10 Quantum Truths About Our Universe
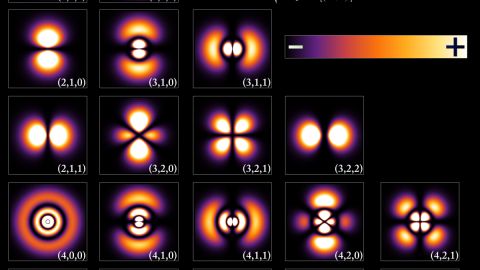
Even most of the pros don’t know all 10.
This post was contributed to Starts With A Bang by Sabine Hossenfelder. Sabine is a theoretical physicist specialized in quantum gravity and high energy physics. She also freelance writes about science.
“In fact, the mere act of opening the box will determine the state of the cat, although in this case there were three determinate states the cat could be in: these being Alive, Dead, and Bloody Furious.” –Terry Pratchett
From the moment that it was discovered that the macroscopic, classical rules that governed electricity, magnetism and light didn’t necessarily apply to the smallest, subatomic scales, a whole new view of the Universe became accessible to humanity. This quantum picture is much larger and all-encompassing than most people realize, including many professionals. Here are ten essentials of quantum mechanics that may cause you to re-examine how you picture our Universe, on the smallest scales and beyond.
1.) Everything is quantum.
It’s not like some things are quantum mechanical and others are not. Everything obeys the same laws of quantum mechanics — it’s just that quantum effects of large objects are very hard to notice. This is why quantum mechanics was a latecomer to the development of theoretical physics: it wasn’t until physicists had to explain why electrons sit on shells around the atomic nucleus that quantum mechanics became necessary to make accurate predictions.
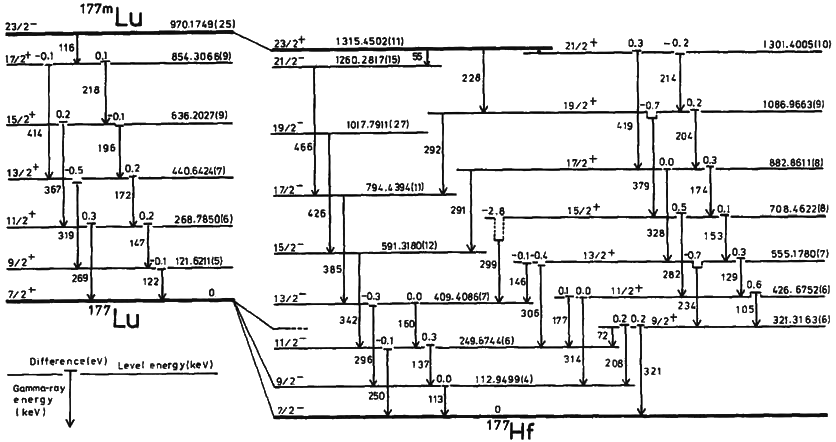
2.) Quantization doesn’t necessarily imply discreteness.
“Quanta” are discrete chunks, by definition, but not everything becomes chunky or indivisible on short scales. Electromagnetic waves are made of quanta called “photons,” so the waves can be thought of as being discretized. And electron shells around the atomic nucleus can only have certain discrete radii. But other particle properties do not become discrete even in a quantum theory. The position of electrons in the conducting band of a metal for example is not discrete — the electron can occupy any continuous location within the band. And the energy values of the photons that make up electromagnetic waves are not discrete either. For this reason, quantizing gravity — should we finally succeed at it — does not necessarily mean that space and time have to be made discrete. (But, on the other hand, they might be.)
3.) Entanglement not the same as superposition.
A quantum superposition is the ability of a system to be in two different states at the same time, and yet, when measured, one always finds a particular state, never a superposition. Entanglement on the other hand is a correlation between two or more parts of a system — something entirely different. Superpositions are not fundamental: whether a state is or isn’t a superposition depends on what you want to measure. A state can for example be in a superposition of positions and not in a superposition of momenta — so the whole concept is ambiguous. Entanglement on the other hand is unambiguous: it is an intrinsic property of each system and the so-far best known measure of a system’s quantum-ness. (For more details, read “What is the difference between entanglement and superposition?”)
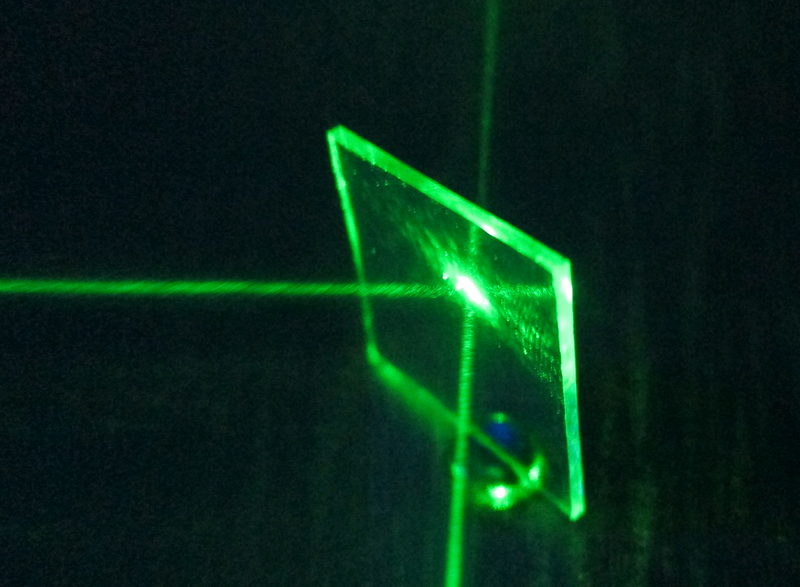
4.) There is no spooky action at a distance.
