The tiniest, most isolated galaxies grow unstoppably
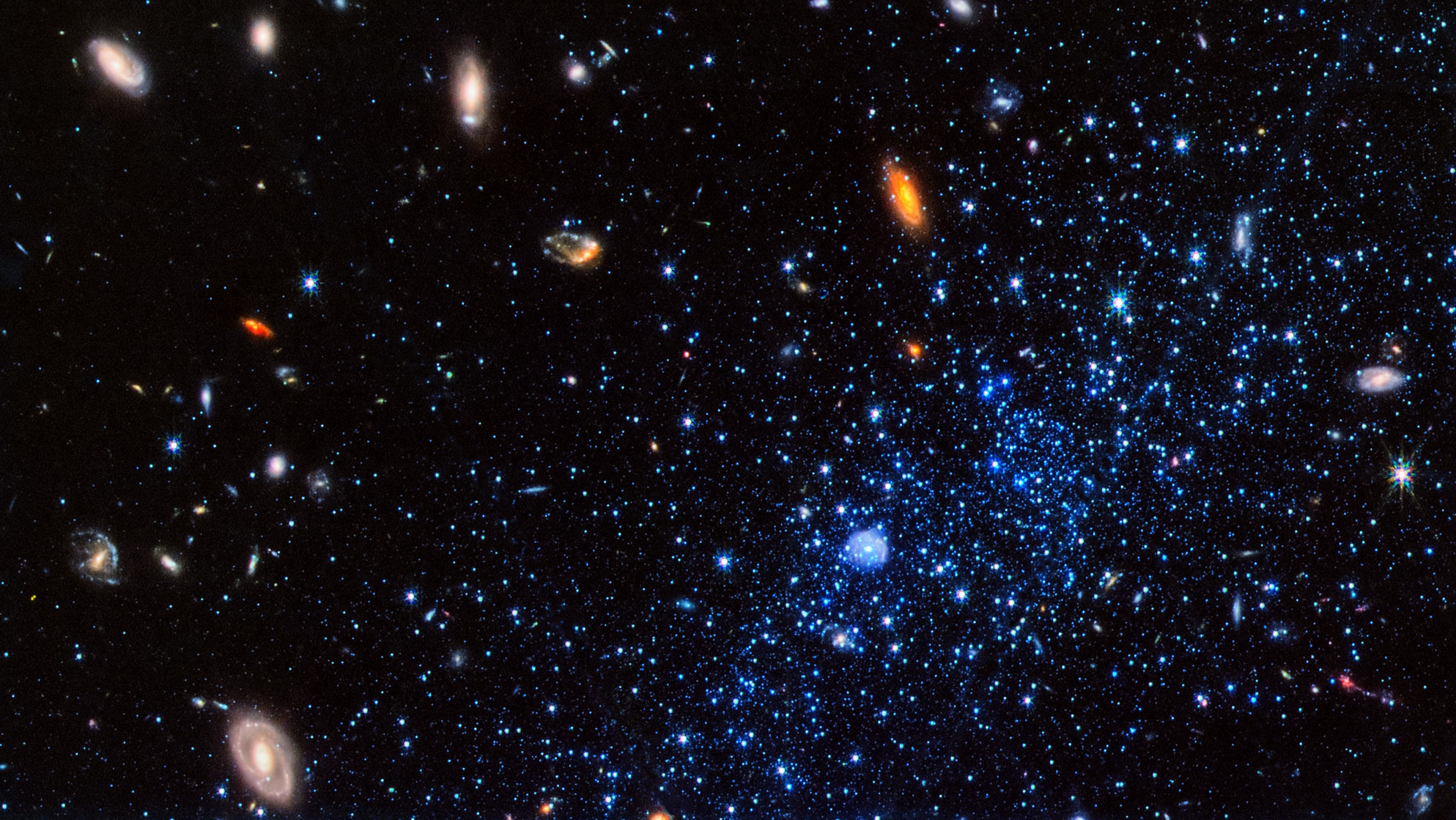
- The environment around the Milky Way, like around many large galaxies, is filled with smaller, lower-mass satellite galaxies: objects that will eventually fall into their larger neighbors.
- While they persist, they struggle to gain new matter and form new stars, as feedback and interaction with their large neighbors dominates their evolution.
- However, intergalactic space can also be very isolated, and the tiny galaxy Leo P sits alone in space. Unlike similarly sized galaxies close by, it’s continued to grow in a way we’ve never witnessed before.
