Ask Ethan: Will the Universe ever reach equilibrium?
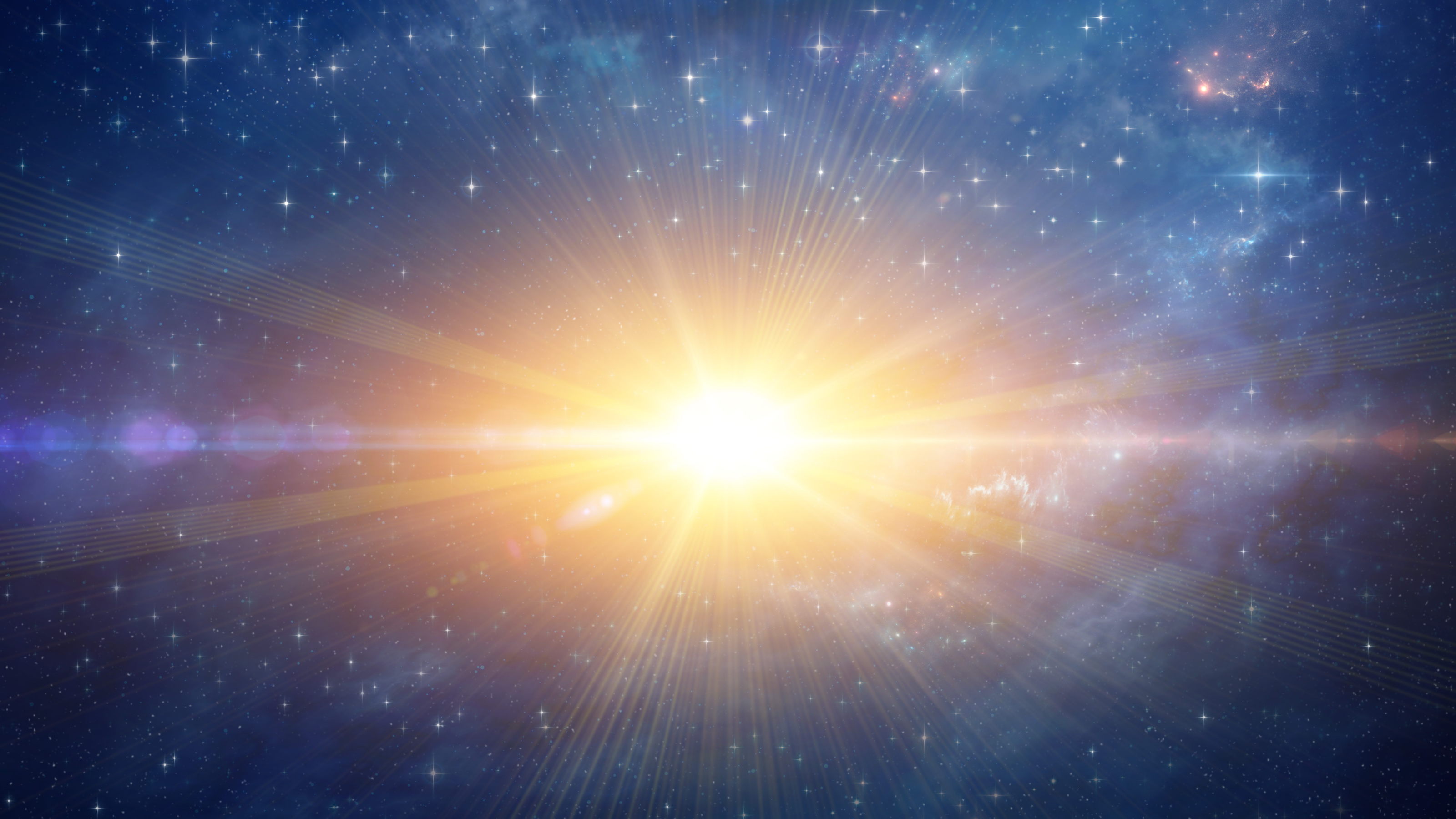
- After starting from a hot, dense, uniform, and rapidly expanding state, our Universe rapidly expanded, cooled, rarefied, and gravitationally clumped, giving rise to the Universe we now inhabit and recognize.
- This cosmic journey took us through many out-of-equilibrium transitions, and led to remarkable increases in entropy concurrently, bringing us ever closer to an equilibrium state where no further useful energy can be extracted.
- In the far future, will we ever reach that vaunted equilibrium state when it comes to the entire Universe, and if so, what will that look like? Here’s the best story that science can tell, at present.
