The Ten Greatest Steps Of The Last Ten Decades
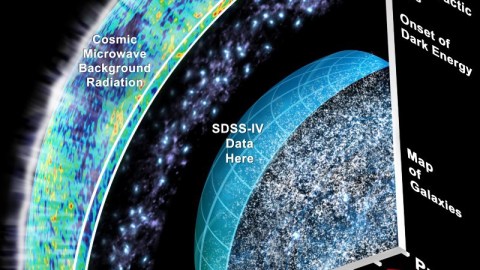
How we went from our Milky Way, alone, to the entire Universe.
“Gamow was fantastic in his ideas. He was right, he was wrong. More often wrong than right. Always interesting; … and when his idea was not wrong it was not only right, it was new.” –Edward Teller
As 2016 dawns, it’s important to recognize that just a century ago, our perception of the Universe was that:
- the stars, star clusters and nebulae in our Milky Way made up the entire Universe,
- all matter was made up of atomic nuclei and electrons,
- the only two forces were gravitation and electromagnetism,
- and Newtonian gravity, which had ruled the Universe since the 1600s, was only two months into its challenge from Einstein’s General Relativity.
Yet over the next 100 years, one great discovery per decade would reshape our perception of the Universe.
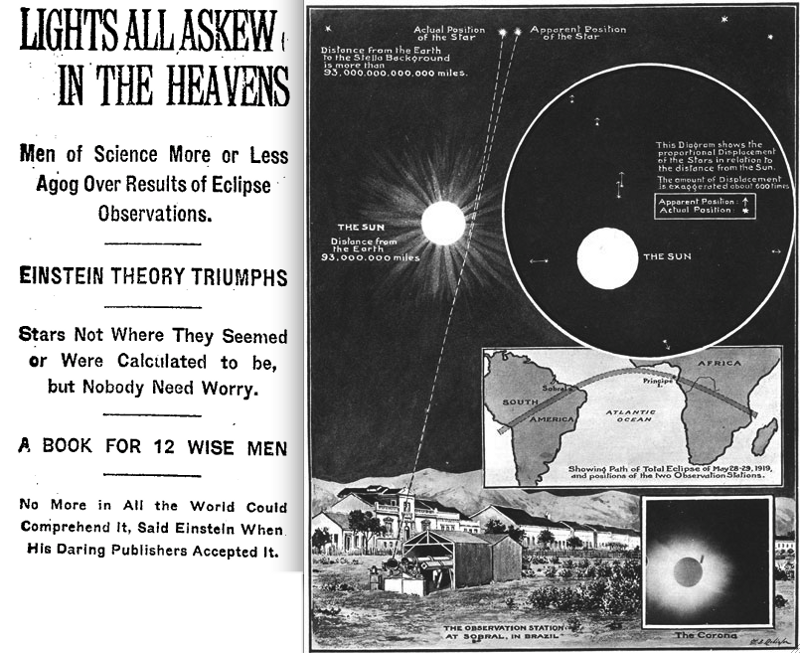
1910s — Einstein’s theory confirmed! General Relativity was famed for making the explanation that Newton’s gravity couldn’t: the precession of Mercury’s orbit around the Sun. But it isn’t enough for a scientific theory to explain something we’ve already observed; it needs to make a prediction about something that’s yet to be seen. While there have been many over the past century — gravitational time dilation, strong and weak lensing, frame dragging, gravitational redshift, etc. — the first was the bending of starlight during a total solar eclipse, observed by Eddington and his collaborators in 1919. The observed amount of bending of starlight around the Sun was consistent with Einstein and inconsistent with Newton. Just like that, our view of the Universe would change forever.
