with alien worlds, full of the potential to explore them, take data, and perhaps even someday return what we find back to those of us who are still here on Earth. We’ve presently learned an incredible amount about alien solar systems from our exoplanet studies from afar, but — as missions like New Horizons, Dawn and Cassini have shown even within our own Solar System — there’s no substitute for studying an alien world up close.
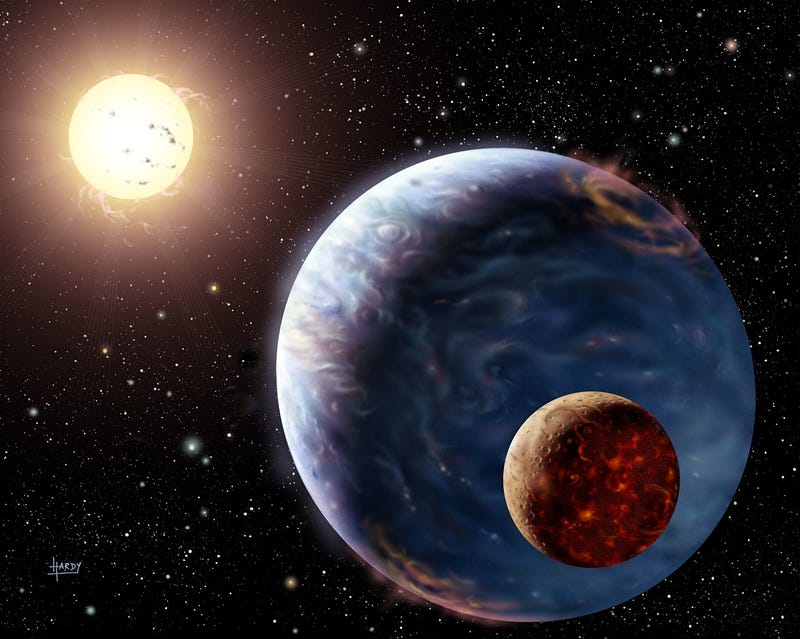
If we can get there, that’s an incredible feat in itself. If we can successfully aim ourselves and accelerate ourselves to sufficient precisions and magnitudes, we’ll be traveling somewhere in the ballpark of 60,000 km/s relative to any planet or star system we arrive at. Think about that speed for a moment: 60,000 km/s, or around 134 million miles-per-hour. If that sounds faster to you than anything else you know of, there’s a good reason for that: it is. It’s faster than any macroscopic object we know of, and is hundreds of times faster than the speeds necessary to escape from our own galaxy’s gravitational pull. If you run into even a tiny, small, diffuse region of neutral gas, the amount of heating that will occur will be simply tremendous. At thousands of times lower speeds, after all, only the most advanced heat shields ever have survived re-entry into our own atmosphere.
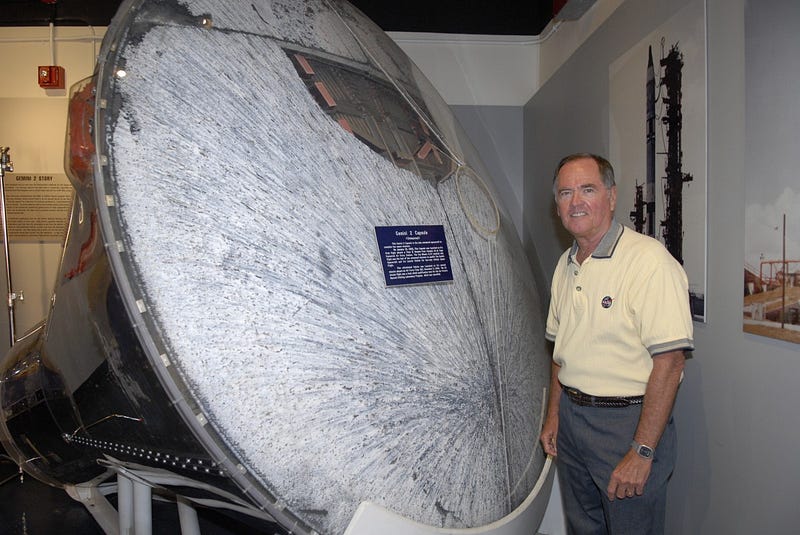
But going thousands of times faster makes the situation millions of times worse. If you’ve ever opened your car window while driving down the street, you might have noticed something interesting about the force of the wind: if you go twice as fast, you feel four times the force. Energy, friction and heating on a spacecraft suffer from that same exact problem; if you travel at twice the speed, you heat up four times as quickly, and if you travel at ten times the speed, you heat up a hundred times as fast. To understand what a Starshot spacecraft might experience in an atmosphere, I want you to consider the closest analogy to that we’ve experienced on Earth: a meteor.