In our experience, all physical systems eventually tend toward equilibrium: where entropy is maximized and no further energy can be extracted from it. This seems like an inevitable consequence of the second law of thermodynamics, and is absolute for any closed-and-isolated system. But our Universe is neither closed nor isolated, as it began from a hot and dense state and has been cooling, expanding, and clumping ever since the hot Big Bang. Even though its entropy has increased dramatically, parts of it like stars, planets, and even biological organisms, routinely extract energy and put it to work toward creating ordered systems. It seems like equilibrium, even 13.8 billion years later, is still very far away in a cosmic sense.
But will the Universe — the ultimate out-of-equilibrium system, in some sense — eventually reach equilibrium after all? That’s what James Calautti wants to know, asking:
“Is it possible that in the far distant future, after every single star has died, after the white dwarfs and neutron stars have faded, and the black holes have decayed, will the universe achieve a state of equilibrium?”
If certain assumptions hold true about our Universe, then yes, we will eventually achieve a state of pure equilibrium: where no further energy can be extracted to do work or enable reactions of any type. But that’s not necessarily how it’s going to shake out, even in the end. Here’s what we need to consider.
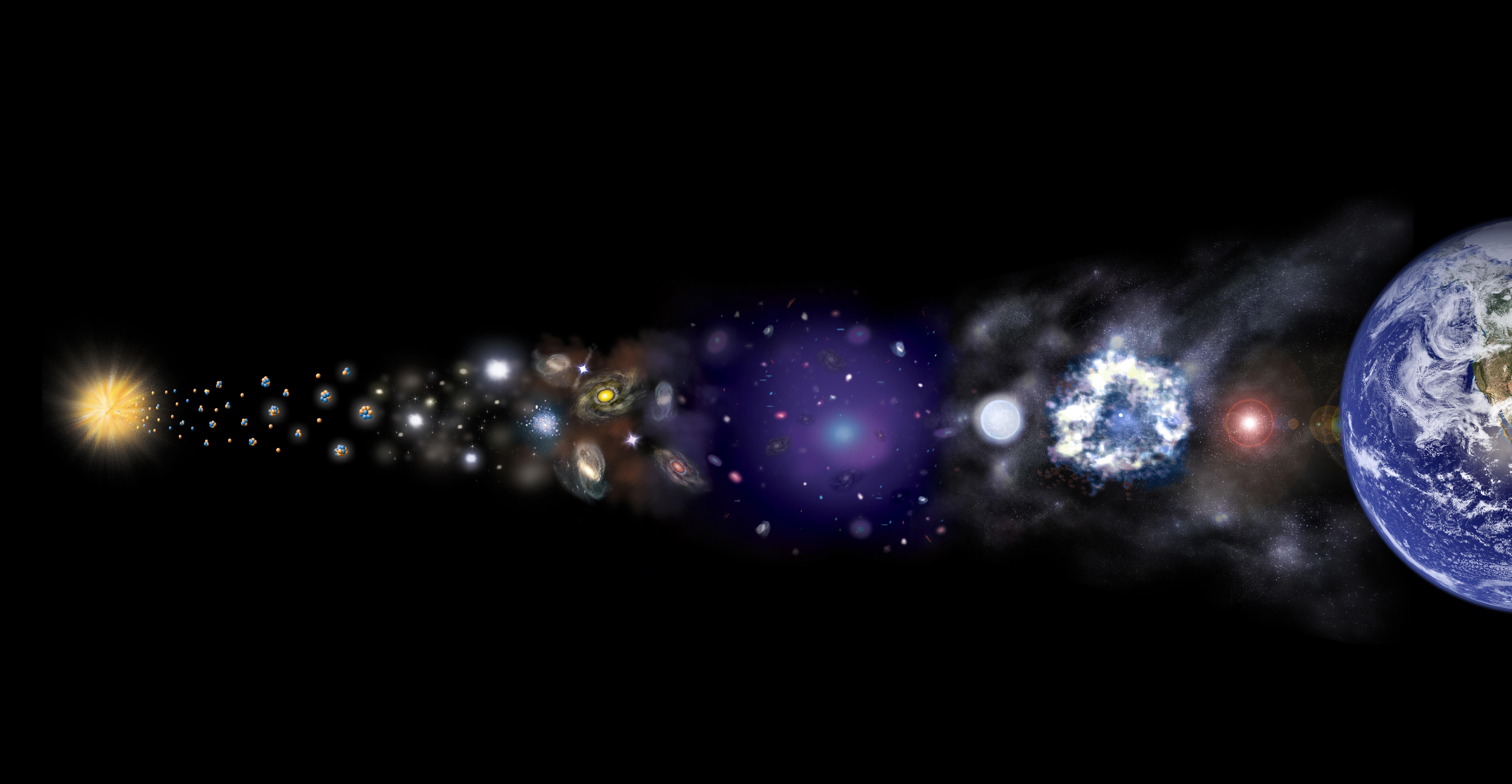
When the Big Bang first began, the Universe had practically no structure in it at all. No stars, no galaxies, no atoms, no atomic nuclei. It was hot, dense, and incredibly uniform: where the least dense regions were still ~99.99% as dense as the average ones and the most dense regions were only ~100.01% as dense as the average. Even though it was filled with ultra-relativistic quanta of radiation, plus particles of matter and antimatter, its entropy was around S = 1088 kB, where kB is Boltzmann’s constant. While 1088 may be a very large number, it’s not maximally large, especially not for the number of particles in the Universe.
