What’s Going On With The Rarest Stars In The Universe?
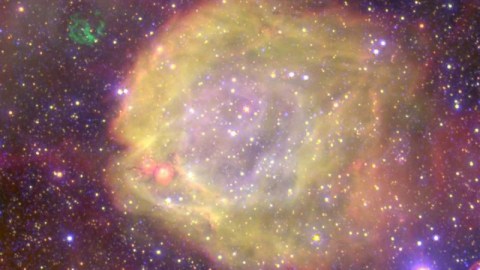
Most stars obey very similar rules, making them almost entirely predictable. But then, there are the weirdos. Catch this live-blog event to learn more.
When we look out at the Universe with our most powerful telescopes, we often think about distant galaxies at the astrophysical limits of what we can perceive. In each one, on average, are hundreds of billions of stars, each with their own one-of-a-kind history. But if we want to learn about what stars are out there, we have to look close by. Only in our own relatively nearby cosmic backyard, in the Milky Way and other galaxies no more than a few million light years away, can we resolve individual stars in detail. Thanks to tremendous surveys like Hipparcos, Pan-STARRS and the ongoing Gaia mission, we’ve been able to measure and categorize literally millions upon millions of stars. When we look at what we find, there are a few general things that most of them have in common. And then, beyond those, there are the outliers.
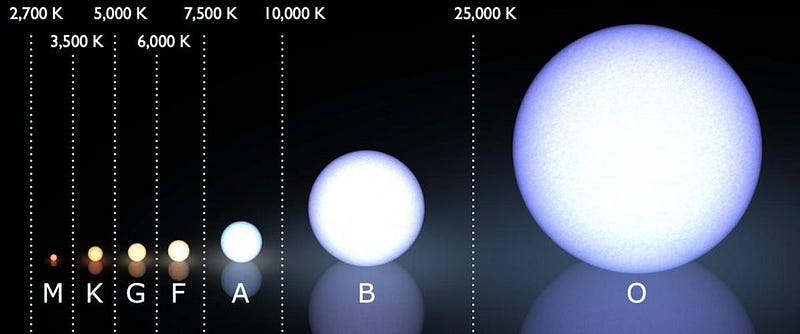
Typically, whenever you form stars, they arise from the collapse of a molecular cloud of gas. The cloud fragments, forming a wide variety of stars: large numbers of low-mass stars, smaller numbers of higher-mass stars, and if the gas cloud is large enough, still smaller but possibly significant numbers of high-mass stars. All of the stars will fuse hydrogen into helium, which is how they create the nuclear energy that powers them. Normally, we break stars like this up into seven different classes, with M-class being the smallest, lowest-mass, reddest and coolest, and O-class being the largest, most-massive, bluest and hottest stars.
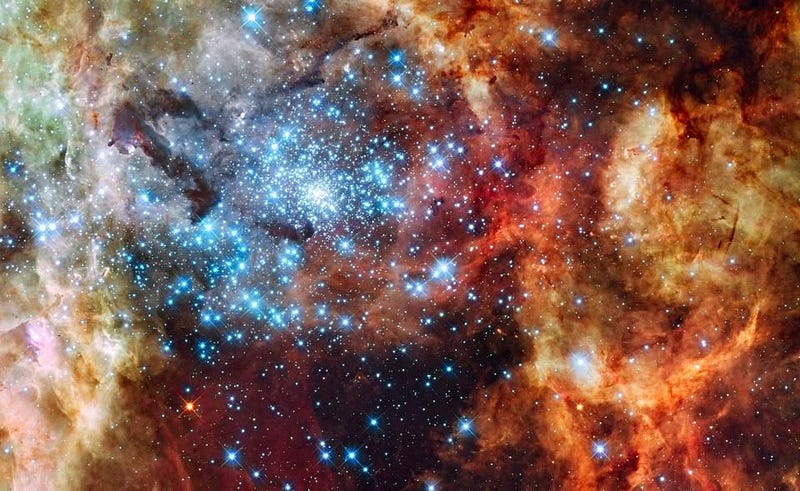
If this were all we had — these types of stars in isolation — then we think we know how they’d all evolve. Individual stars would grow as large as possible from the molecular clouds they formed from, cooling from their elements, heating up from gravitational collapse, growing until radiation pressure from the internal processes like fusion created an upper limit. Then:
- The lowest-mass M-class stars, up until about 40% of the Sun’s mass, would burn hydrogen into helium slowly, eventually dying by contracting into a helium white dwarf.
- Mid-range K-class through B-class stars, from about 40% to 800% of the Sun’s mass, burn hydrogen into helium, then heat up to fuse helium into carbon, becoming a red giant, and finally dying in a planetary nebula accompanied by a carbon/oxygen white dwarf.
- And the highest-mass stars, including the heaviest B-class and the O-class stars, will go beyond helium fusion into stages like carbon burning, oxygen burning, and all the way to silicon burning, leading to a supernova with either a neutron star or black hole at their cores.
This, at least, is our typical picture of stellar evolution.
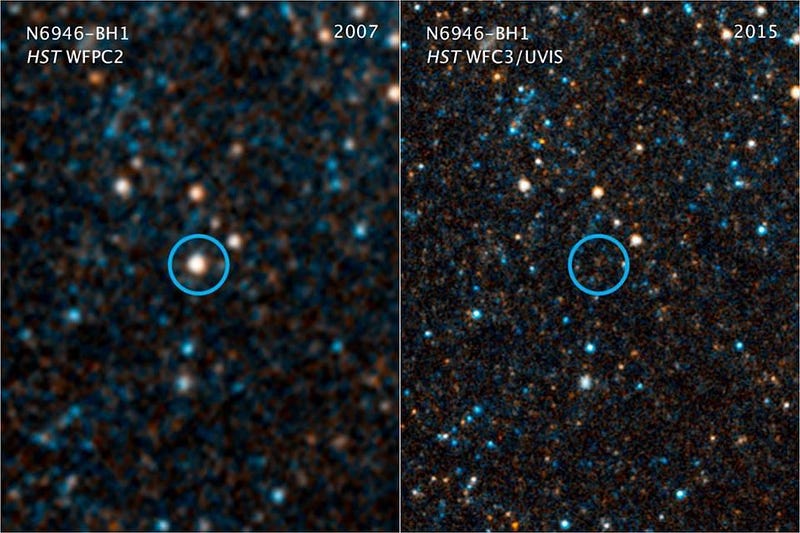
But then there are the weirdos. There are the supermassive stars that collapse directly to black holes, with no supernovae. There are the stars that get so hot that they start spontaneously producing electron/positron pairs on the inside, leading to a special kind of supernova.
