What color is the Sun? An astrophysicist answers
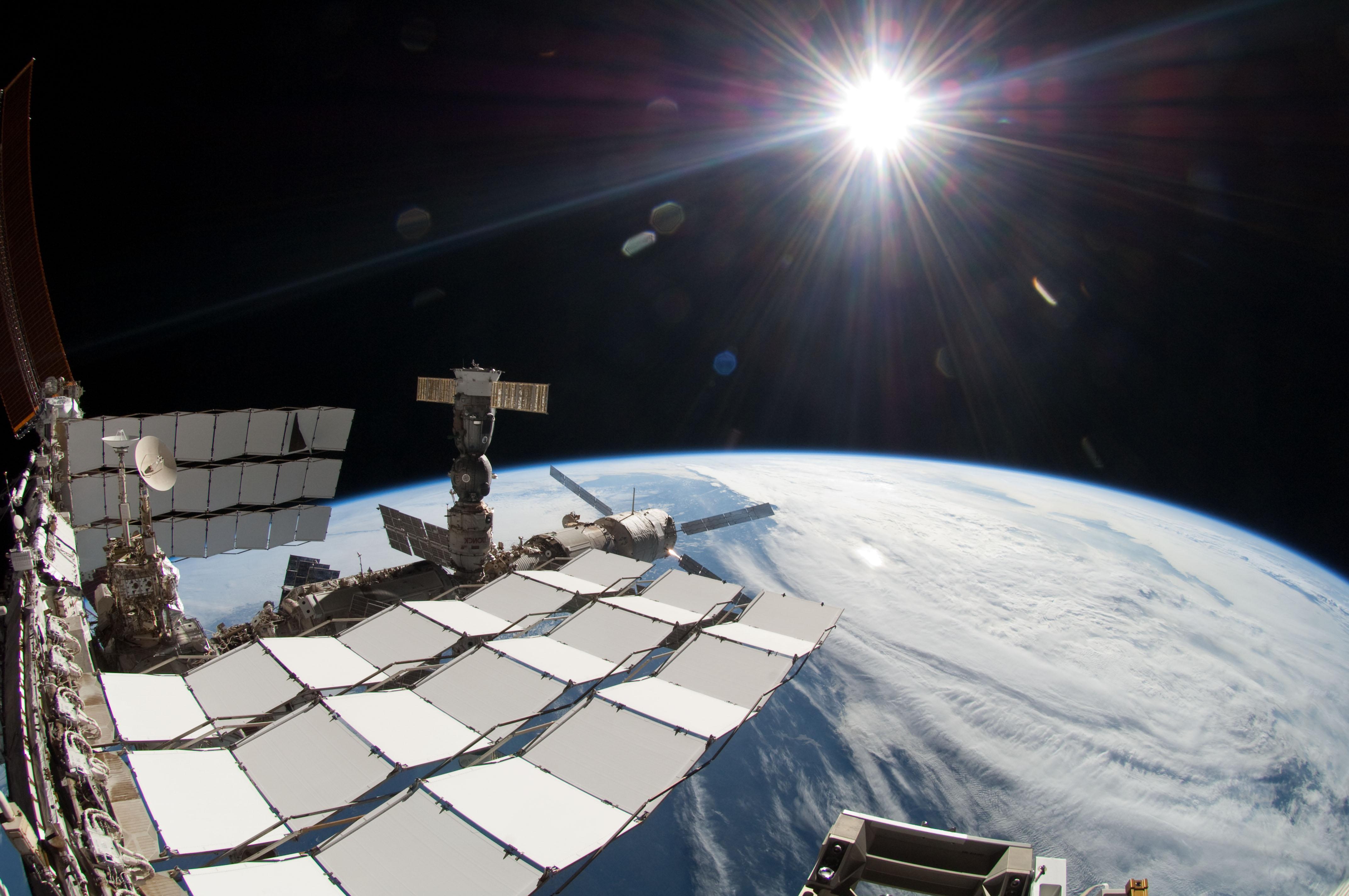
- The Sun, if you were to break up its light into all the different wavelengths that make it up, has its peak at green-to-yellow wavelengths.
- But there’s no such thing as a green-colored star, and the Sun is no exception: it looks white to our eyes, except when it yellows and reddens near the horizon.