Over time, as the Universe has cooled and gravitated, all sorts of structures have formed, from atomic nuclei to atoms to molecules, all the way up to planets, stars, stellar systems, galaxies, and clusters of galaxies embedded within a cosmic web. It’s as though the initially high energy state of the Universe, as the Universe expanded and cooled:
- proceeded through a number of transitions,
- where, from the hotter-and-denser conditions to the colder-and-sparser conditions, these transitions proceeded in an out-of-equilibrium fashion,
- leading to the binding and formation of structure,
- that seemingly created tiny “ordered” pockets at the expense of a larger-scale increase in “disorder,”
so that entropy increased tremendously over time. Today, the entropy of the Universe is about S = 10103 kB, or about 15 orders of magnitude (a factor of a quadrillion) greater than it was 13.8 billion years ago.
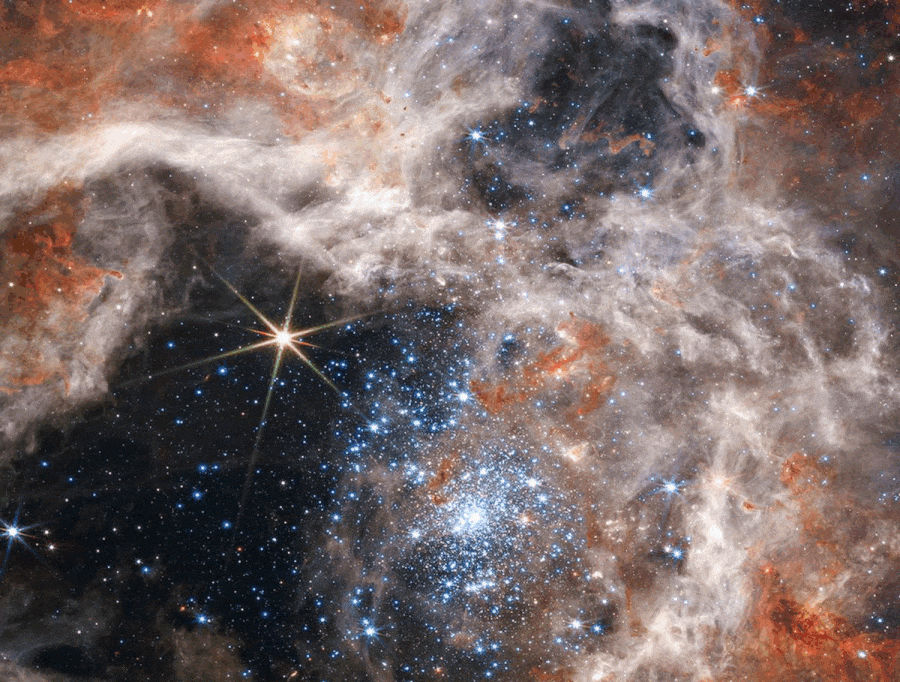
As time continues to march on, all sorts of energy-emitting reactions will still occur. Neutral atoms will form from the ionized plasmas in interstellar space. Light atomic nuclei will fuse into heavy ones inside the cores of stars. Clouds of gas will gravitationally collapse into bound structures like stars and planets. And massive objects will collapse down to create black holes, among many other natural processes. All of these processes, as well as many others, emit energy, which allows work — the physicist term for energy-that-gets-put-to-use — to be performed. These processes all increase entropy on a global scale, but the emitted energy can be used to create regions that are more ordered, the same way that sunlight absorbed by photosynthetic organisms on Earth can be used to locally create order.
Nevertheless, the more energy-emitting reactions occur and the more time that passes, the greater the Universe’s entropy gets. As this occurs, there are now fewer opportunities for extracting energy from various processes. The Universe runs out of hydrogen, and fewer and fewer new stars form. Dark energy drives galaxy groups and clusters apart, and fewer cosmic mergers occur. More black holes form, and more compact masses get ejected into intergalactic space. Eventually, the entropy of the Universe starts to level off at a maximum value of around S = 10121 kB, which it will reach around ~1020 years from now.