Here in our cosmic neighborhood, galaxies come in all sorts of sizes, masses, and shapes. The largest, most massive galaxies in the Local Group are the Milky Way and our big sister, Andromeda: two galaxies representative of modern large spirals. While galaxies like our own are easy to find all throughout the Universe, they’re not the most common type of galaxy at all. Instead, it’s smaller, lower-mass galaxies that are far and away the most common, with an estimated 30-to-100 tiny dwarf galaxies found throughout the Universe for every one Milky Way-like galaxy out there. While the largest, brightest, most massive galaxies are the easiest ones to spot, it’s actually the smaller, more common, lower-mass galaxies that are primarily responsible for blowing away the intergalactic neutral gas and making the cosmos visible.
Some of the small galaxies that we find have large reservoirs of gas within them, where they continue to form stars throughout their lives, while others are virtually gas-free, having formed all of their stars in just one major burst that occurred long ago. However, the small galaxies that have been examined most studiously are the closest ones: the ones contained within our own Local Group, located no more than about three million light-years from us.
What would we find, then, when we examined — first with Hubble and then with JWST — a tiny, low-mass, isolated galaxy located in deep intergalactic space? Evidence for unstoppable growth that occurred over billions of years. Here’s what we learned.
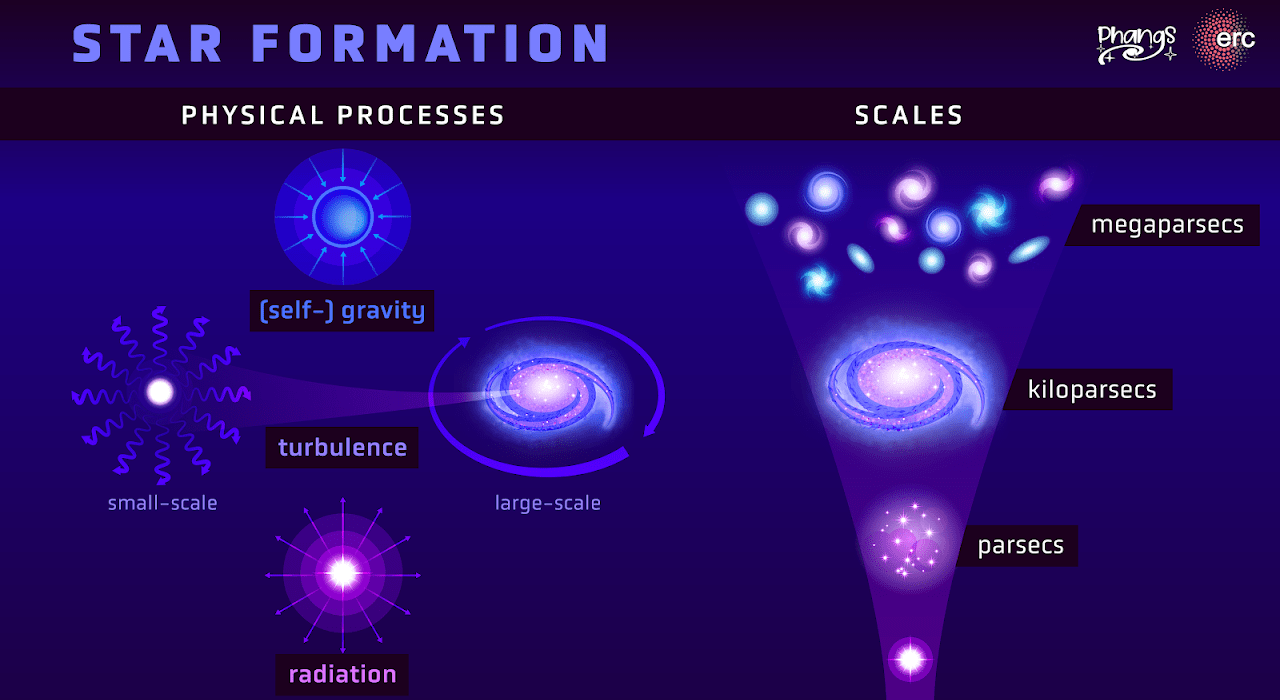
There have been a series of important lessons we’ve learned about cosmic history here in the JWST era: lessons for not merely what’s in the Universe, but for how the Universe grew up. Very early on, there were no stars or galaxies; it took tens to hundreds of millions of years for the first stars to form. Very quickly, the star-formation rate within the Universe increased, as the largest, brightest galaxies swiftly grew to contain millions, hundreds of millions, and then billions of solar masses worth of stars inside. However, these bright, early behemoths aren’t primarily responsible for producing the ultraviolet light that ionizes neutral atoms throughout intergalactic space; it’s the much more abundant low-mass galaxies that are responsible for reionizing the Universe.
When we examine the tiniest, lowest-mass galaxies found within our Local Group, we find that their evolution is greatly affected by the presence of large, nearby galaxies such as the Milky Way and Andromeda. As they speed through space, any neutral atoms (such as gas) that are present within the tiny galaxy collide with particles on the outskirts of these larger galactic halos, stripping that intervening matter away. Streams of cold gas from the space between galaxies, additionally, gets preferentially funneled into the larger, higher-mass galaxies, preventing these tiny dwarf galaxies from continuing to form stars over time.