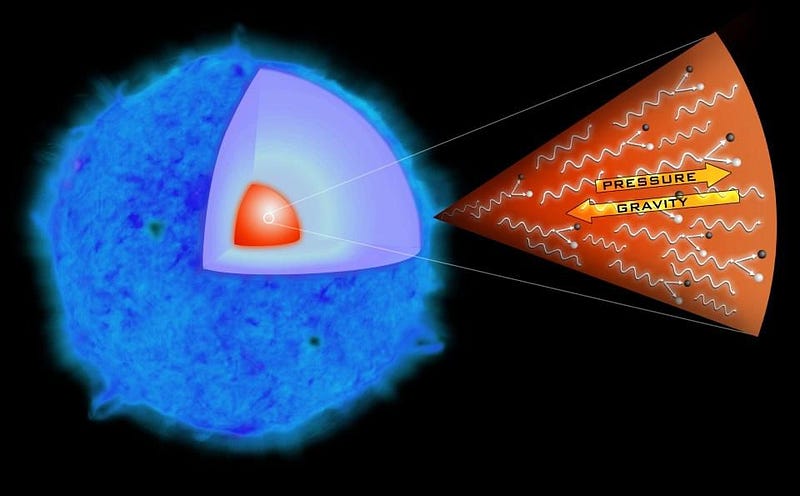
There are binary stars that steal mass off of one of the members, sometimes siphoning off all the massive hydrogen from a giant star. There are stars that should have a collapsed object at the center of a still-alive giant star, known as a Thorne-Zytkow object. There are stars, young-and-old, that exhibit extremely rare flaring behavior, like Herbig-Haro objects or Wolf-Rayet stars.
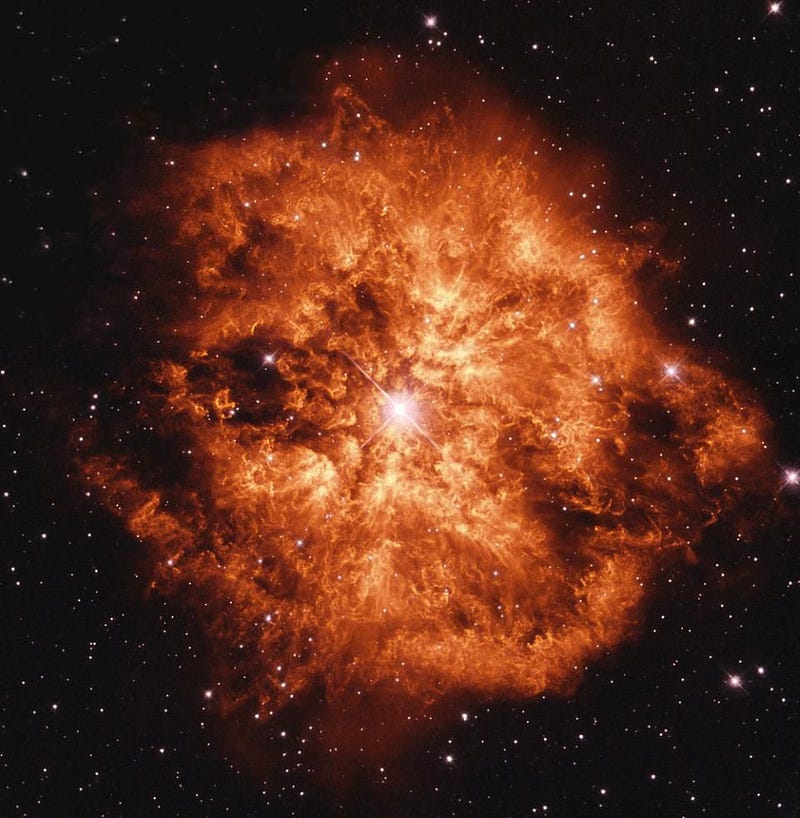
And, yet unconfirmed, there are stars made completely from pristine clouds of gas, composed solely of hydrogen and helium: the first stars in the Universe. Stars from this era may reach as much as 1,000 solar masses, and will hopefully be revealed by the James Webb Space Telescope, which was built — in part — to decipher the Universes secrets from exactly this early stage.
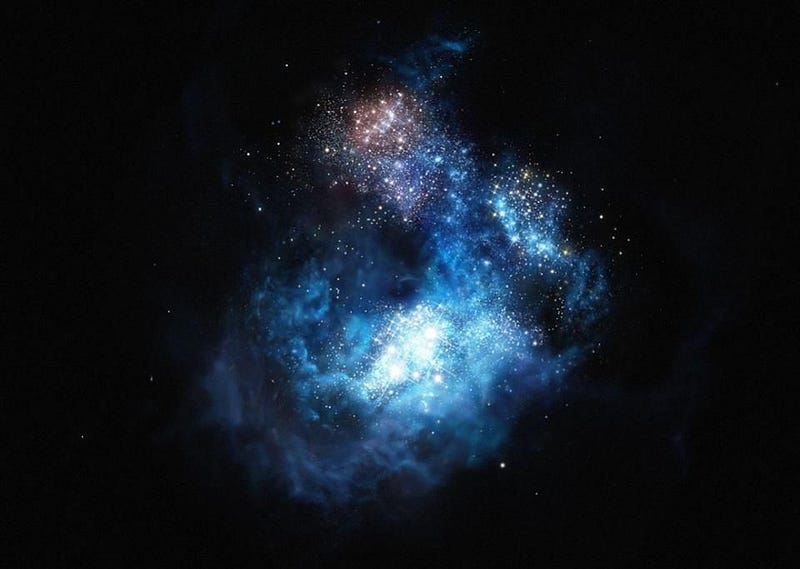
So what do we know so far? And what do we expect to find out about these weird and wild objects in the near future? That’s the subject of Emily Levesque’s public lecture, on The Weirdest Objects In The Universe, at the Perimeter Institute, from March 7th, at 7:00 PM ET/4:00 PM PT. You can, at any time, tune in right here to watch it:
And follow along, below, as I’ll be live-blogging it! Feel free to follow along and live-tweet any questions with the hashtag #piLIVE. You won’t want to miss it!
(Live blog begins at 3:50 PM. All times given in Pacific Time.)