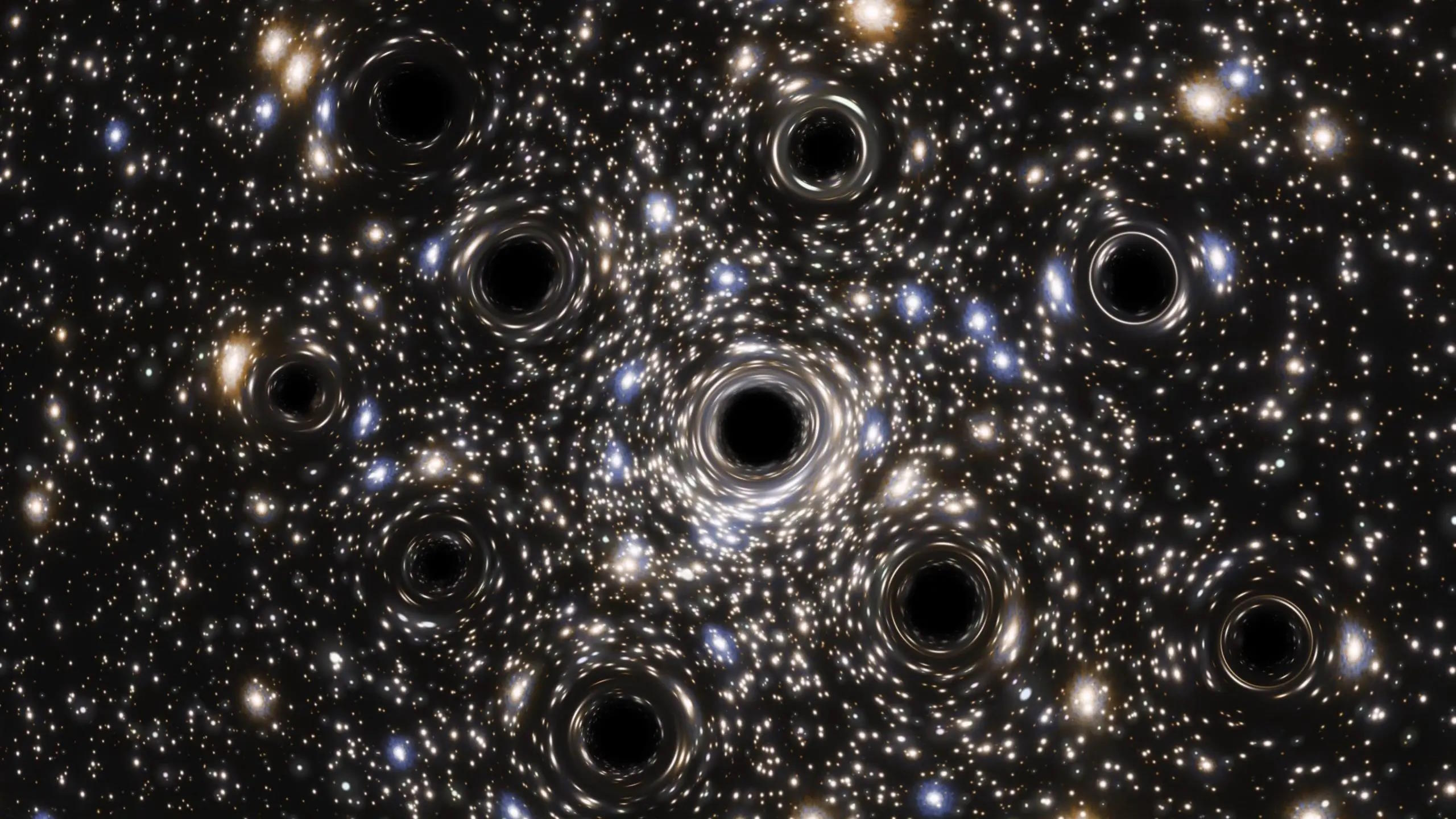
At that point in the far future, all of the stars that exist today will have long since burned out. The future generations of stars that will have formed from their ashes and the remaining gas within galaxies will have burned out, too, leaving only stellar remnants behind: white dwarfs (which will have faded to black), neutron stars (which will have faded to black as well), black holes, and failed stars. On occasion, two failed stars will merge together and briefly create a low-mass red dwarf star: the last luminous lights present in our cosmos. When they burn out and fade to black as well, the last stellar lights will be extinguished.
Gravitational interactions will cause galactic remnants to decay and dissociate. Objects in orbital systems, like planets around stellar corpses, will see their orbits decay due to gravitational radiation, leading to inspirals and mergers. Black holes themselves will decay away through Hawking radiation, with stellar mass black holes taking ~1067 years to decay and the largest supermassive black holes taking upward of ~10100 years to decay away completely. Meanwhile, intergalactic space becomes sparser and sparser as dark energy continues to accelerate unbound objects away from one another. Eventually, there are no energy-producing sources left in the Universe, and the entropy of what remains is maximized.