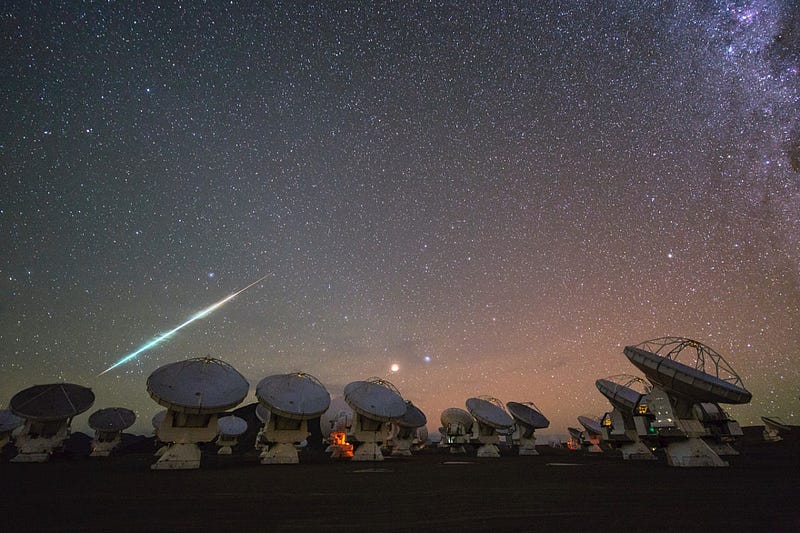
Most of the meteors that strike Earth during a meteor shower are of comparable masses to what these spacecraft are going to be: between 0.1 and 10 grams, total. The amount of kinetic energy that a meteor has, however, is proportional to the meteor’s mass but also is proportional to its speed, relative to the atmosphere, squared. These meteors move quickly: somewhere between 20 and 110 km/s, and normally burn up in the atmosphere in just a fraction of a second. Over the course of a long, glorious meteor shower, you might see tens or even hundreds of such fiery streaks in a single night.
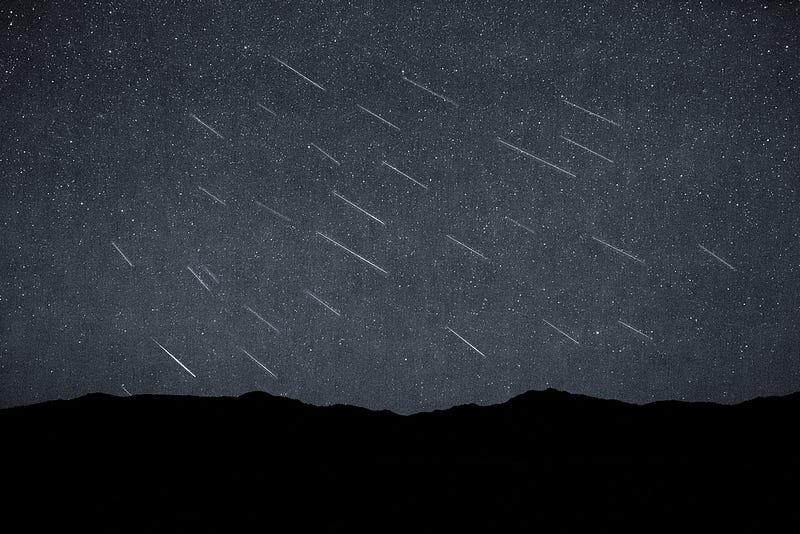
So now we come to the spacecraft: of a comparable mass but with around 1,000 times the speed of a typical meteor. That means, in terms of kinetic energy, it has about 1,000,000 times as much to deal with and dissipate as a typical meteor. A planet getting hit by a ~1 gram spacecraft moving at 60,000 km/s is going to experience the same level of catastrophic effects as a planet getting hit by a ~1 tonne asteroid moving at ~60 km/s: the equivalent of which happens on Earth just once per decade.