3:50 PM: Welcome, everyone! I’ve been very excited about this talk, because I don’t know which rare/weird stars Emily will be talking about. For the first time, I don’t know what the subject of a public lecture that I’m live blogging will be, for perhaps the first time ever. It puts me in a unique situation, and I guess I’ll have to be ready for anything!
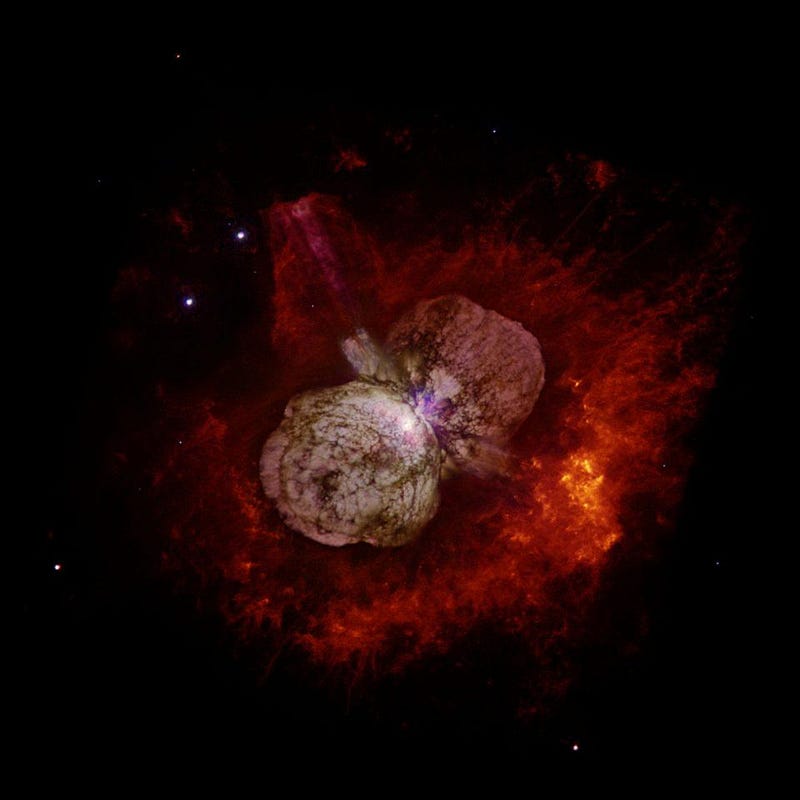
3:53 PM: For example, will we be talking about events that happen in ultramassive stars towards the ends of their lives? Will we touch on bizarre things that may be really uncommon, like supernova impostors (above)?
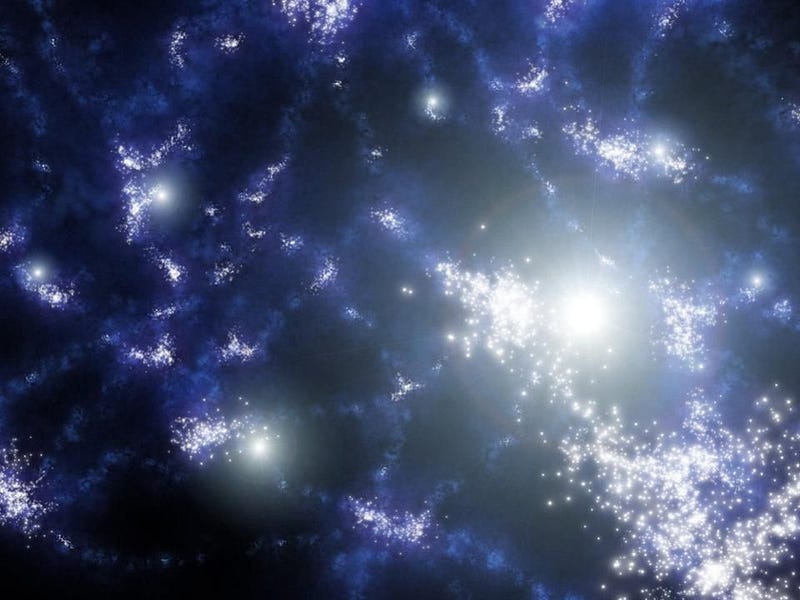
3:56 PM: Or will it focus more on the first stars in the Universe: the kind we’re struggling but hoping to detect, the ones made of pristine elements? There are so many things we don’t yet know about stars, including how, exactly, they form at a variety of stages.
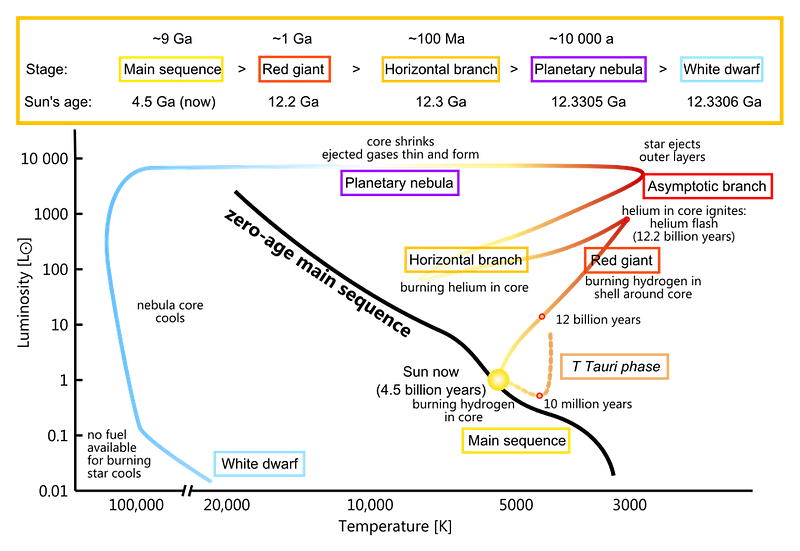
4:00 PM: Or, perhaps, are we going to be talking about the short-lived, and hence, rare-and-weird, stages in a star’s potential life? Or, just maybe, Emily will cover it all. No matter what, it’s time to get excited; it’s about to start!
4:03 PM: Emily is being introduced, and wow… is her list of awards and fellowships she’s already won enough to make anyone feel inadequate. Remember, we’re not the imposters, it’s the failed supernovae that are the imposters!