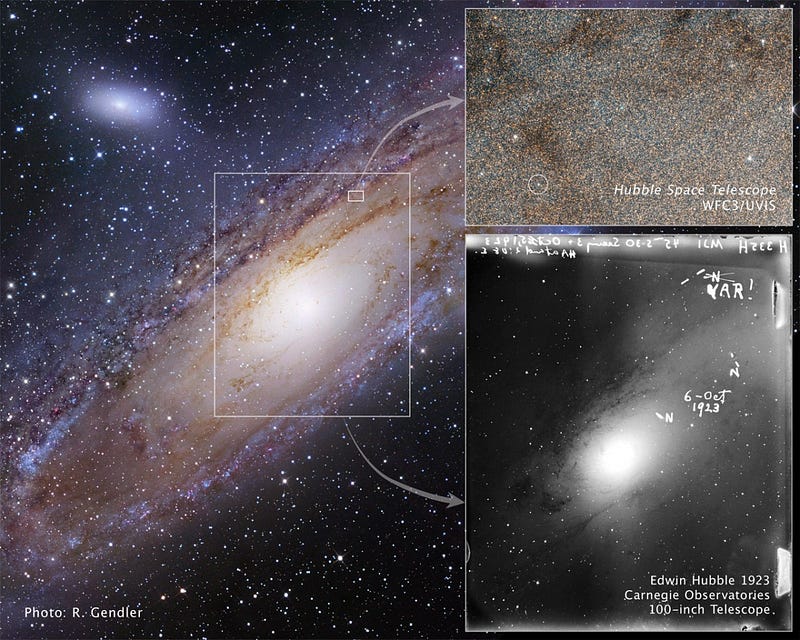
1920s — We still didn’t know there was a Universe out there beyond the Milky Way, but that all changed in the 1920s with the work of Edwin Hubble. While observing some of the spiral nebulae in the sky, he was able to pinpoint individual, variable stars of the same type that were known in the Milky Way. Only, their brightness was so low that they needed to be millionsof light years away, placing them far outside the extent of our galaxy. Hubble didn’t stop there, measuring the recession speed and distances for over a dozen galaxies, discovering the vast, expanding Universe we know today.
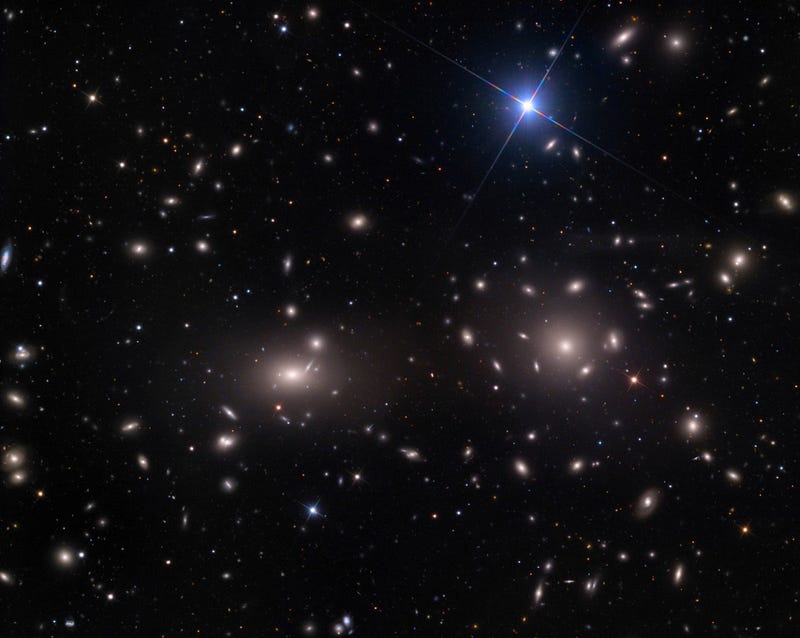
1930s — It was thought for a long time that if you could measure all the mass contained in stars, and perhaps add in the gas and dust, you’d account for all the matter in the Universe. Yet by observing the galaxies within a dense cluster (like the Coma cluster, above), Fritz Zwicky showed that stars and what we know as “normal matter” (i.e., atoms) was insufficient to explain the internal motions of these clusters. He dubbed this new matter dunkle materie, or dark matter, an observation that was largely ignored until the 1970s, when normal matter was better understood, and dark matter was shown to exist in great abundance in individual galaxies. We now know it to outmass normal matter by a 5:1 ratio.