When the final black hole in the Universe decays, releasing energy via Hawking radiation, the last source of electromagnetic energy will have been created. When the final gravitationally bound objects inspiral into one another and merge, the last source of gravitational wave energy will have been created. At last, there will be no more energy to extract from any natural, physical process anywhere in the Universe. That end state marks what we call the thermodynamic “heat death” of a system: in this case, the system is the entire Universe. That state, where:
- no further energy can be extracted,
- no more useful work can be done,
- and where entropy has reached its absolute maximum and can increase no further,
is what truly represents an equilibrium state. So long as there are no further transitions that are going to occur — assuming dark energy is truly a cosmological constant and that there are no undiscovered fundamental forces, interactions, or reactions that can occur — that’s the end state we’re headed for. There will be isolated, stable clumps of dead matter that are present, all within an expanding, dark energy-dominated Universe, with a tiny, low-energy background of uniform radiation arising from dark energy’s presence (via Unruh radiation) with a minuscule temperature of around ~10-30 K.
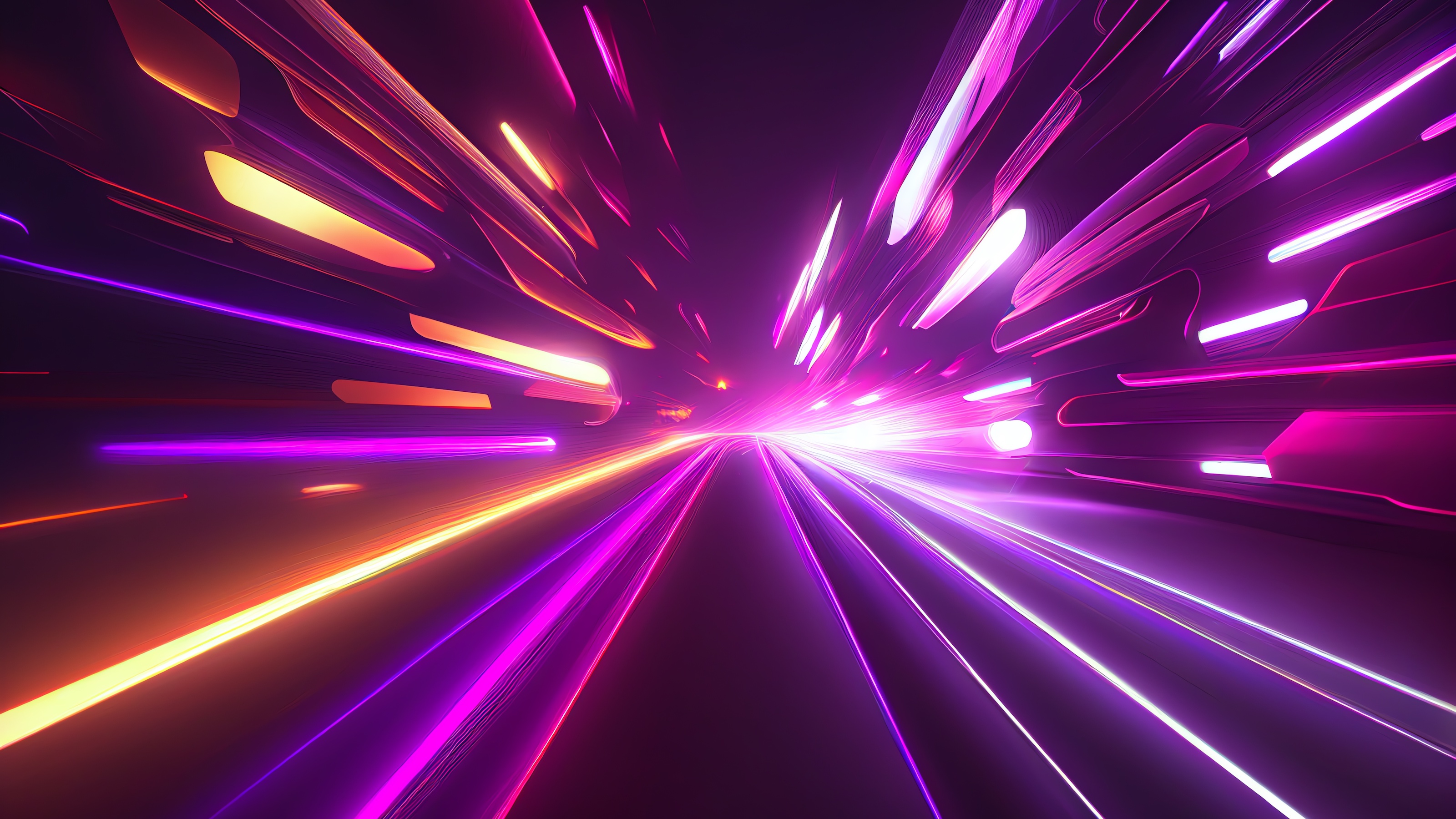
At least, that’s what we can expect in a completely “vanilla” Universe. Our Universe, however, may not be completely vanilla at all, as by vanilla, we’re assuming a number of things:
- that dark matter is stable, collisionless, and non-self-interacting,
- that dark energy is a cosmological constant and will never change in its properties,
- that protons are fundamentally stable and there are no super-heavy exotic bosons that will lead to its decay,
- and that there are no new matter-or-energy transitions that are allowed to occur, such as vacuum decay.