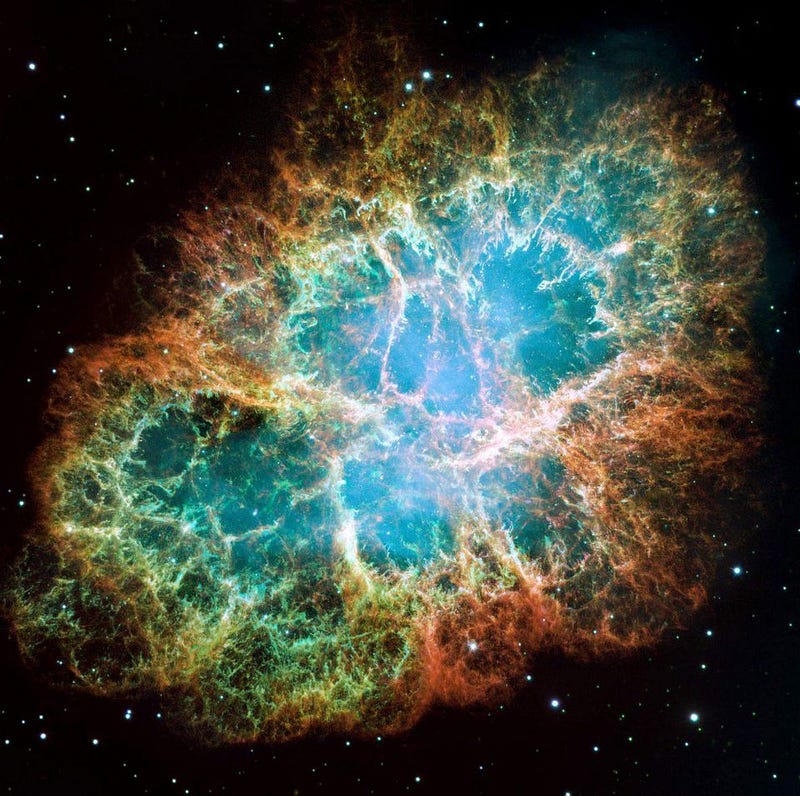
4:05 PM: Well, this is assuring… Emily is saying that we will actually be talking about “weird” objects that I’ve mostly seen or heard of before, like the Crab supernova remnant or, as we showed you above, Eta Carinae.
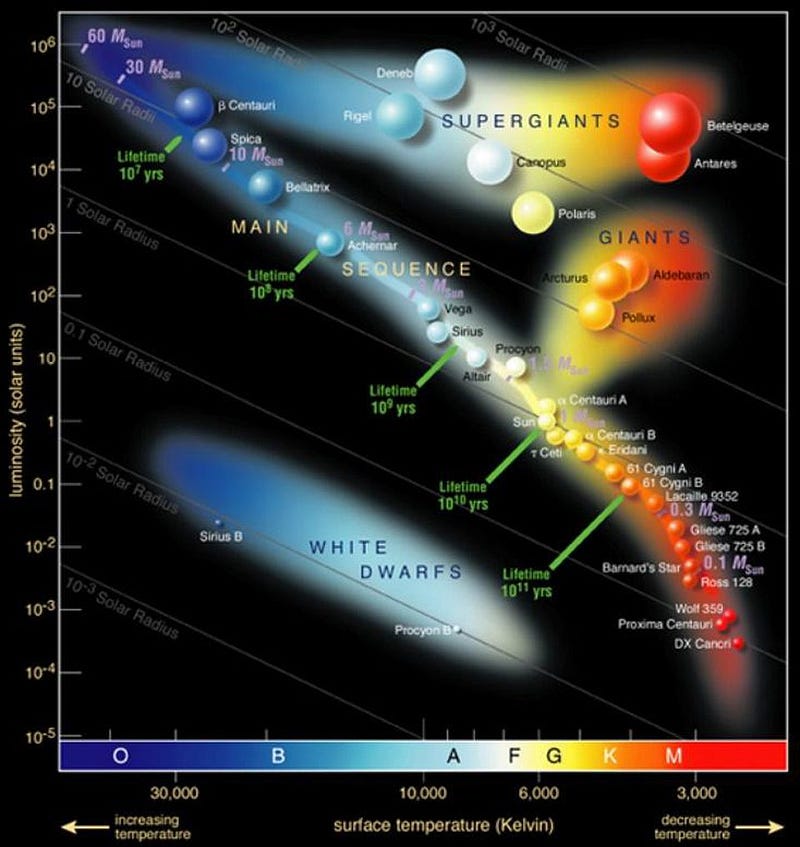
4:07 PM: See, there’s nothing to be afraid of, here. Emily is telling us how stars, in general work, and it’s nice and simple and straightforward. You burn through your fuel when you’re on the main sequence, or this big streaky diagonal line. As you burn enough fuel and run out of hydrogen in your core, you evolve off of this line, towards the right (and up), and that’s when you enter the red giant or supergiant phase… and that’s where the fun begins.
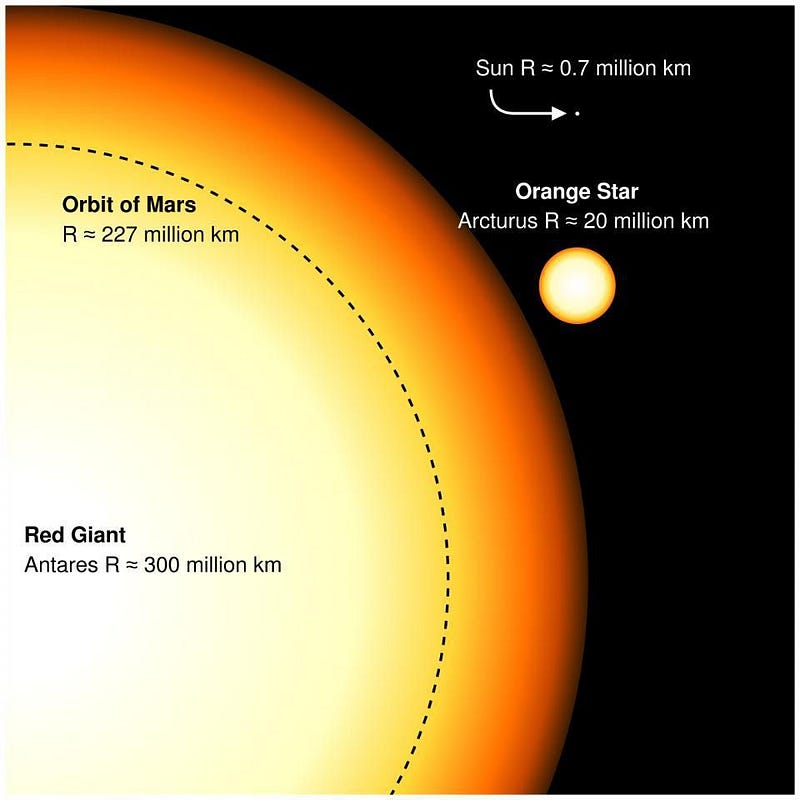
4:09 PM: It’s true: when you become a star like this, you become very different from how the Sun is now. But this doesn’t mean you’re “weird” in any real way… it means you’re obeying your normal phase of stellar evolution. And that’s only weird from the perspective of normalizing us. In reality, there’s a wide variety of what “normal” is. Perhaps we should learn that stellar lesson for ourselves, at the moments where we feel we’re not normal: there’s a wide variety of what normal looks like.