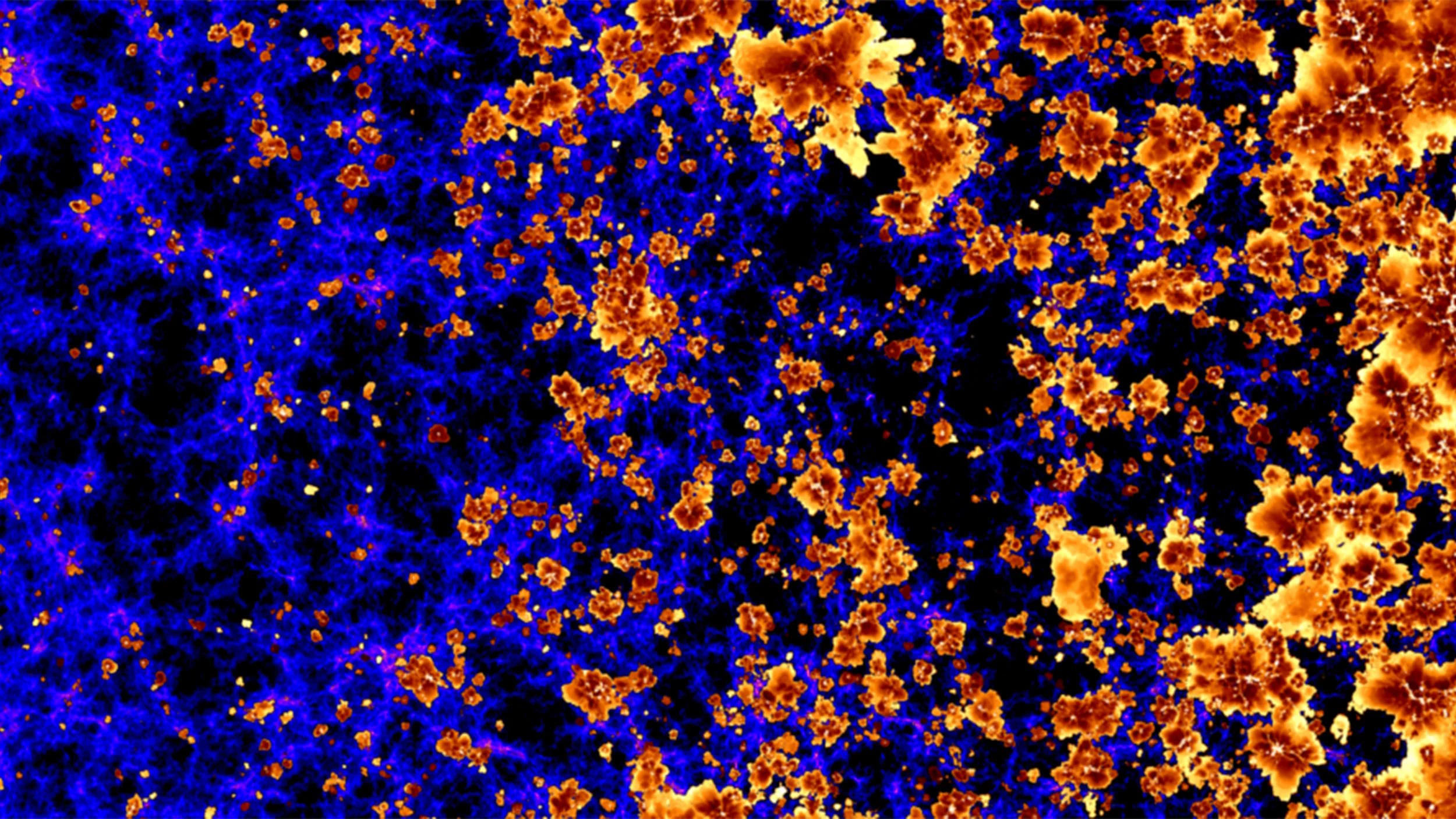
Because the Local Group is where we live, it’s the galaxies within it that are the easiest, closest ones to study. And we can measure the properties of stars and gas within those tiny, low-mass galaxies. Some of them formed stars all at once early on, and then never again in all the billions of years since. Some of them exhibit multiple populations of stars that formed at several different epochs throughout cosmic history. However, because there are large, massive galaxies nearby — again, the Milky Way and Andromeda — it’s difficult to know how things like mergers and galactic interactions affected those histories. Many confounding factors make it difficult to know why the objects we see nearby have the properties that they do.