- So what color is the Sun really? After you read this astrophysicist’s explanation, you won’t ever incorrectly say “green” again.
If there’s one instance where “seeing is believing,” it’s got to be wherever human eyes actually perceive the light that enters them. After all, it’s the very definition of what it means, in human terms, for us to see anything. And yet, somehow, people are falling for the very, very dubious claim that the Sun, “actually,” is a green-colored star.
If you are a person who:
- has opened their eyes in the past,
- has seen the Sun before,
- and has seen the color green before,
you know, from your own firsthand experience, that the Sun is not, in fact, green in color. So how is it that otherwise intelligent people are convincing themselves that the Sun really is blue-green in color?
Buried inside this absurd claim — and make no mistake, it is absurd — is a tiny kernel of truth: that the Sun contains a greater intensity of “green light” photons, or the quantum particles that compose light, than of any other wavelength or color. But simply having a wavelength peak in the spectrum of your light, or a maximum intensity at a given frequency, or a larger number of photons over a particular color range, isn’t enough to determine what color an object, even an object like the Sun, is in reality. The Sun, just as your eyes tell you, really is a white-light star, as the simplest experiment of all can reveal.
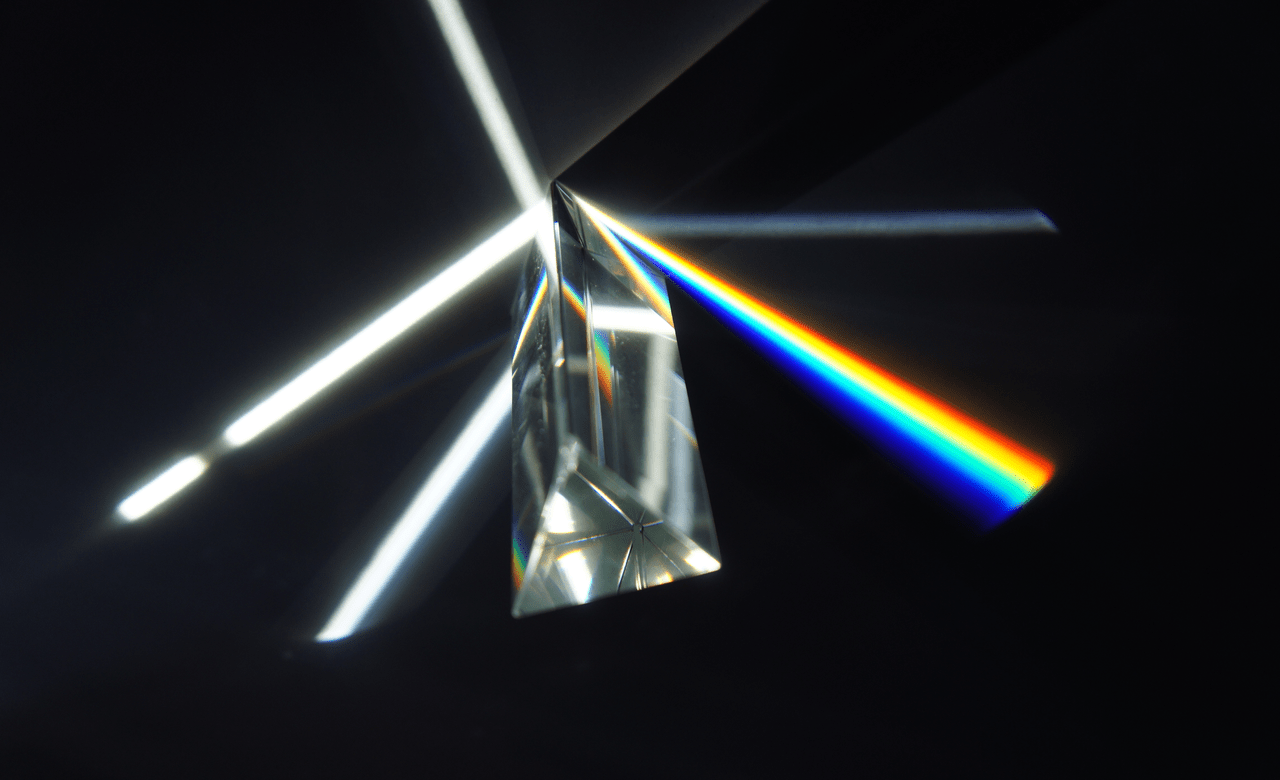
What experiment is that?