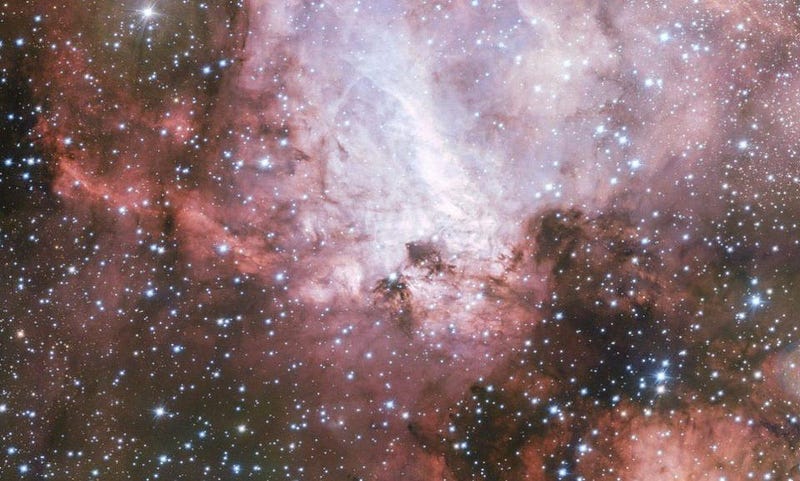
4:13 PM: What’s fun about stars and stellar evolution is that these very massive stars, the ones that become the red supergiants, are actually the shortest-lived of all stars. We find them even in star-forming regions, as they’ve burn through their hydrogen fuel in their core so fast, and when they expand, they cool, so drastically that they can actually form stable molecules (like titanium dioxide) in their outer atmospheres.
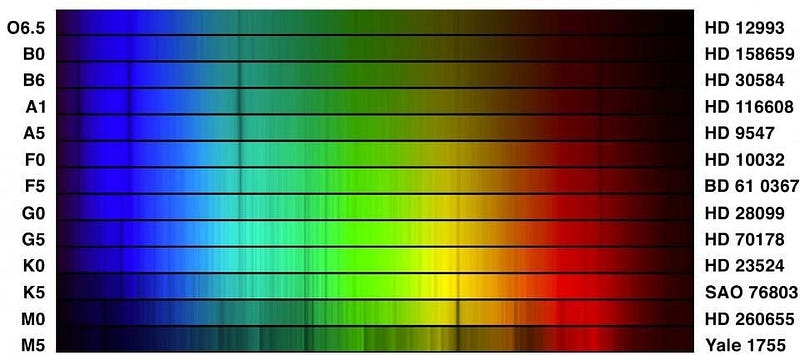
4:16 PM: What’s interesting is that these stellar atmospheres are so large and so cool, that the molecules forming at the edges can absorb blue light, preferentially, which shift the fitted temperatures of these stars to values that were too low: in theory, stars that were too cool to exist. It’s an interesting study in how we can fool ourselves if we don’t account for all the physical effects, including, oddly, molecules on the surfaces of stars!
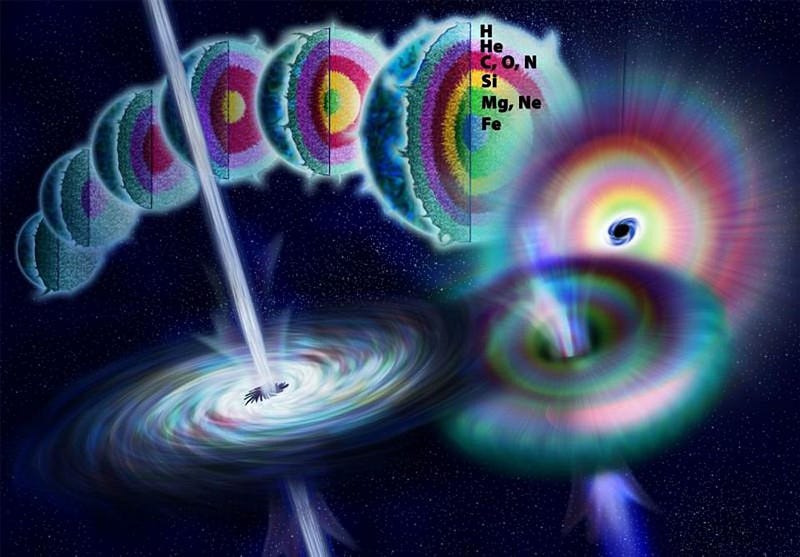
4:20 PM: Okay, so how do you go through stellar evolution, and go supernova? To hold up your star against gravitational collapse, you have to fuse elements: the outward push of radiation fights gravity. When you run out of hydrogen to fuse, radiation starts to lose, and gravitational collapse happens. That means, though, that you heat up as you get compressed, and if you have enough mass, you can heat up fast enough to start fusing helium.
This goes on: you fuse helium into carbon, carbon into oxygen… all the way up until you make iron, nickel, and cobalt. And then, my friend, you die.
4:23 PM: This is fast: while these different stages of burning last from days (like silicon) to thousands of years (for carbon/oxygen) to hundreds of thousands (for helium)… but supernovae occur in seconds.