- Did galaxies that have just one population of stars inside gain that population because they formed stars all at once and then expelled their neutral matter? Or would they have continued to form stars if they were isolated, but interactions with the large, neighboring galaxy’s halo stripped that matter away?
- Have galaxies that exhibit several populations of stars inside accreted matter from intergalactic space to feed their gas reservoirs over time, or have they resulted from multiple smaller galaxies that have merged together over time?
- And do galaxies that form stars many times do so because of internal processes alone, or were they triggered by gravitational interactions with larger neighboring galaxies?
If we want to answer these questions, we’re compelled to look at isolated low-mass galaxies: galaxies that lack these complications.

That’s where the tiny, isolated galaxy known as Leo P comes in. Located 5.3 million light-years from Earth, Leo P was only discovered relatively recently: back in 2013. Unlike galaxies within our Local Group, Leo P is located far away — about a million light-years away — from even the closest other tiny galaxies in its vicinity. Without a large halo of material nearby, or a hot region where starlight and/or star-formation occurred in great abundance, it should have experienced very few external factors that could have contributed to disrupt its natural star-forming history over cosmic time.
With only about 200,000 solar masses worth of stars inside (fewer stars than even most of the globular clusters found littered throughout the Milky Way), it’s the perfect laboratory for studying how star-formation occurred in very isolated low-mass galaxies throughout the Universe. Even though galaxies such as Leo P might be very difficult to find and study in the nearby Universe, it’s galaxies such as this one that are thought to be the ones primarily responsible for producing the ultraviolet light when the Universe was only about ~500 million years old. Since that’s the epoch in cosmic history where the Universe becomes reionized — i.e., when the neutral atoms formed shortly after the Big Bang became ionized, allowing starlight to stream freely throughout space — studying the history of galaxies such as this is of paramount importance.