None of these things are necessarily true, of course. They’re largely consistent with our best observations to date (although there are some hints that suggest otherwise), but we are compelled to keep an open mind as to what surprises still remain possible.
It’s possible that there will be additional sources of energy that we have not yet discovered that will reveal themselves down the line, delaying equilibrium. It’s possible that further decays are going to occur, either of normal matter, of dark matter, or even of dark energy and/or the quantum vacuum itself. And it’s also possible that dark energy will evolve in a way that will cause it to either strengthen over time, leading to a Big Rip scenario and/or a new inflationary epoch, or that will cause it to reverse sign and recollapse the Universe in a Big Crunch. We have to keep our minds open to these possibilities, even if there isn’t yet data to support their reality.
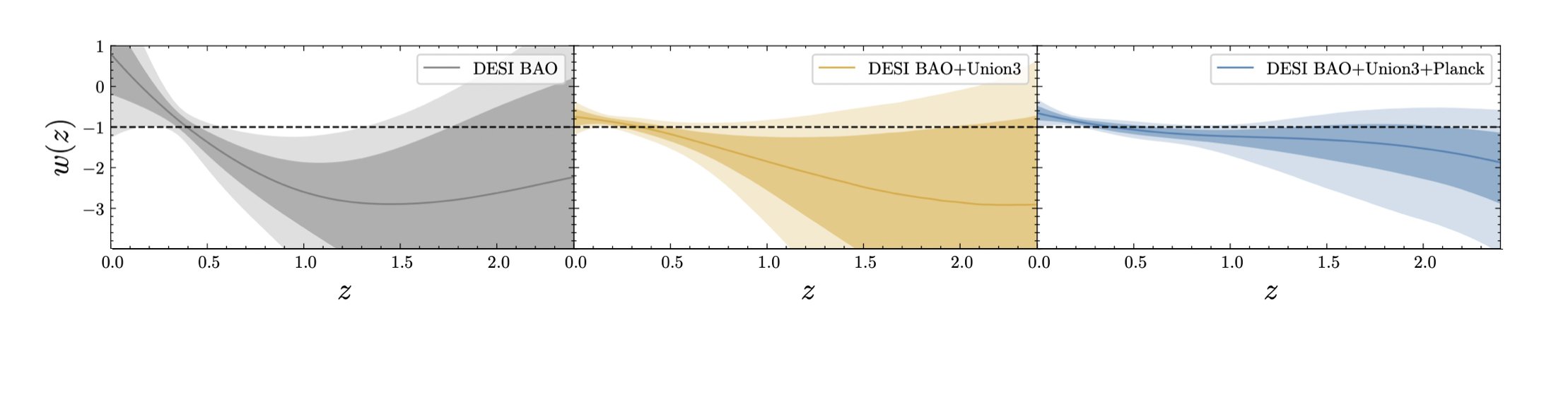
Does dark energy evolve over time? Is it something other than a pure cosmological constant? If we look at the most recent data from DESI collaboration — the Dark Energy Spectroscopic Instrument collaboration — which has taken data from millions of galaxies across billions of light-years of space, it suggests (but does not prove) that dark energy used to be stronger, and “more negative” in the past, and is now weaker, and “less negative” than it used to be. A recent paper from collaboration members (as pointed out by Ciaran O’Hare) shows that, from DESI data alone, this evolution is strongly indicated, but that when you include supernova data and CMB data, the evidence for dark energy’s evolution weakens substantially.
If dark energy either:
- does evolve,
- can evolve,
- or can transition to having a different value than it has today,
then it’s no longer necessarily the case that we’ll approach an equilibrium state as outlined in the “vanilla” Universe case. It’s possible that there will be more energy to extract, but it’s also possible that our modern picture for the far future of the Universe is incomplete and missing an important transition or event that will someday occur. If dark energy is non-constant or if it is possible to extract energy from the vacuum of space after all, then all bets are off.