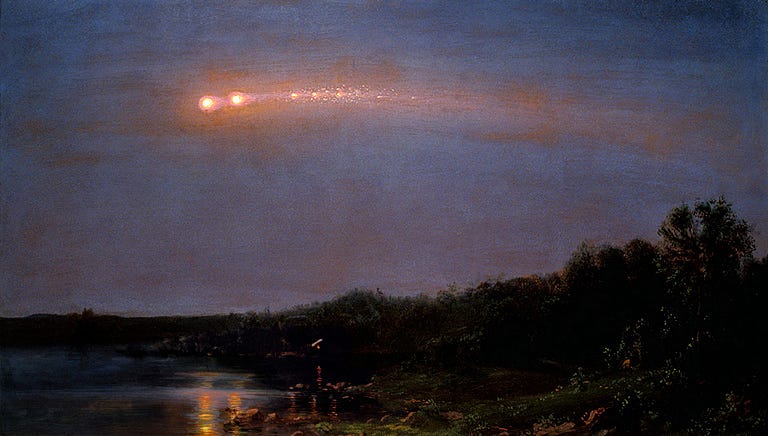
At speeds this high, the spacecraft material itself will become a plasma, with the atoms/molecules on board having their electrons stripped off from it. The spacecraft, if it’s as thin and spread-out as conceived, will disintegrate in a matter of microseconds, which is good, because it will only take approximately 1,000 microseconds for it to go from the top of an Earth-like atmosphere to the ground.
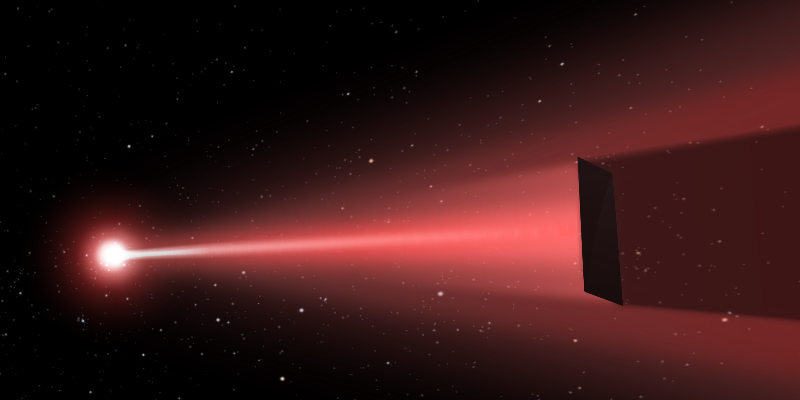
Your best hope of deceleration, if you want your spacecraft to remain intact, is to have the same type of laser array waiting for you at your destination, ready to provide your spacecraft with the same frequency of light that accelerated it in the first place. We’ve gotten excellent at designing materials that can reflect somewhere in the neighborhood of 99.999% of light at a very particular frequency, which is how a laser sail concept is plausible at all. But if you’re running into anything other than light at that frequency — including any other form of radiation or, more relevantly, matter — you’re going to absorb an awful lot of that energy. At these speeds, that means disintegration.
So, Alex, I regret to tell you and your dad both that yes, atmospheric drag will slow your spaceship down appreciably, but the way it goes about doing it results in a fiery catastrophe that should absolutely destroy everything on your spacecraft, down to the individual atoms themselves.
Submit your Ask Ethan considerations to ethan dot siegel at gmail dot com.
This post first appeared at Forbes. Leave your comments on our forum, check out our first book: Beyond The Galaxy, and support our Patreon campaign!