It’s very simple: take a substance that can reflect all the wavelengths of (visible, to human eyes) light that exist equally well, shine the light whose color you want to measure onto it, and then use your eyes to perceive what color you see when that light illuminates your reflective surface.
Where can you find this mystical substance that reflects all the wavelengths of visible light equally well?
It’s very simple: any solid, perfectly white object will do. A bright white sheet of paper, a white-painted section of wall, a whiteboard, or even a white flower, towel, or bedsheet will serve you just fine.
If you shine red light on it, it appears red, because it reflects the red light. If you shine green or yellow or pink or magenta or orange light, the outcome is exactly what you’d expect: it reflects the color of the light you shine on it, and hence it appears to take on that color for itself.
If you do an experiment, then, like taking a white piece of paper outside and holding it so that direct sunlight shines directly onto it, simply observing the apparent color of that paper will tell you what color the Sun is. Unless you’re looking at it during sunrise, sunset, during a total solar eclipse, or under highly polluted skies (such as during wildfire season), the color of that paper will be — at least to your eyes — unambiguously white.
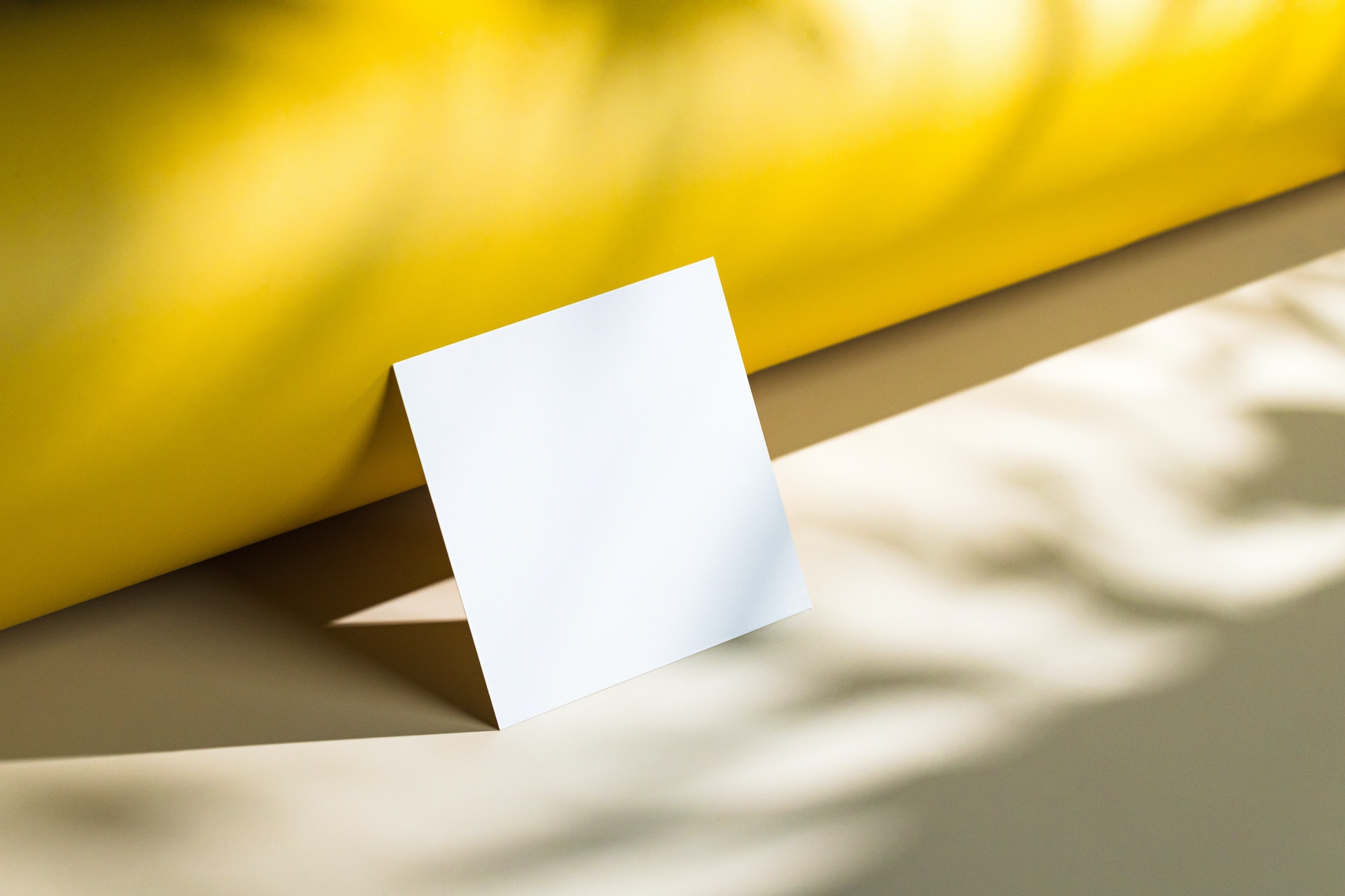
In fact, astronomers often say that there’s no such thing as a “green” star because of this very test. If you were to perform this type of experiment around any star within the known Universe, you’d find that there are only a finite set of colors that appear.