This galaxy, Leo P, is in many ways very similar to what we know as ultra-faint dwarf galaxies: a population of galaxies that might have as few as several hundred stars within it, but can have up to a couple of hundred thousand stars as well. They aren’t like globular clusters in the sense that globular clusters have little-to-no dark matter within them, as their dynamics are dominated by stars and other forms of normal matter (i.e., stuff formed from protons, neutrons, and electrons). When we observe these ultra-faint dwarf galaxies, we find that the stars within them move around very quickly: much more quickly than the amount of stars inside them would allow.
With such fast speeds for the stars and so little stellar mass inside, they must be dominated by dark matter. On a cosmic scale, dark matter outmasses normal matter by a 5-to-1 ratio, and this ratio appears in most places: in the cosmic web, in galaxy clusters, in groups of galaxies, and in individual large, massive galaxies. But as we go to lower mass galaxies, we find that the dark matter-to-normal matter ratio appears to increase precipitously. The ultra-faint dwarf galaxies are the most extreme, with ratios of several hundred to one, thought to be caused by star-formation “kicking” the normal matter out of these objects, leaving only the stars and the normal matter behind.
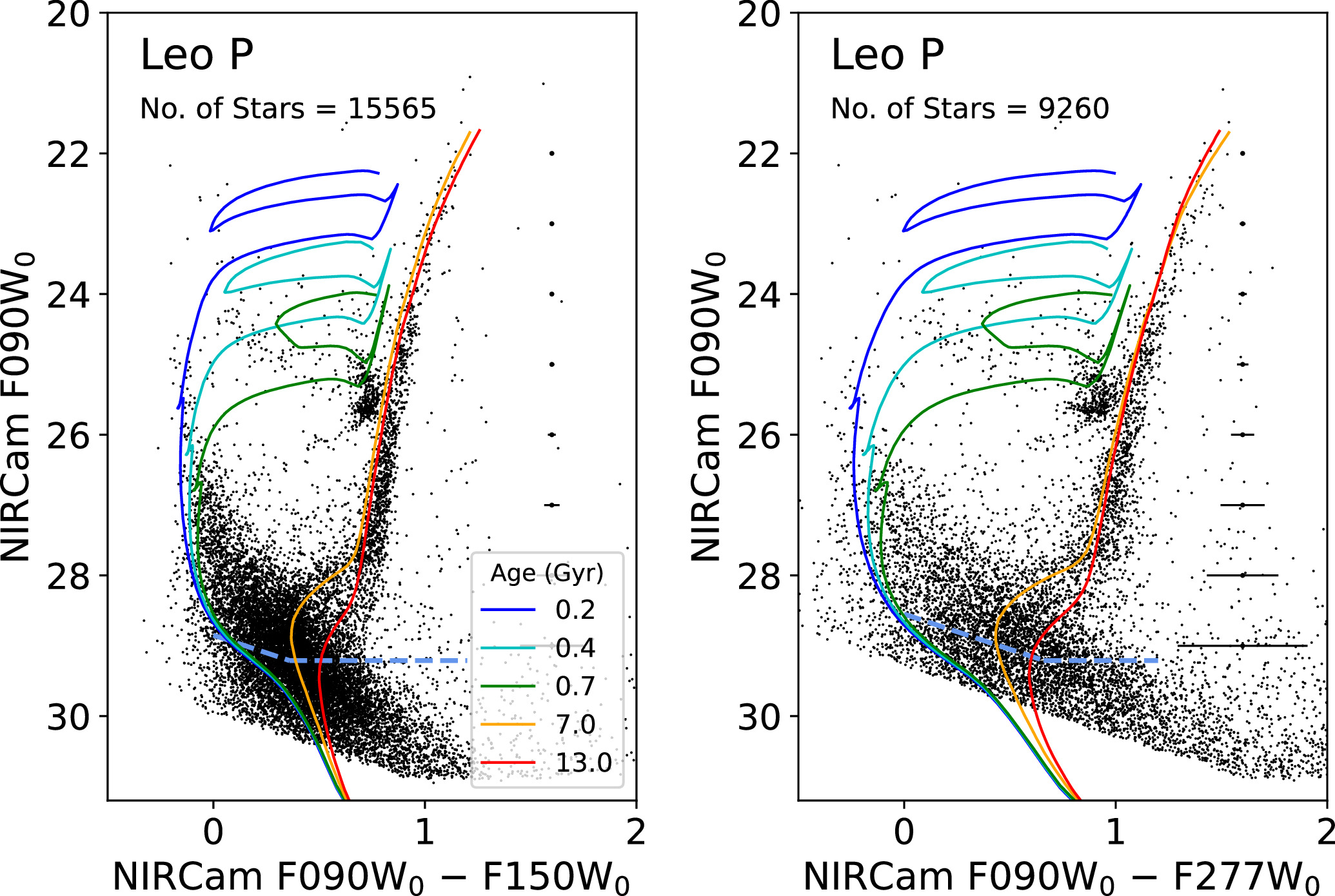
So what would we find, as far as its history went, as we looked to the galaxy Leo P with our modern arsenal of space telescopes? 5.3 million light-years may seem like a far distance to us, but to a telescope like Hubble or JWST, it’s close enough that we can resolve individual stars inside this galaxy. When Hubble viewed this galaxy years ago, it was able to teach us that the stars in this galaxy aren’t all old; instead, it found evidence for relatively recently-formed populations of stars. Hubble excels at revealing the brightest, bluest stars, which are also the shortest-lived stars. The fact that it saw a large population of bright blue stars tells us that star-formation didn’t occur all at once, long ago, and then never occurred again. Instead, it indicates a history where star-formation occurred in several bursts, including one or more bursts that occurred only in the recent past.