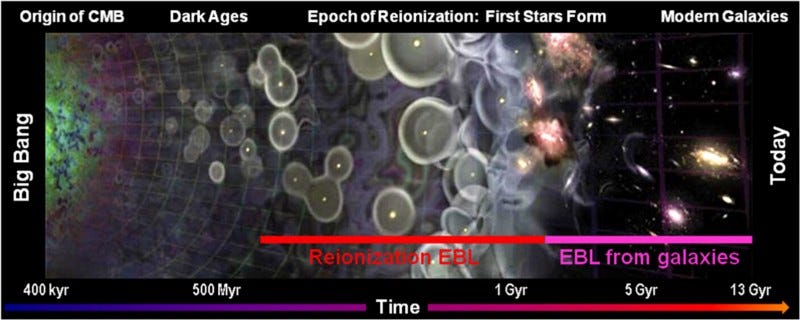
1940s — While the vast majority of experimental and observational resources went into spy satellites, rocketry and the development of nuclear technology, theoretical physicists were still hard at work. In 1945, George Gamow made the ultimate extrapolation of the expanding Universe: if the Universe is expanding and cooling today, then it must have been hotter and denser in the past. Going backwards, there must have been a time where it was so hot and dense that neutral atoms couldn’t form, and before that where atomic nuclei couldn’t form. If this were true, then before any stars ever formed, that material the Universe began with should have a specific ratio of the lightest elements, and there ought to be a leftover glow permeating all directions in the Universe just a few degrees above absolute zero today. This framework is today known as the Big Bang, and was the greatest idea to come out of the 1940s.
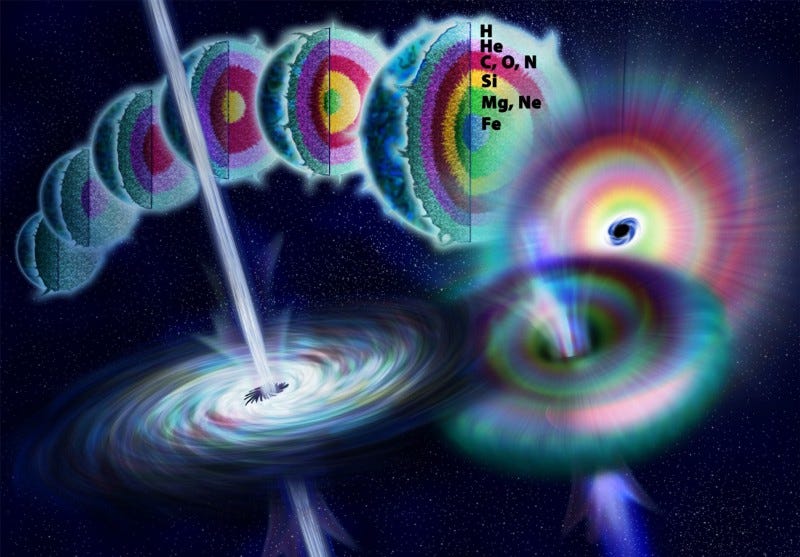
1950s — But a competing idea to the Big Bang was the Steady-State model, put forth by Fred Hoyle and others during the same time. But what was most spectacular is that they argued that all the heavier elements present on Earth today were formed not during an early, hot and dense state, but rather in previous generations of stars. Hoyle, along with collaborators Willie Fowler and Geoffrey and Margaret Burbidge, detailed exactly how elements would be built up the periodic table from nuclear fusion occurring in stars. Most spectacularly, they predicted helium fusion into carbon through a process never before observed: the triple-alpha process, requiring a new state of carbon to exist. That state was discovered by Fowler a few years after it was proposed by Hoyle, and is today known as the Hoyle State of carbon. From this, we learned that all the heavy elements existing on Earth today owe their origin to previous generations of stars.