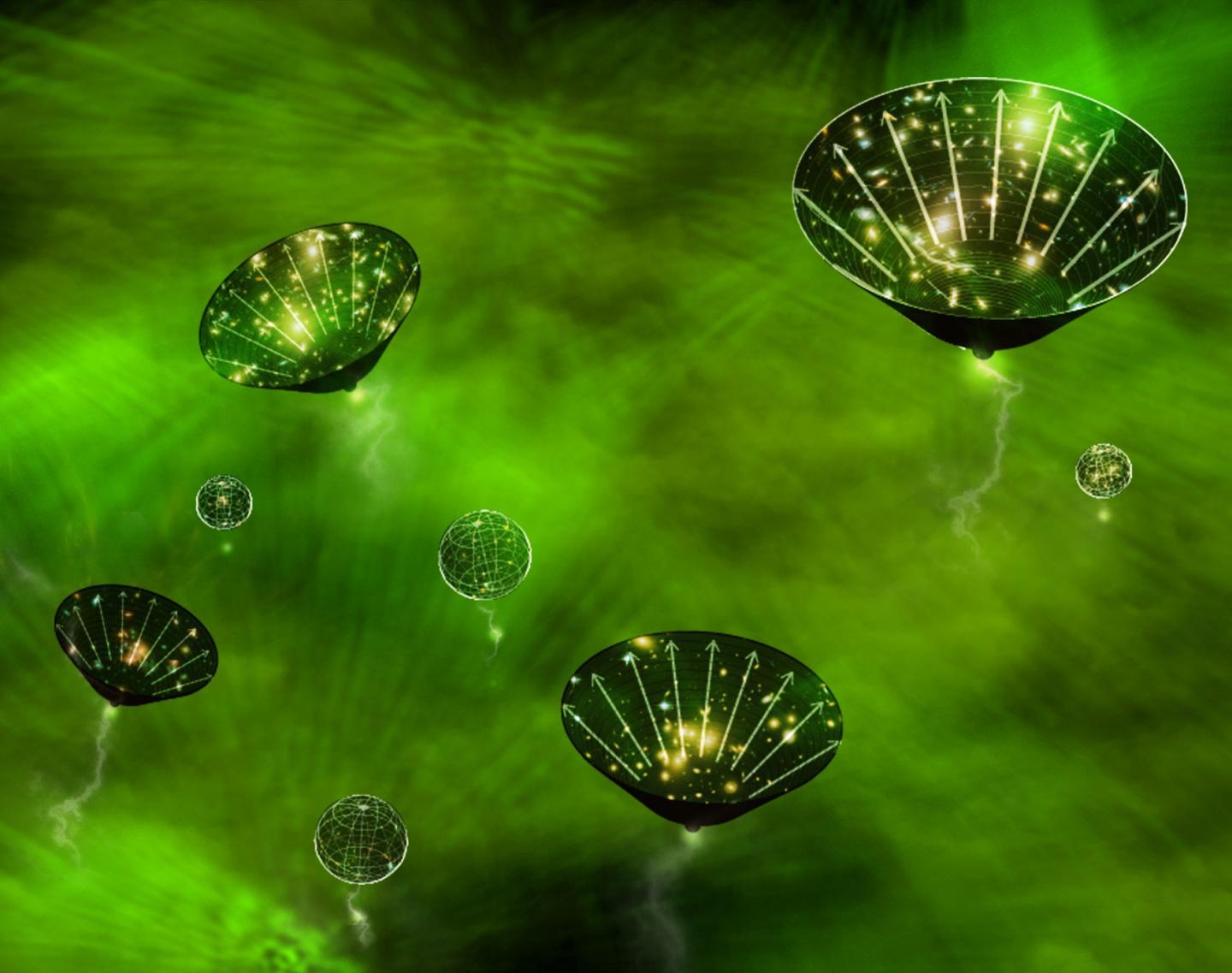
It’s also possible that our current understanding of black holes is incomplete. It’s possible that what we perceive as black holes are merely gateways to a baby universe, but that the only way to know is to be “inside” that black hole, in which case we’ll never be able to know that from our perspective outside the black hole’s event horizon. It’s possible that every time a black hole is formed, a new baby universe is spawned, with someone inside that Universe perceiving their own inflationary event followed by a hot Big Bang. If this is actually true, then what we perceive as “an equilibrium state” in our own Universe is actually accompanied by an enormous suite of cosmic evolution in each of the baby universes that arise as “daughters” of our own.