But JWST, with its:
- larger aperture,
- higher resolution,
- and infrared-optimized capabilities,
is adept at measuring older, redder, fainter populations of stars inside galaxies such as this one. Although we had strongly suspected that there would be a mix of old-and-young stellar populations inside, it was JWST data that was required for unveiling the full star-formation history of this galaxy, showing us when it did — and importantly, when it didn’t — form stars within it over the course of its life.
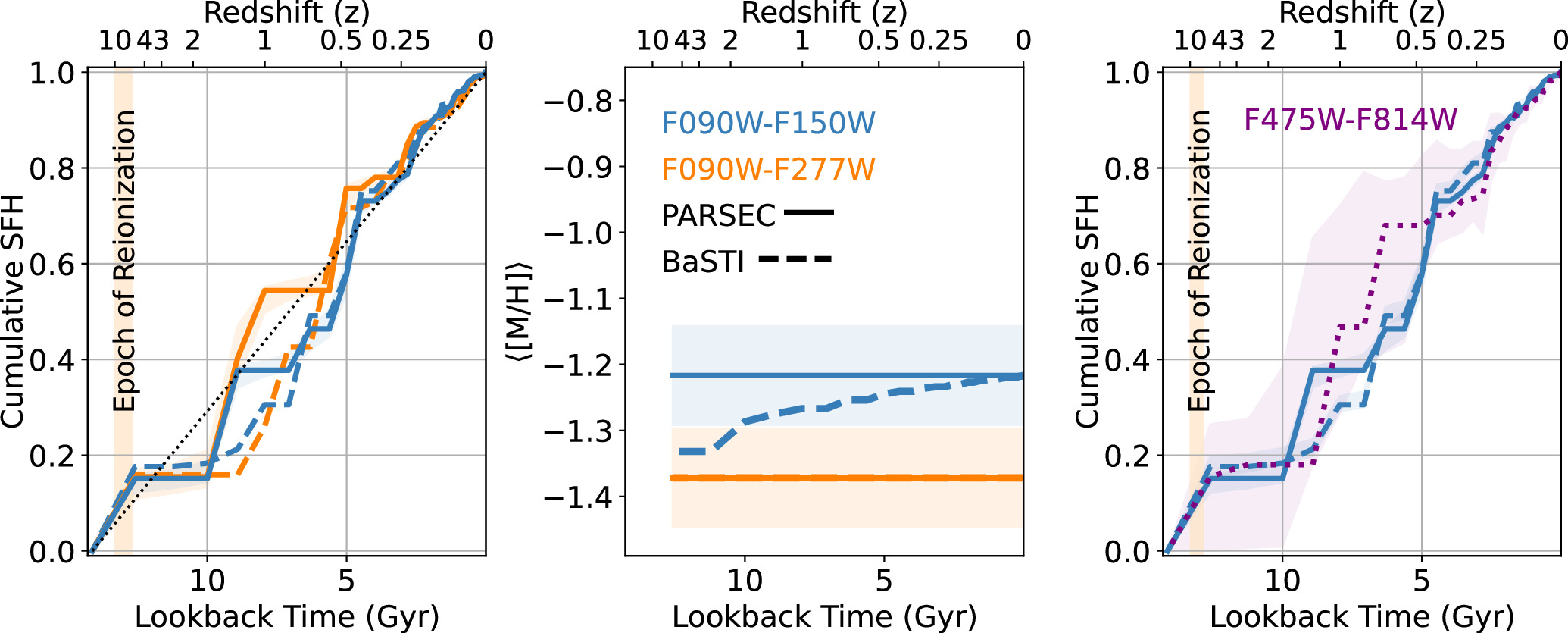
What was found inside was remarkable. As you can see from the above chart, there are several different stellar populations inside. In chronological order:
- the first population formed ~500-600 million years after the Big Bang, representing only about 15-20% of the stars found inside,
- then everything was quiet, with no star-formation at all, for the next 3-4 billion years,
- and then another, larger, longer-lasting burst of star-formation occurred, spanning a couple of billion years and forming about twice as many stars as the original burst,
- and then after another quiet period, with another 3-4 million years of inactivity, star-formation picked up again,
- with a massive burst occurring about 5 billion years ago (bigger, slightly, than the first original starburst),
- and then with star-formation gradually continuing to trickle in steadily over the past 5 billion years.
Now, what’s most remarkable about this is how different it is from the star-formation history we find in nearby ultra-faint dwarf galaxies. Either they all form stars in one burst, early on, and then never again, or they exhibit a rich history of star-formation, but only if their masses are much greater. In other words, something must be happening within this isolated galaxy that doesn’t happen for galaxies within our Local Group, or for any of the other ultra-faint dwarf galaxies that we know of.
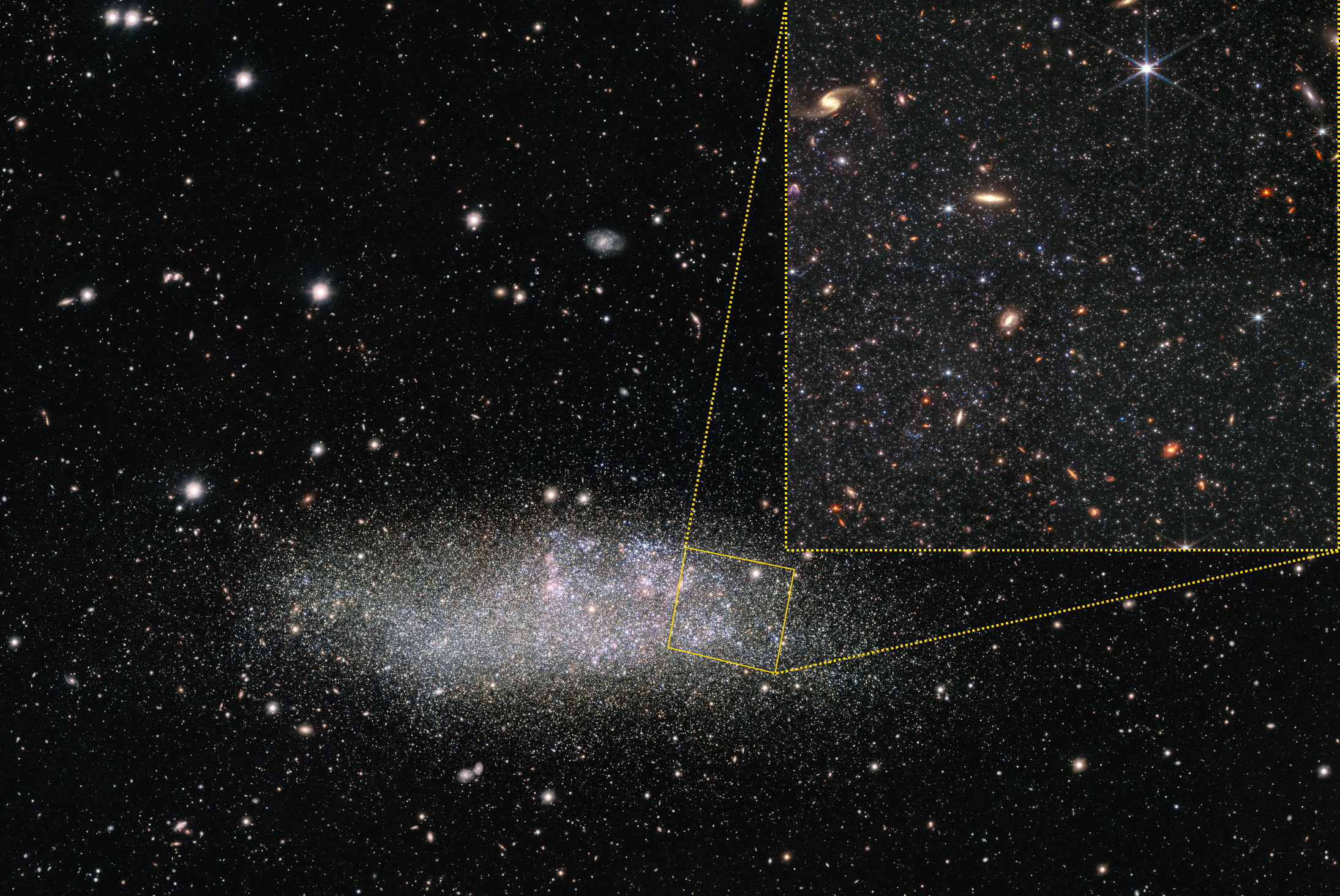
So how can we explain this?