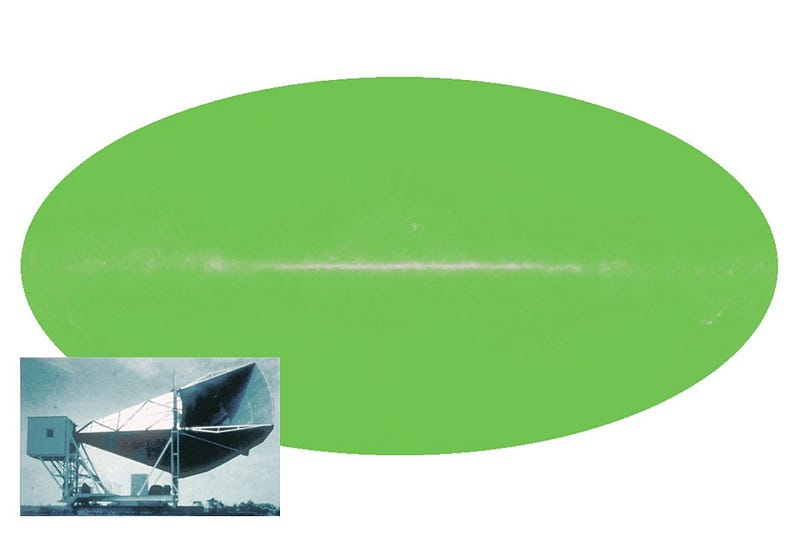
1960s — After some 20 years of debate, the key observation that would decide the history of the Universe was uncovered: the discovery of the predicted leftover glow from the Big Bang, or the Cosmic Microwave Background. This uniform, 2.725 K radiation was discovered in 1965 by Arno Penzias and Bob Wilson, neither of whom realized what they had discovered at first. Yet over time, the full, blackbody spectrum of this radiation and even its fluctuations were measured, showing us that the Universe started with a “bang” after all.
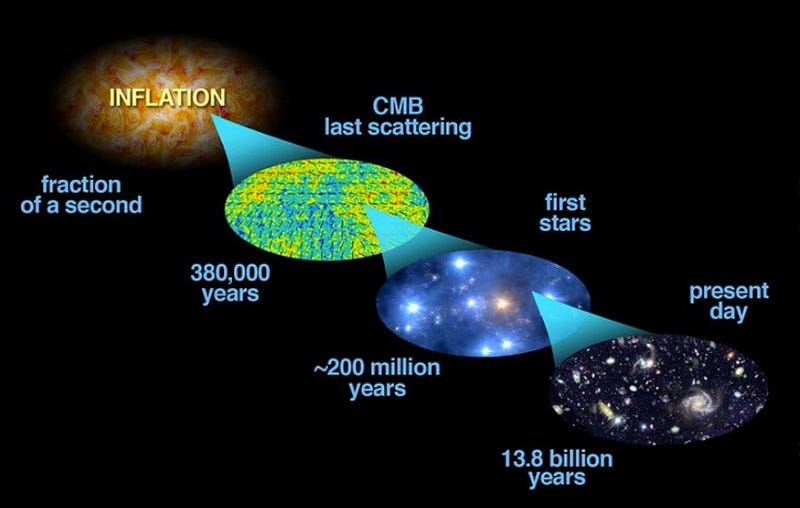
1970s — At the very end of 1979, a young scientist had the idea of a lifetime. Alan Guth, looking for a way to solve some of the unexplained problems of the Big Bang — why the Universe was so spatially flat, why it was the same temperature in all directions, and why there were no ultra-high-energy relics — came upon an idea known as cosmic inflation. It says that before the Universe existed in a hot, dense state, it was in a state of exponential expansion, where all the energy was bound up in the fabric of space itself. It took a number of improvements on Guth’s initial ideas to create the modern theory of inflation, but subsequent observations — including of the fluctuations in the CMB, of the large-scale structure of the Universe and of the way galaxies clump, cluster and form — all have vindicated inflation’s predictions. Not only did our Universe start with a bang, but there was a state that existed before the Big Bang ever occurred.