Nowhere in quantum mechanics is information ever transmitted non-locally, so that it jumps over a stretch of space without having to go through all places in between. Entanglement is itself non-local, but it doesn’t do any action — it is a correlation that is not connected to non-local transfer of information or any other observable. When you see a study where two entangled photons are separated by a great distance and then the spin of each one is measured, there is no information being transferred faster than the speed of light. In fact, if you attempt to bring the results of two observations together (which is information transmission), that information can only travel at the speed of light, no faster! What constitutes “information” was a great source confusion in the early days of quantum mechanics, but we know today that the theory can be made perfectly compatible with Einstein’s theory of Special Relativity in which information cannot be transferred faster than the speed of light.
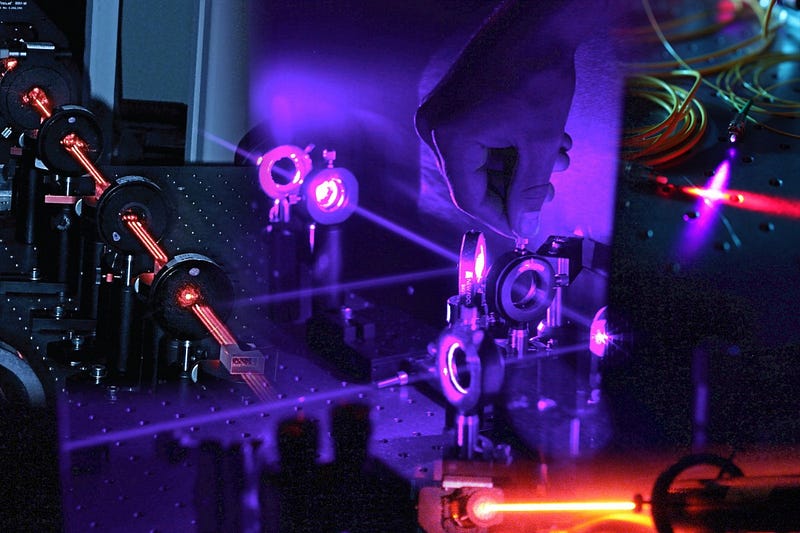
5.) Quantum physics an active research area.
It’s not like quantum mechanics is yesterday’s news. True, the theory originated more than a century ago. But many aspects of it became testable only with modern technology. Quantum optics, quantum information, quantum computing, quantum cryptography, quantum thermodynamics, and quantum metrology are all recently formed and presently very active research areas. With the new capabilities brought about by these technologies, interest in the foundations of quantum mechanics has been reignited.
6.) Einstein didn’t deny it.
Contrary to popular opinion, Einstein was not a quantum mechanics denier. He couldn’t possibly be — the theory was so successful early on that no serious scientist could dismiss it. (In fact, it was his Nobel-winning discovery of the photoelectric effect, proving that photons acted as particles as well as waves, that was one of the foundational discoveries of quantum mechanics.) Einstein instead argued that the theory was incomplete, and believed the inherent randomness of quantum processes must have a deeper explanation. It was not that he thought the randomness was wrong, he just thought that this wasn’t the end of the story. For an excellent clarification of Einstein’s views on quantum mechanics, I recommend George Musser’s article “What Einstein Really Thought about Quantum Mechanics” (paywalled, sorry).
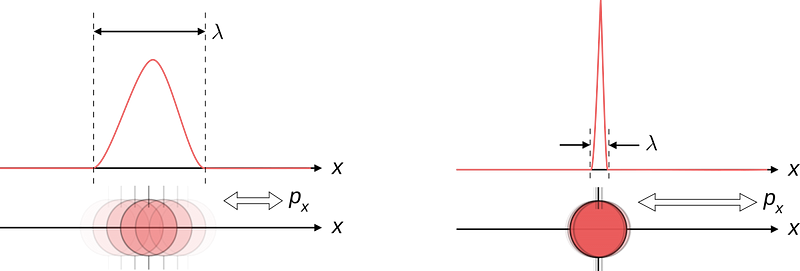
7.) It’s all about uncertainty.