Perhaps the leading idea is to remember how structure formation works in the Universe. Initially, it’s the regions of greatest overdensity — where the actual density exceeds the cosmic average density by the greatest amount — that gravitationally collapse first. This applies to galaxies of all sizes and masses: big ones, intermediate sized ones, small ones, and the tiniest ones of all.
But here’s where the difference comes in.
If your tiny galaxy is in the vicinity of other galaxies, including high-mass galaxies, they’re going to attract one another to give rise to a galaxy group. Within that group, the most massive galaxies are going to come to dominate: they’ll sink to the center, they’ll attract and accrue the smaller mass galaxies, and when matter from the intergalactic medium falls into that galaxy group, it’ll be the most massive galaxies that absorb most-to-all of it. In other words, if a tiny galaxy is found with other, larger galaxies nearby, it’s the largest galaxies that are going to dominate. Much like a kitten placed in a pride of lions, the smallest galaxies miss out on a cosmic feast, even when there’s an abundance of matter.
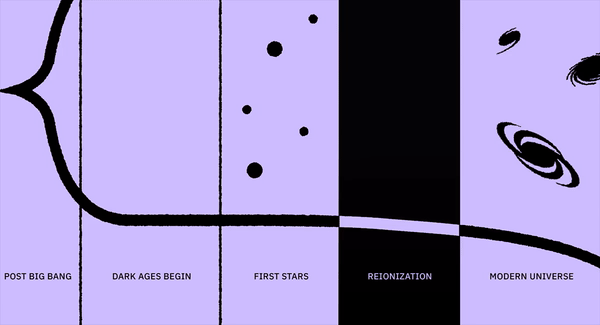
However, if your tiny galaxy is isolated, there are no other competitors for that infalling intergalactic matter. There is no ram pressure stripping; there are no tidal interactions; there is no “hot halo” of gas that prevents gravitational infall or collapse onto a smaller, lower-mass object. Instead, Leo P, because of its cosmic isolation, had that initial phase of star-formation that kicked out the remaining gas within it, leading to an early population of stars: the same type of population that likely existed in these tiny, dwarf galaxies that reionized the Universe back during the first half-a-billion years of cosmic history.
But then, because of its isolation, intergalactic matter gradually began to accrue onto this galaxy: rebuilding its stores of gas over time. Eventually, after billions of years of growth, enough matter accumulated that a molecular cloud of gas collapsed, triggering a new burst of star-formation: a larger one than the initial one. The cycle repeated itself, and after another few billion years of quiet, star-formation ignited for a third time, and then continued quietly and steadily: a process that’s still ongoing today.