- For low-mass stars, like red dwarfs or the even cooler classes of stars (like the “failed star” class known as brown dwarfs), they’ll appear with a range of colors that depend on their temperature, with the lowest-temperature objects at between 800-1600 K appearing a faint, ruddy brown color that eventually transitions, at higher temperatures (1600-2700 K), to deep, prominent reds.
- As you move to higher stellar masses (or more evolved giant/supergiant stars), you can find stars more in the ballpark of ~2700-4000 K in temperature, appearing red-orange at the low end and orange-yellow at the high end, like Arcturus or Aldebaran.
- As the temperature of your star rises into the ~4000-5000 K range, the color becomes more yellow to yellow-white, such as the bright star Pollux. These lighting conditions are what we see on Earth at times corresponding to early mornings and late afternoons: where the atmosphere blocks a substantial amount of the shortest-wavelength light, leaving the longer-wavelengths behind.
- At temperatures ranging from about 5000 to 6000 K, which includes our Sun and stars similar to it, the color appearance is yellowish-white to white, which includes not just the Sun but many bright stars, including Capella.
- And then, the farther above 6000 K your star is, the color begins to gain first a cyan and then a brighter blue hue, such as the bright stars Castor, Rigel, and the brightest star of all as seen from Earth, Sirius.
The double star Albireo, shown below, provides a great example of two stars very close to one another with very different color-temperature properties, as its less-bright blue member has a temperature of around 13,000 K while its brighter, yellow member only has a temperature of around 4,400 K.
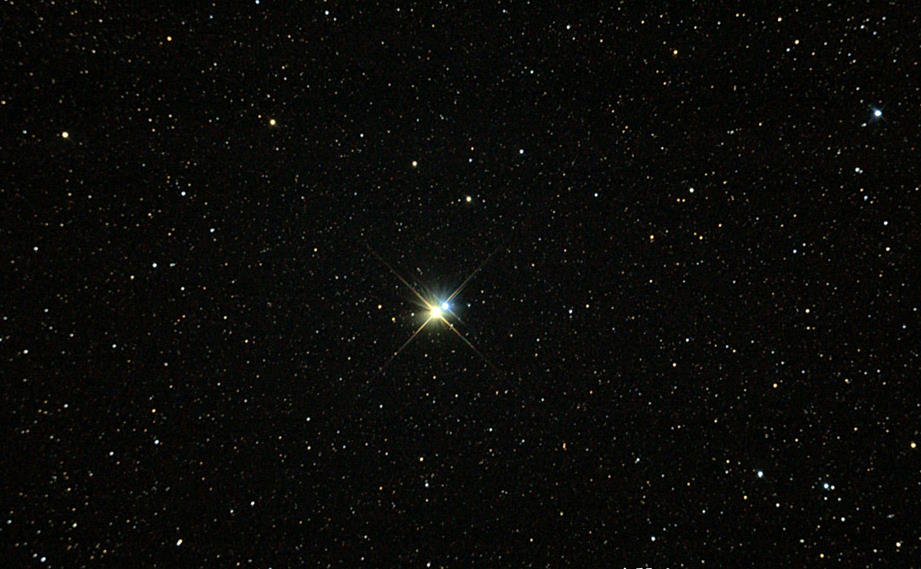
That’s it. When it comes to stars, those are the only options as far as color goes: you can go from brownish-red to red to orange to yellow to white to bluish-white to blue, and there are no other options. Those are the only colors stars come in, at all, with none of the more exotic colors you might have hoped for. There are no stars that come in any other color, including purple, green, pink, magenta, maroon, chartreuse, or aquamarine, among many others.