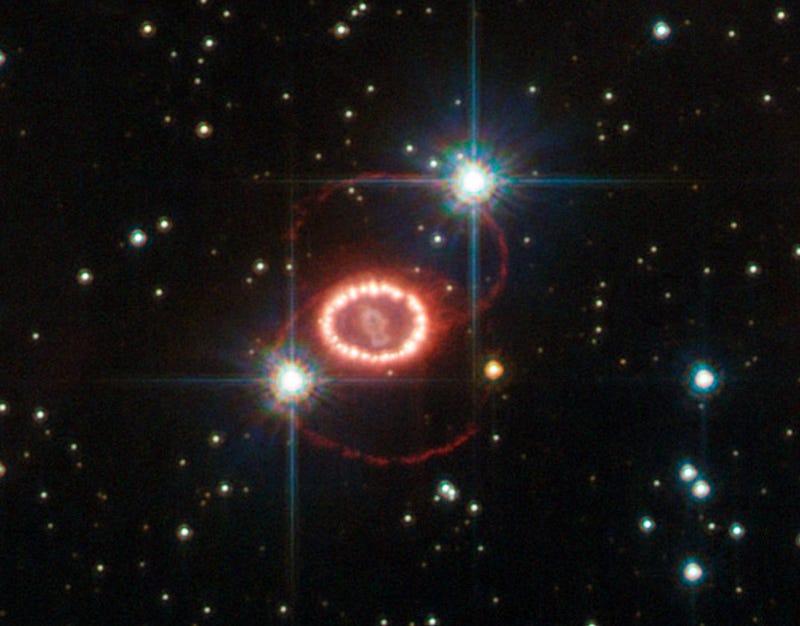
1980s — It might not seem like much, but in 1987, the closest supernova to Earth occurred in over 100 years. It was also the first supernova to occur when we had detectors online capable of finding neutrinos from these events! While we’ve seen a great many supernovae in other galaxies, we had never before had one occur so close that neutrinos from it could be observed. These 20-or-so neutrinos marked the beginning of neutrino astronomy, and subsequent developments have since led to the discovery of neutrino oscillations, neutrino masses, and neutrinos from supernovae occurring more than a million light years away. The next supernova within our galaxy will have over a hundred thousand neutrinos detected from it.
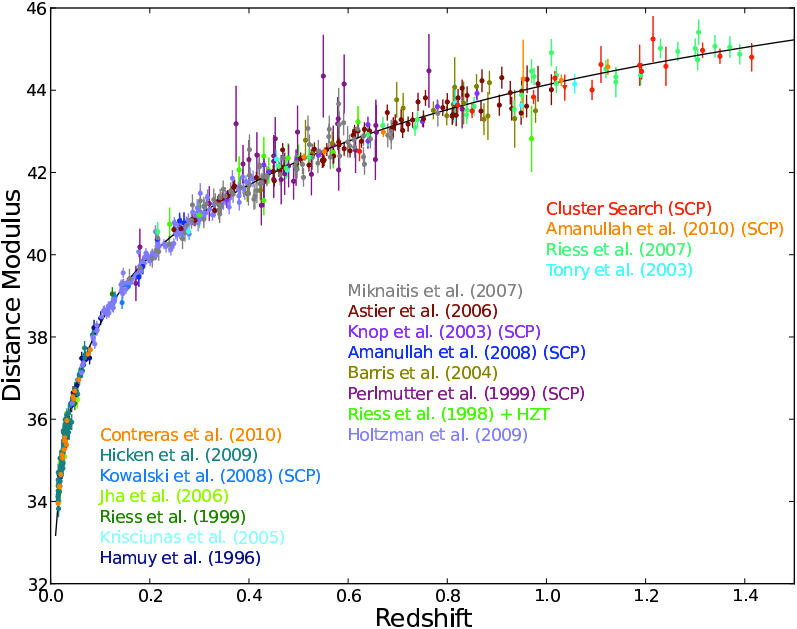
1990s — If you thought dark matter and discovering how the Universe began was a big deal, then you can only imagine what a shock it was in 1998 to discover how the Universe was going to end! We historically imagined three possible fates:
- That the expansion of the Universe would be insufficient to overcome everything’s gravitational pull, and the Universe would recollapse in a Big Crunch.
- That the expansion of the Universe would be too great for everything’s combined gravitation, and everything in the Universe would run away from one another, resulting in a Big Freeze.
- Or that we’d be right on the border between these two cases, and the expansion rate would asymptote to zero but never quite reach it: a Critical Universe
Instead, though, distant supernovae indicated that the Universe’s expansion was accelerating, and that as time went on, distant galaxies were increasing their speed away from one another. Not only will the Universe freeze, but all the galaxies that aren’t already bound to one another will eventually disappear beyond our cosmic horizon. Other than the galaxies in our local group, no other galaxies will ever encounter our Milky Way, and our fate will be a cold, lonely one indeed. In another 100 billion years, we’ll be unable to see any galaxies beyond our own.