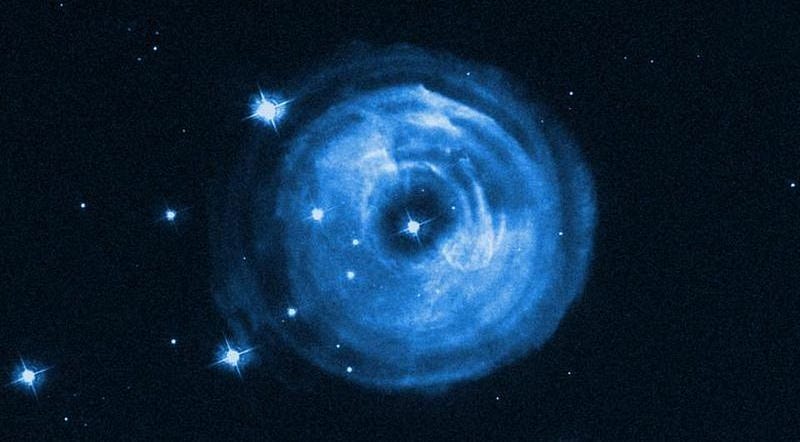
4:26 PM: But not everything is smooth like you think of. Emily’s now telling us about luminous blue variables, which throw off ejecta as they go through their late-stages in life. This is an interesting process that isn’t fully understood: why do some stars (usually the ones with more heavy elements) do this, while others don’t? This kind of open question is part of why astronomy and astrophysics, despite all we know, is nowhere close to coming to an end!
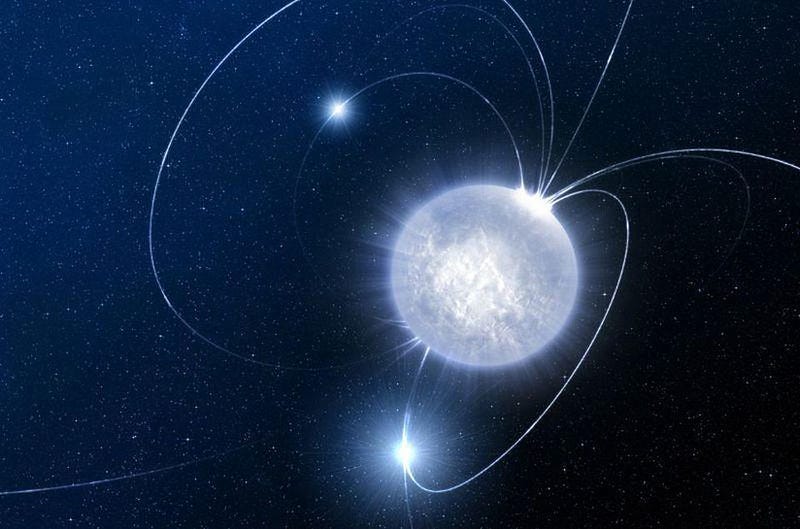
4:30 PM: The tough thing about a public talk like this is when you do a survey of objects or phenomena, you can’t go too far in-depth into anything. Emily talked about neutron stars and specifically the ones that are pulsars, but then went right on to black holes. Why? Because if you want to cover it all, you can’t spend too much time talking about any one thing in particular. There are, as a result, going to be lots of questions that flash through your mind, and then are lost as you go onto your next topic.
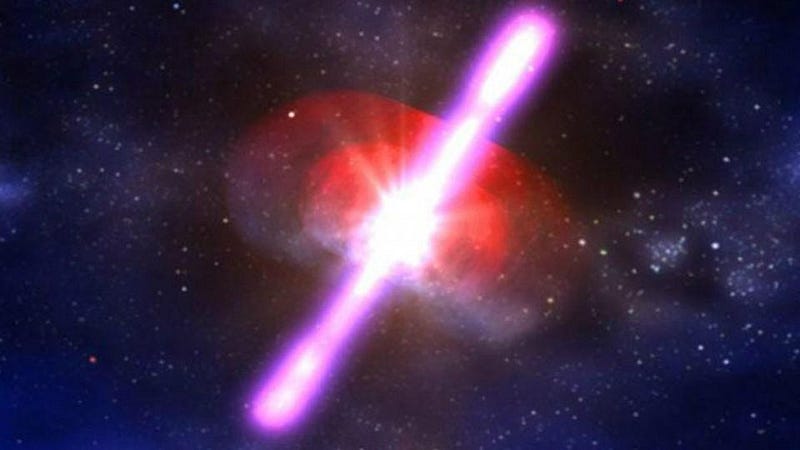
4:32 PM: But on the other hand, it’s also really cool, because you get to have a great survey of a whole slew of topics, like gamma-ray bursts… which we know now, thanks to LIGO/Virgo, are at least partially due to neutron star mergers!
4:35 PM: Here’s something that you don’t often get to appreciate in science: when you detect a rare or important event, here’s the process for how it works.
- You get a notification that something interesting and timely occurred.
- People get kicked off of their observation runs, and the big/important telescopes turn to point at what you’re seeking to detect.
- These follow-up observations, across a variety of wavelengths, give you a slew of data to look at.
- And it’s the data, not a pretty picture, that tells you the interesting physics/astrophysics/astronomy that’s going on.
And finally, you don’t announce it, you post your results in a publication and then the community synthesizes the suite of what all the astronomers have to determine exactly what went on.
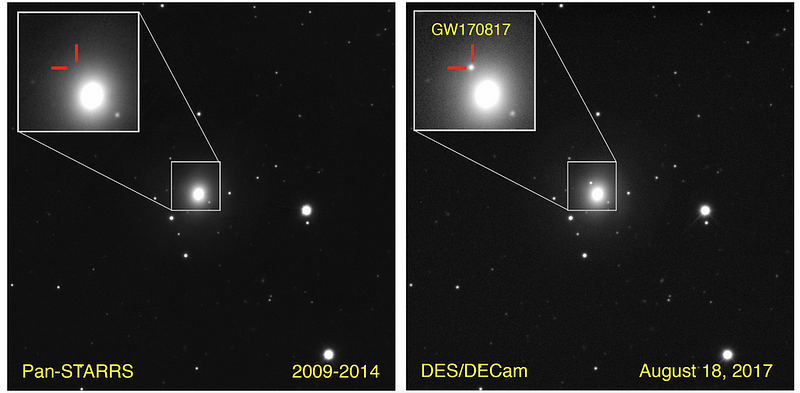
4:38 PM: This is really a vital part of the process: being careful and making sure you’re seeing what you think you’re seeing. Science isn’t always about being first or fastest or the one who puts all the pieces together; it’s about learning as much as possible and getting it right in the end. It’s how we combined gravitational wave astronomy, gamma-ray astronomy, and then multiwavelength follow-ups across over 70 observatories.