The central postulate of quantum mechanics is that there are pairs of observables that cannot simultaneously be measured, like for example the position and momentum of a particle. These pairs are called “conjugate variables,” and the impossibility to measure both their values precisely is what makes all the difference between a quantized and a non-quantized theory. In quantum mechanics, this uncertainty is fundamental, not due to experimental shortcomings. One of the most bizarre manifestations of this is the uncertainty between energy and time, which means that unstable particles (with a short lifetime) have inherently uncertain masses, thanks to Einstein’s E=mc2. Particles like the Higgs boson, the W-and-Z bosons and the top quarks all have masses that are intrinsically uncertain by 1–10% because of their short lifetimes.
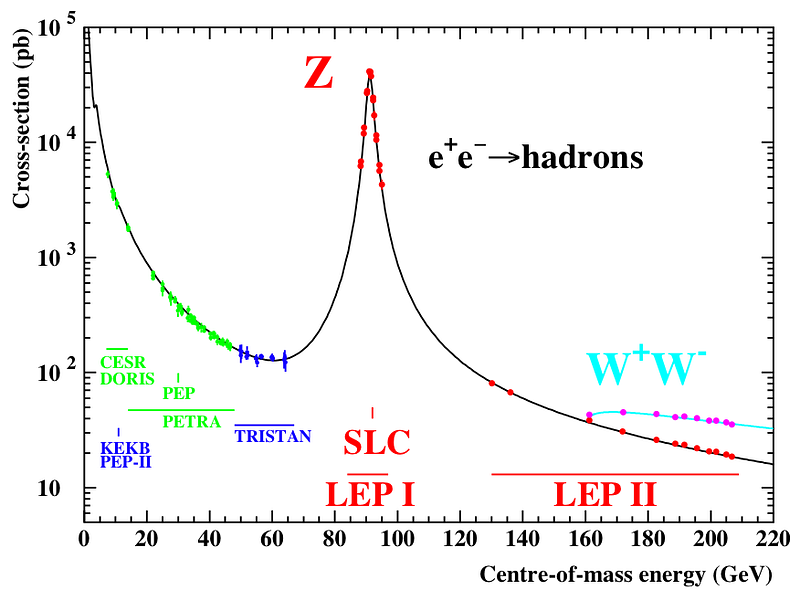
8.) Quantum effects are not necessarily small…
We do not normally observe quantum effects on long distances because the necessary correlations are very fragile. Treat them carefully enough however, and quantum effects can persist over long distances. Photons have for example been entangled over separations as much as several hundreds of kilometers. In Bose-Einstein condensates, a degenerate state of matter found at cold temperatures, up to several million of atoms have been brought into one coherent quantum state. And finally, some researchers even believe that dark matter may have quantum effects which span across entire galaxies.
9.) …but they dominate the small scales.
In quantum mechanics, every particle is also a wave and every wave is also a particle. The effects of quantum mechanics become very pronounced once one observes a particle on distances that are comparable to the associated wavelength. This is why atomic and subatomic physics cannot be understood without quantum mechanics, whereas planetary orbits are effectively unchanged by quantum behavior.
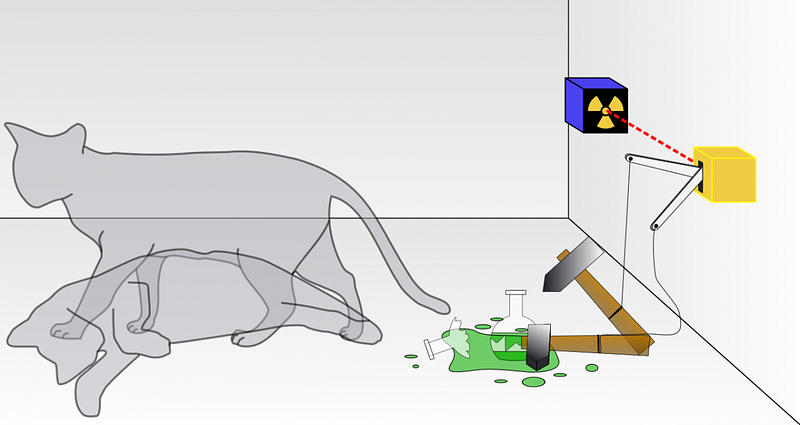
10.) Schrödinger’s cat is dead. Or alive. But not both.
It was not well-understood in the early days of quantum mechanics, but the quantum behavior of macroscopic objects decays very rapidly. This “decoherence” is due to constant interactions with the environment which are, in relatively warm and dense places like those necessary for life, impossible to avoid. This explains that what we think of as a measurement doesn’t require a human; simply interacting with the environment counts. It also explains why bringing large objects into superpositions of two different states is therefore extremely difficult and the superposition fades rapidly. The heaviest object that has so far been brought into a superposition of locations is a carbon-60 molecule, while the more ambitious have proposed to do this experiment for viruses or even heavier creatures like bacteria. Thus, the paradox that Schrödinger’s cat once raised — the transfer of a quantum superposition (the decaying atom) to a large object (the cat) — has been resolved. We now understand that while small things like atoms can exist in superpositions for extended amounts of time, a large object would settle extremely rapidly in one particular state. That’s why we never see cats that are both dead and alive.
This post first appeared at Forbes. Leave your comments on our forum, check out our first book: Beyond The Galaxy, and support our Patreon campaign!