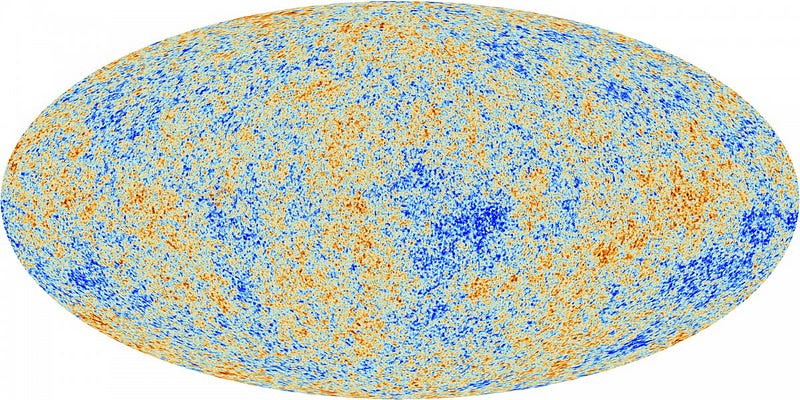
2000s — The discovery of the Cosmic Microwave Background didn’t end in 1965, but our measurements of the fluctuations (or imperfections) in the Big Bang’s leftover glow taught us something phenomenal: exactly what the Universe was made of. Data from COBE was superseded by WMAP, which in turn has been improved upon by Planck. In addition, large-scale structure data from big galaxy surveys (like 2dF and SDSS) and distant supernova data has all combined to give us our modern picture of the Universe:
- 0.01% radiation in the form of photons,
- 0.1% neutrinos, which contribute ever so slightly to the gravitational halos surrounding galaxies and clusters,
- 4.9% normal matter, which includes everything made of atomic particles,
- 27% dark matter, or the mysterious, non-interacting (except gravitationally) particles that give the Universe the structure we observe, and
- 68% dark energy, which is inherent to space itself.
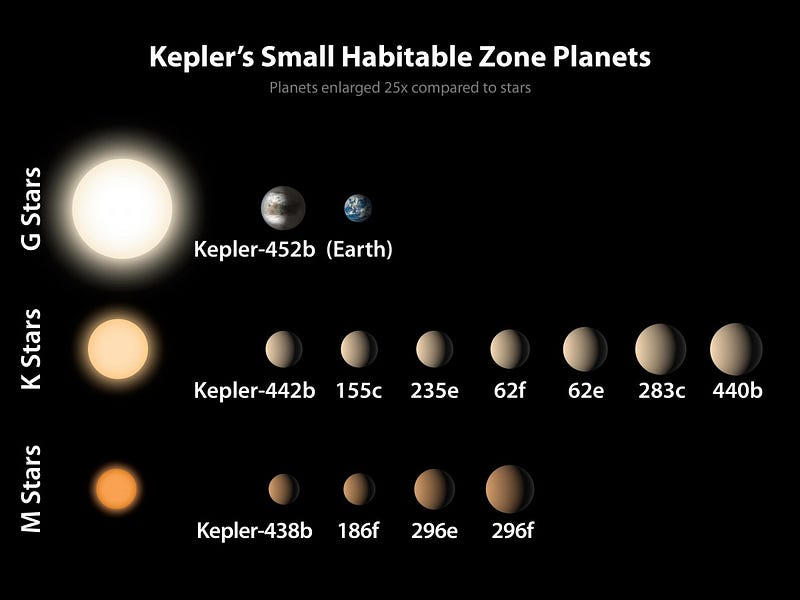
What will the 2010s hold as its greatest discovery? Will it usher in gravitational wave astronomy? Will we discover what dark matter actually is? Will the last great prediction of inflation be confirmed? Or will we find the first evidence of life beyond Earth in the Universe?
One thing is for certain: as 2016 dawns, our understanding of the Universe is limited only by the resources we invest in discovering it.
Leave your comments on our forum, and check out our first book: Beyond The Galaxy, available now, as well as our reward-rich Patreon campaign!