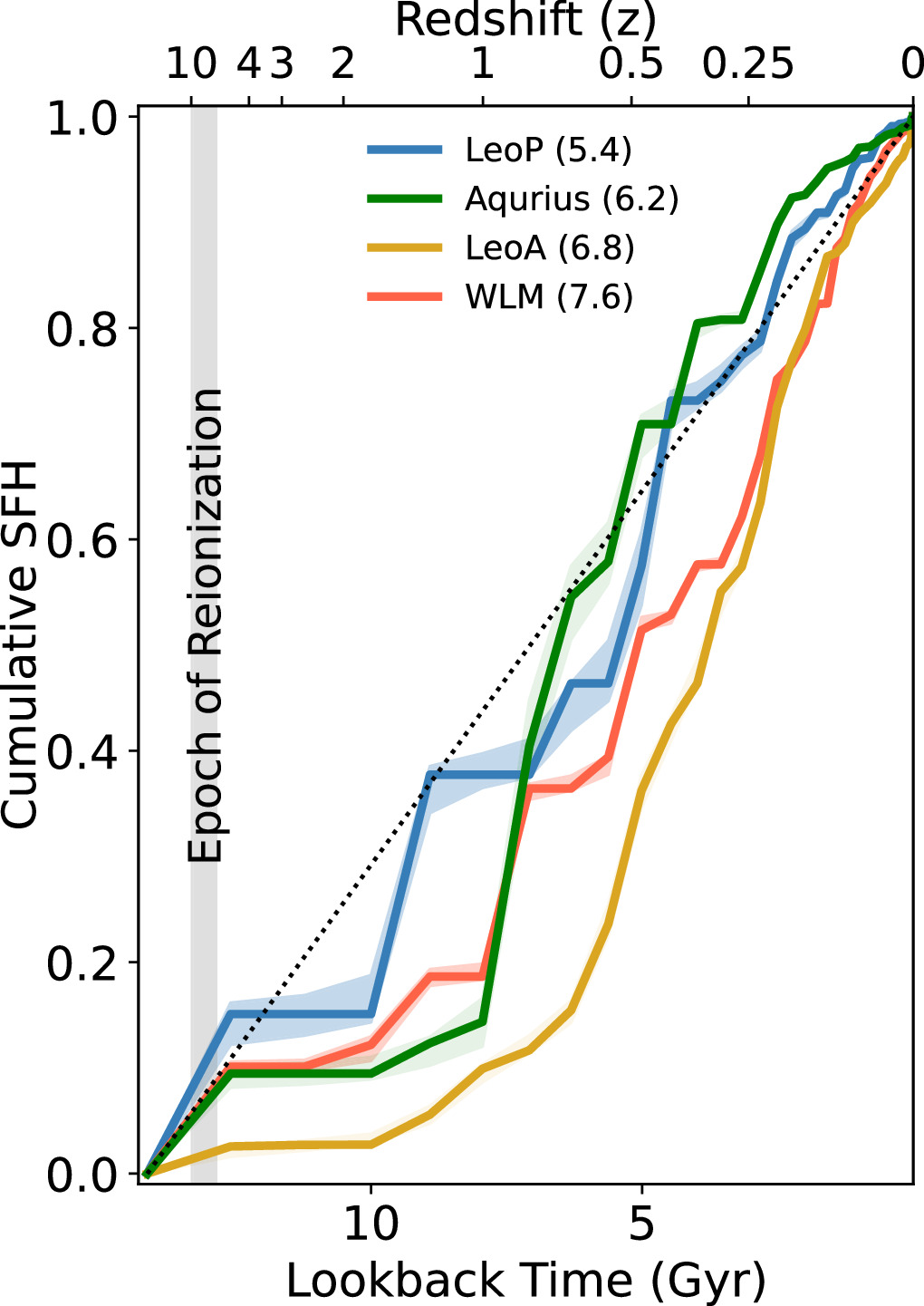
When we put all of these pieces together — what happened inside this galaxy combined with what happened in other ultra-faint dwarf galaxies, plus what we already know about the epoch of reionization — we start to arrive at a unified picture for how the tiniest galaxies in the Universe grow up. Initially, there are few enough galaxies in the Universe, and little enough time for them to merge, that these ultra-faint dwarf galaxies are the most common type: with a mass of no more than a few hundred thousand solar masses. They gravitate, collapse, form stars, and it’s the light from those stars that reionize the Universe. In most cases, that burst of star-formation provides enough feedback to prevent future star-formation from occurring, in ultra-faint dwarfs like this and perhaps in even more massive galaxies.
But then, the evolution of any one particular ultra-faint dwarf depends mightily on its environment. If it gets drawn into a galaxy group or cluster, it likely won’t be able to accrue any more star-forming material, and will be “frozen in” as a relic from the past. Its larger, more massive neighbors will act like territorial bullies, gobbling up any infalling matter and leaving practically none for the smaller satellites. In isolation, however, it can continue to grow and flourish, where new reservoirs of gas will lead to star-formation billions of years in the future, in both bursts and in a gradual steady stream, depending on the particulars of its history. In a rich group, star-formation ceases for the tiniest galaxies. But in isolation, ongoing star-formation is all but guaranteed.