The reason so many people get this wrong — and why even, if you look hard enough, you can find NASA pages that get this wrong, too — is because they conflate two phenomena together: the color of an object and the wavelength of light that corresponds to some sort of “peak” in an object’s spectrum.
There is a physical circumstance where you can map “wavelength of light” onto “color” directly, but it’s a relatively rare circumstance: only when you have monochromatic light, or where all the photons (or particles-of-light) coming from your light source are of the same, precise wavelength. This circumstance frequently occurs when working with laser light or with some classes of LED light — which can consist of a single wavelength of red, yellow, green, blue, or violet, among other colors — but this is generally not applicable to the light that comes from stars.

Unlike lasers or other sources of monochromatic light, starlight from actual stars is composed of light that spans a huge range of wavelengths, dependent on the star’s temperature.
Any object that’s heated up to a certain temperature will emit radiation of a variety of wavelengths and frequencies, with the intensity peaking at:
- shorter wavelengths,
- higher energies,
- and higher frequencies,
as the temperature of the object rises. This is why a metal cauldron heated on a stove will begin to feel hot long before you can see it, as its intensity peak will fall in the infrared spectrum, which we feel as heat.
As you go to higher and higher temperatures, the object gets hotter, and the peak wavelength that it emits at shifts to shorter wavelengths: into the visible light spectrum. Interestingly, hotter objects continue to emit greater amounts of radiation than cooler ones at all wavelengths, even in the wavelength range where the cooler object has its intensity peak. The more heat an object contains, the greater the amount of energy it radiates away at all wavelengths, and the shorter-wavelength its intensity peak will be. In the most idealized gas, this object would also be a perfect absorber of all external radiation. If this is true, its radiation will follow an explicit spectrum: that of a blackbody radiator, which serves as an excellent approximation for the spectrum of most stars.