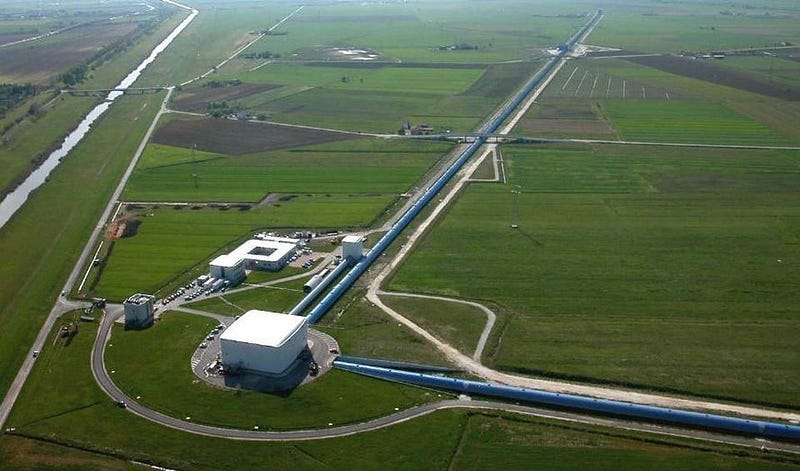
4:41 PM: I have to say, by the way, how exciting it is to see a pure astronomer like Emily, not an astrophysicist but an astronomer, talking about gravitational wave astronomy. That’s right, something that was once purely in the realm of physics, and then astrophysics, has made it to the point where astronomers talk about this as actual astronomy. This isn’t just physics anymore; astronomers no longer need telescopes to do astronomy!
4:43 PM: By the way, it’s important that Emily talks about these sensitive, transient events that are happening quickly, as time-domain astronomy. In other words, when time is of the essence, you absolutely have to look, because if you don’t jump at your chance to take that data, you’ll miss it!
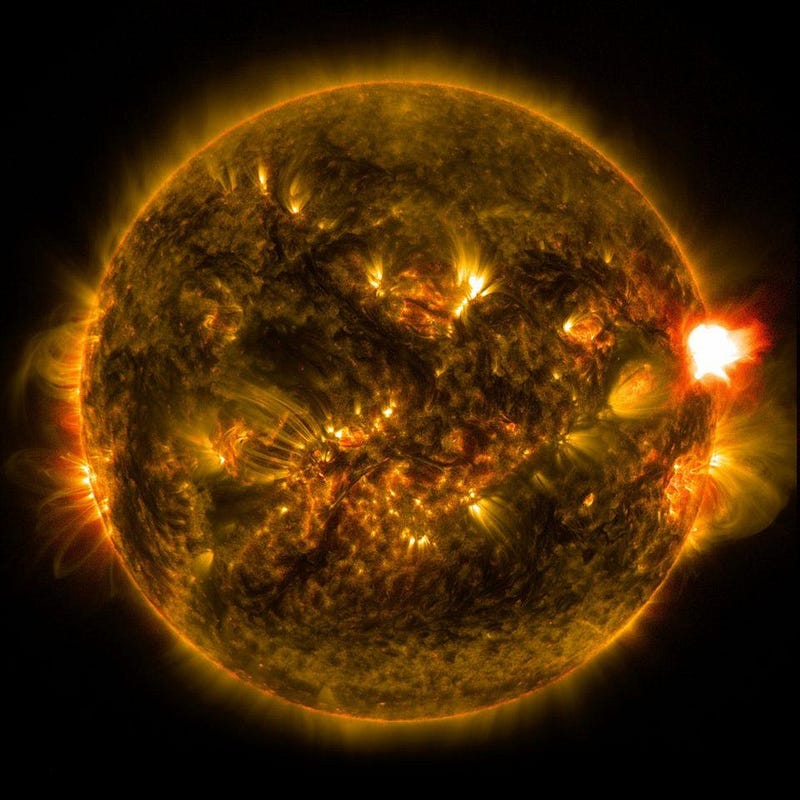
4:45 PM: Also, it’s important to recognize that sometimes there are false positives. For example, potassium-flare stars. Who sees stars flaring and emitting signatures of potassium? The answer is one telescope does, in France, and no others. It wasn’t due to potassium in the star, though, but potassium in the detector apparatus room, because people were striking matches.
4:48 PM: But… it turns out that there may be actual potassium-flare stars, since a non-smoker (haha) observed a similar signature. It is easy to fool yourself if a source you didn’t account for is causing an effect, but that doesn’t mean the effect you’re seeing isn’t actually real! For example, at the Parkes radio observatory, using the microwave at lunchtime, and opening the door, caused a brief flash of radio waves that made people think they were seeing a fast radio burst, but no, it was the microwave. Yet… fast radio bursts are real, and now we know more about them and have seen a bunch!
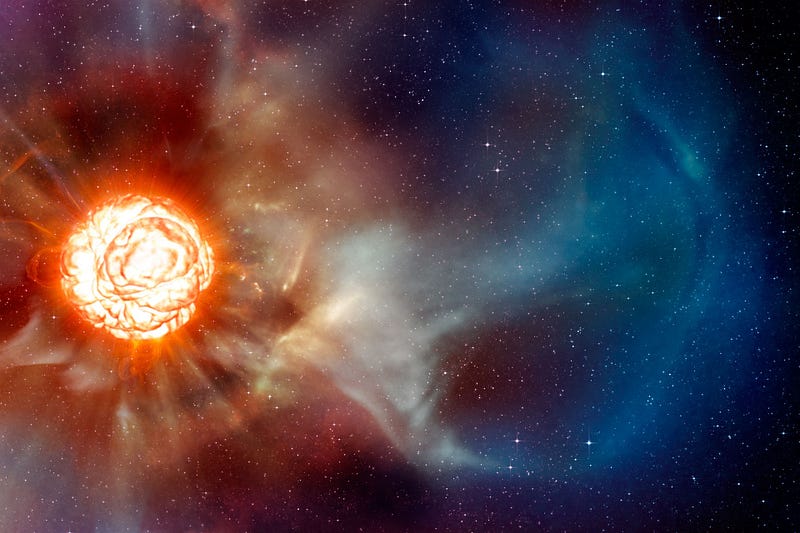
4:51 PM: Here’s a fun thing to imagine: what happens if you have a binary star system, where both are large and will go supernova? Well, one will go first, and perhaps it will produce a neutron star. Now, what happens if they spiral in, and merge? The neutron star will sink to the core, and so you get a red supergiant (eventually) with a neutron star at its core. This is what a Thorne-Zyktow object is, and it makes very explicit predictions for what you’ll observe at the surface!