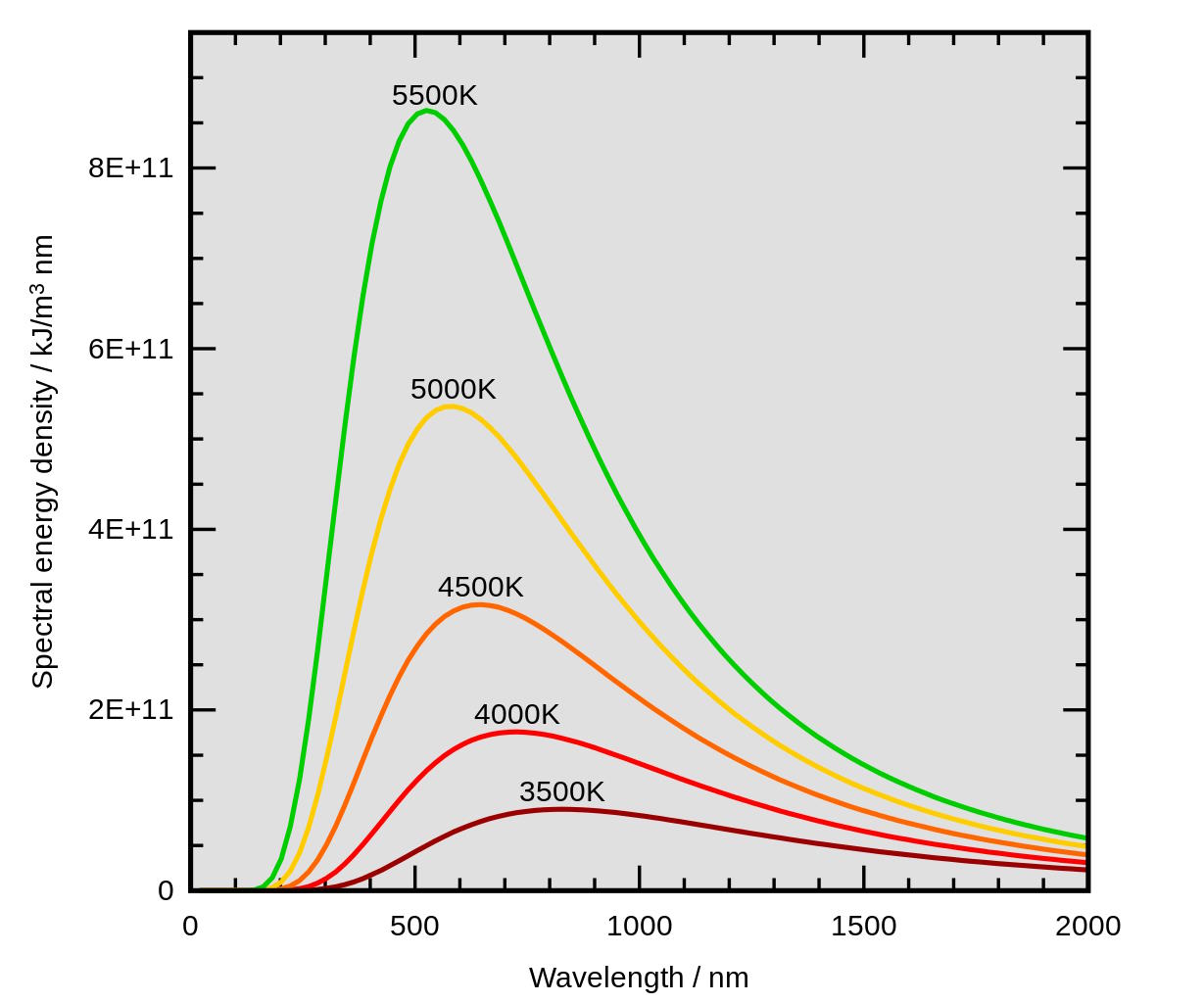
If you want to get even more detailed, it turns out that the Sun (or any star) isn’t a true blackbody, because it doesn’t have a solid, perfectly absorptive surface to radiate from. Instead, stars have photospheres, which are semi-transparent to light; they’re good absorbers, but they’re also low in density and possess a temperature gradient. The farther you are from the center of a star, the cooler you are, which has a big consequence for slowly rotating stars, like the Sun, but even bigger consequences for fast rotators, like the nearby bright star Vega.
Only a small fraction of the energy we receive from the Sun is emitted from the very edge of the photosphere; much of the light that we perceive originates several hundred or even a few thousand kilometers down into the Sun’s depths. Because it’s hotter in there, the Sun’s light doesn’t behave as a single “blackbody” at one temperature, but rather as a sum of blackbodies over a temperature range from about ~5700 K all the way up to nearly 7000 K farther into the Sun’s interior.
For rapidly rotating stars, like Vega, the temperature isn’t uniform across the star, but the star itself is compressed at the poles and bulges at the equator, just like Earth. As a result, the polar temperatures can be several thousands of degrees hotter than the farther-from-the-center equatorial regions.
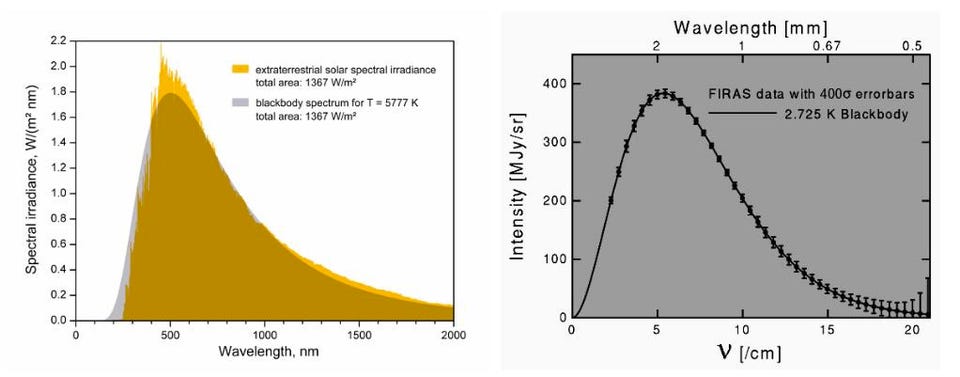
We’ve found stars in great varieties as far as their masses, temperatures, brightnesses, and many other properties are concerned. We’ve learned that a star can have its wavelength peak in intensity at any wavelength at all, including all over the visible light spectrum (from violet down to red) or even outside of it, such as in the ultraviolet or the infrared, including extremely far into these non-visible wavelengths of light.
But don’t be tempted to conflate “where the wavelength peak is” with color; because we’re not dealing with monochromatic light, this is simply an incorrect property to assign to light. In fact, “color” does not exist independent of our human perception, and for that, you need to understand what makes color for humans: the response of cone cells in our eyes and the interpretation of those responses by our brains.
Inside the typical human eye, there are three types of cone cells and one type of rod cell. The rods see only brightness (a monochrome property) and are our most prominent tools in low-light conditions and in our peripheral vision. The cones, on the other hand, are primarily located in our forward-facing field of vision and work best in bright light (e.g., daytime) conditions, and come in three varieties: S, M, and L, corresponding to short, medium, and long wavelengths.

The relative magnitude of the response in each of our three cone cell types enables our brain to interpret the color of objects, and even enables us to see composite colors: colors that aren’t part of the visible light spectrum, but that exist in nature as combinations of various wavelengths of light all summed together.
- Pink, for example, is white light with an additional red component to it.
- Magenta light, for another example, is a combination of blue/violet and red light together, which is why lights optimized for plant growth (i.e., absorption by both Chlorophyll A and B molecules) have that hue.
- And brown, for still another example, is a mix of greater amounts of red light with lesser amounts of green/yellow light, but with a dearth of blue light.
The Sun, being a mix of all different colors of light, is the truest example of “white light” that we know of, capable of having any wavelength of light (or combination of wavelengths) absorbed and/or reflected. However, just because it consists of green light as part of it doesn’t make it green; there are no stars that human eyes would perceive as green anywhere in the Universe.
However, some natural phenomena truly are green, like the Aurora Borealis, glowing green planetary nebulae, or the so-called green pea galaxies we see in space. The reason these appear green is because their light arises from a specific electron transition — within ions of doubly-ionized oxygen — which occurs at a monochromatic wavelength: 500.7 nanometers, a very green-colored wavelength.

Considering that the Sun does truly give off white light, it might seem strange to realize that it doesn’t always appear white. There’s a good reason for this: very few of us ever get the chance to observe the Sun from the vacuum of space. Rather, almost all of us are stuck down here, on the surface of the Earth, which means we only get to see the Sun’s light as it appears after getting filtered through Earth’s atmosphere.
Earth’s atmosphere consists of particles like molecules, and those molecules can scatter light. In particular, they scatter different wavelengths of light with different efficiencies: shorter-wavelength light, like blues and violets, get scattered more easily, while longer-wavelengths, like oranges and reds, get scattered less easily. The sky appears blue because the Sun’s blue light gets scattered in all different directions within the atmosphere, for example.
When the Sun is high overhead, it only passes through a little of Earth’s atmosphere, appearing white. As it lowers closer to the horizon, it appears with a cooler color-temperature, appearing red at sunset/sunrise, but progressing to orange, yellow, and eventually white as it rises higher, just as the Moon does. Under very favorable circumstances, right as the Sun or Moon is either rising or setting, you can see a slight “flash” of green or even blue light above it, as these shorter wavelengths can be “bent” just a little bit more as they pass through Earth’s atmosphere than the longer-wavelength yellows, oranges, and reds.