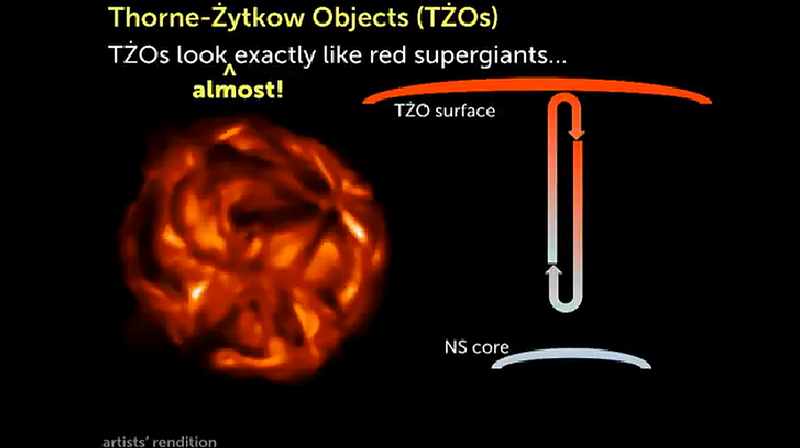
4:54 PM: How fun, that what’s going on is a combination of nuclear physics, thermal physics, and chemistry… and that when an atomic nucleus touches the surface of the neutron star, it only stays there for about 10 milliseconds, and will produce a chemical signature we don’t see anywhere else. And, lo and behold, you can find this odd, predictive chemical signature in a very small number of red supergiants, one-out-of-70, leading us to conclude that Thorne-Zyktow objects are real!
4:57 PM: I love the care that Emily is taking in calling this object a candidate, though. We have to make sure that there isn’t something else mimicking the effect we expect. Even when an observation fits your theory perfectly, you need confirmation from multiple objects and multiple lines of evidence. This is the way scientists work: we have to overwhelmingly convince ourselves, or it’s just likely rather than convincing.
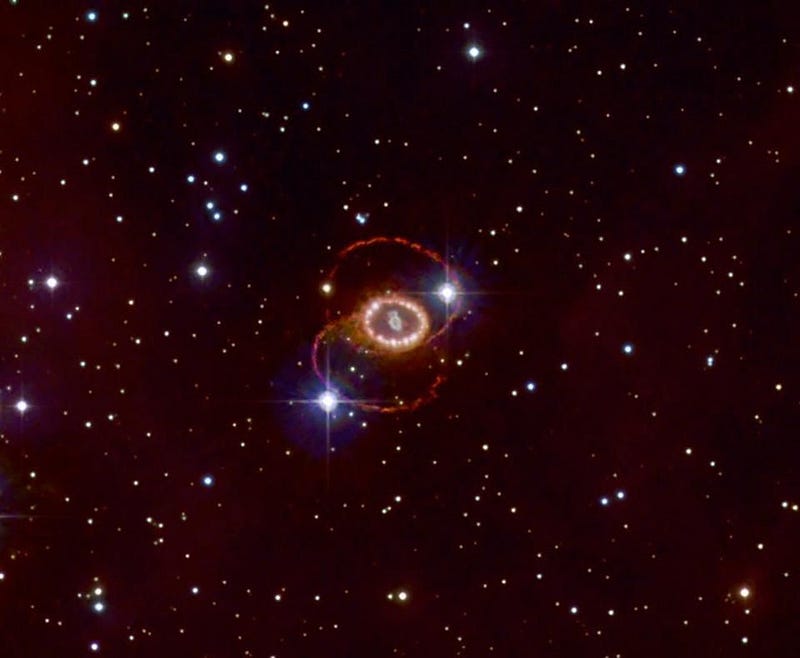
5:00 PM: There’s a great hope that stellar astronomers have: that someday within our lifetime, we’ll have a supernova we can observe with our own naked eyes. We haven’t seen one from Earth since 1604… but we could get one at any time. If you thought the eclipse was spectacular… just imagine what this would be like!
5:02 PM: Her talk is done, and it felt like it went fast and covered a lot of ground! I’m happy that she covered so many stars and star types, but I’m a little bit sad that things didn’t get weirder overall. Supernovae are great, but they’re not that weird. Thorne-Zyktow objects, though… I’ll give you that, those are weird!
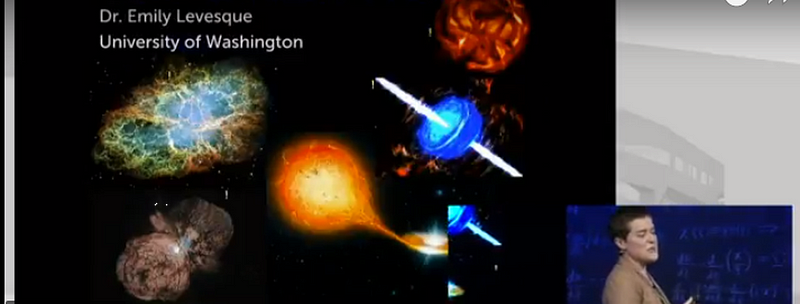
5:06 PM: So Emily showed these “weird objects” and said you’d be able to identify them all. Can you? It looks like we have, counterclockwise from the upper left:
- The crab nebula (supernova remnant), which is real,
- Eta carina, which is an ejection nebula around a luminous blue variable (real),
- A binary star pair, with one of them a neutron star accreting matter (illustration),
- A gamma-ray burst (illustration),
- and a Thorne-Zyktow object (simulation).
Not bad!
5:08 PM: And that’s it! I like Emily’s story of her excitement and passion, and when she knew she wanted to study the stars. Who knew from age 2? Well, Emily, born in 1984, knew: she saw Halley’s comet. She was fascinated with it… and she always wanted to be an X or an astronomer. A ballerina or an astronomer. A paleontologist or an astronomer. A marine biologist or an astronomer. And now, here she is! Science activities, stories (with representation, like a Wrinkle In Time), and encouragement helped.
Science is for everyone, and a tour of a public lecture like this is a great example of why we’re glad that it is! Thanks Emily, thanks Perimeter, and thanks to you for tuning in!
Ethan Siegel is the author of Beyond the Galaxy and Treknology. You can pre-order his third book, currently in development: the Encyclopaedia Cosmologica.