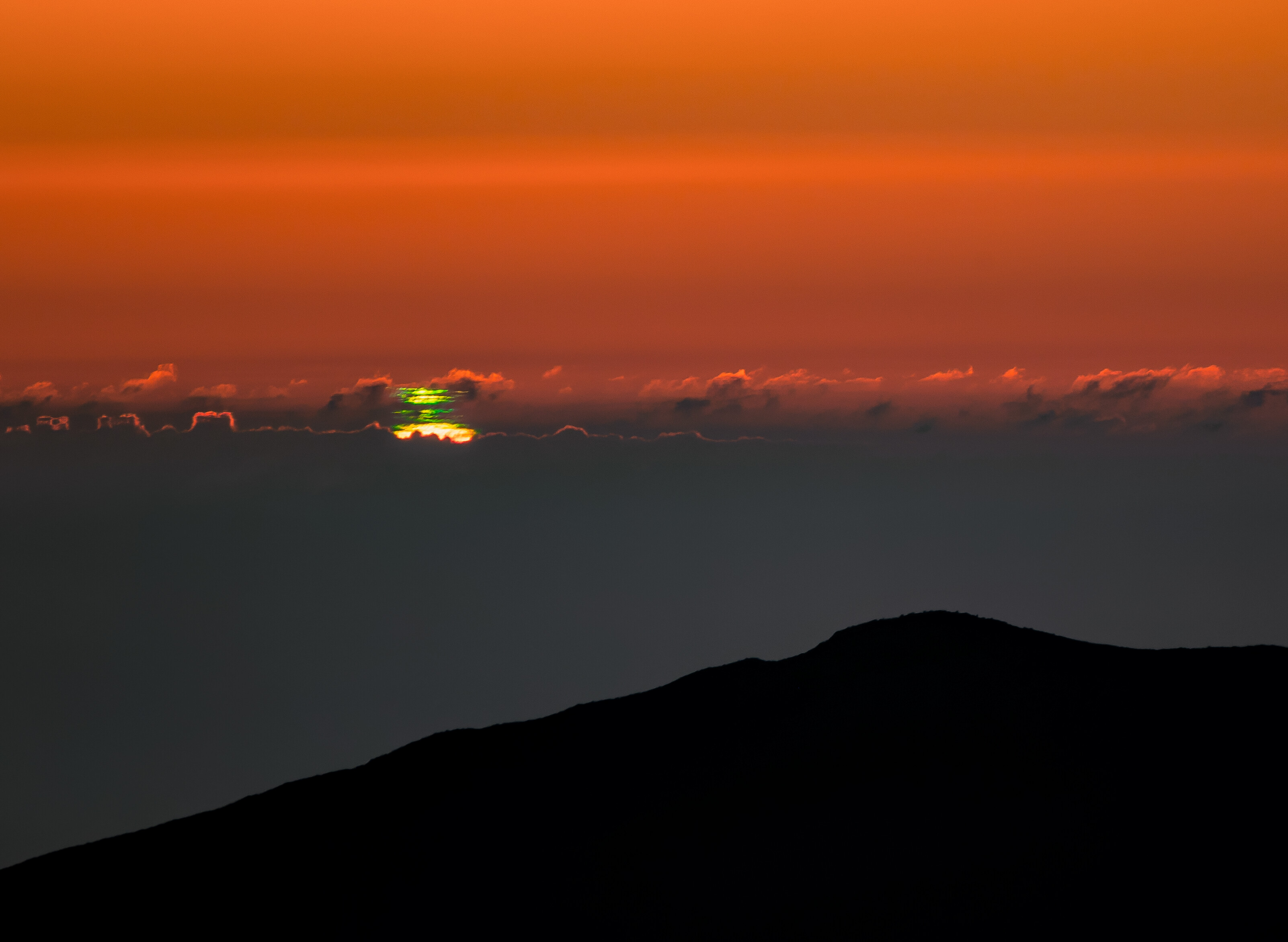
But simply being able to separate out, under just the right set of conditions, the green portion of our Sun’s emitted light doesn’t translate into our Sun actually being a green star. While there are still some that refer to our Sun as a “yellow dwarf” star, the truth is that our Sun is the whitest light we know of. In fact, it’s not a coincidence that we see sunlight as white, as our eyes and the cones within them have evolved from earlier forms of life that have always known a very similar Sun to the Sun we see today. Perhaps, had we come into existence around a hotter or cooler star, we’d have evolved with eyes, cones, and brains that interpreted whatever color light our star emitted as “white.”
But the reason people give to justify the statement that “stars are green” is fundamentally flawed, as “wavelength peak” has very, very little to do with what the intrinsic color of an object or an aggregate form of light actually is. The two ideas of “wavelength” and “color” can only be used interchangeably where purely monochromatic light is present. Whenever light is composed of many different wavelengths, that overly simplistic definition just doesn’t do the job; color, to our eyes, is a very human concept. This is one case where you really can believe your eyes: although sunlight contains green, it contains all of the other colors as well. When you add it all up — which our eyes and brains do automatically — it really is just white.