There are many ways to envision our own Universe failing to come to equilibrium as well. Our far future, if dark energy is a constant, looks a lot like our earliest beginnings: dominated by empty space that’s expanding exponentially. This correspondence between the late-time dark energy state and the early-time inflationary state has only the magnitude and scale of inflation vs. dark energy as the major difference. It’s possible that some mechanism that hasn’t yet been discovered can result in some type of transition in the far future: one that leads to a new type of Big Bang (or its analogue) and then gives rise to all sorts of other non-equilibrium reactions.
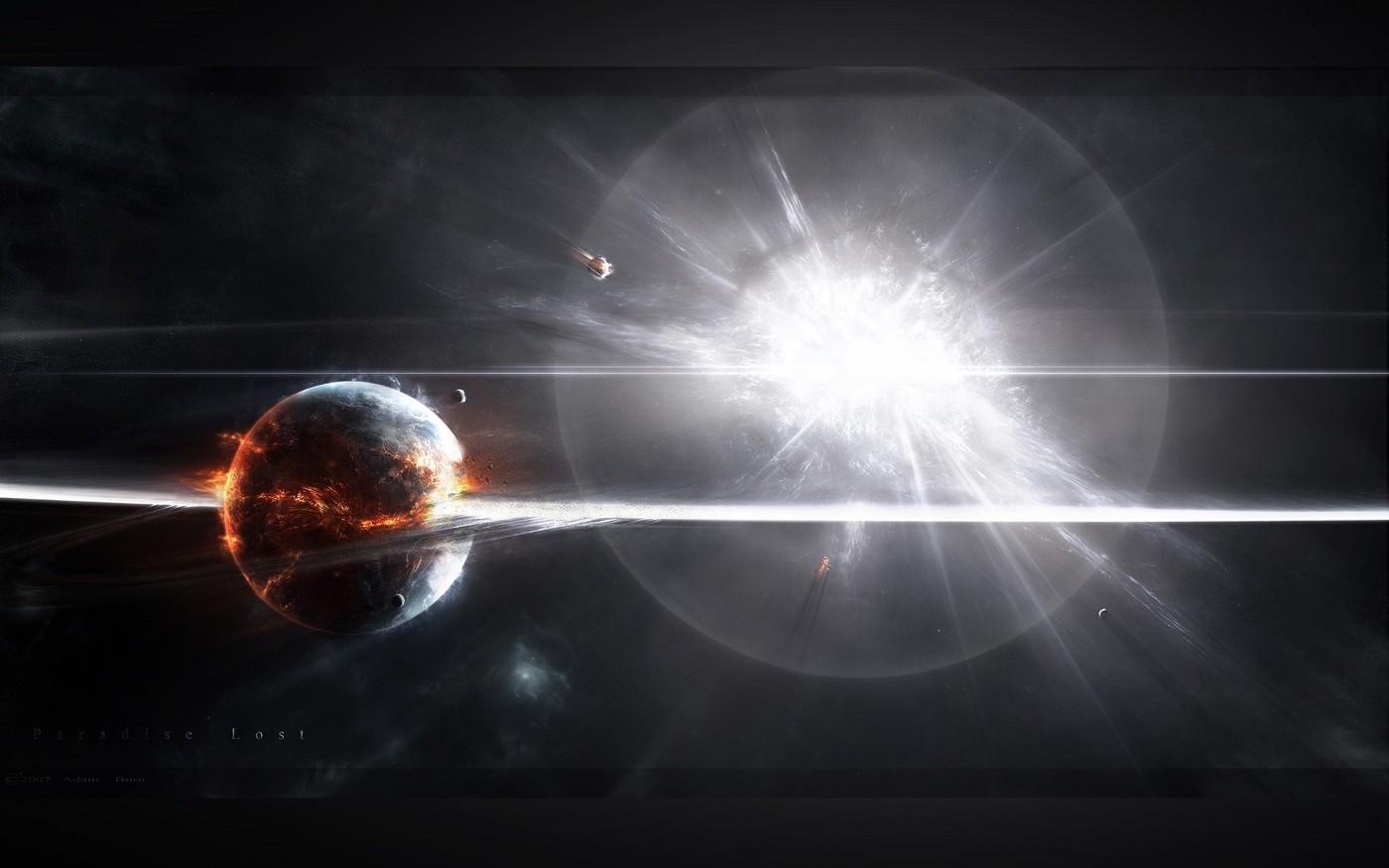
We can only draw conclusions about what we expect to occur, of course, based on what we know and observe today. We assume that our best observations, measurements, and theories about the Universe represent an accurate picture of reality, and all of our conclusions are reliant on how good those assumptions are. If general relativity and quantum field theory paint a complete picture of the forces in our Universe, and if dark matter (as a collisionless, non-self-interacting species of particle or fluid) and dark energy (as a cosmological constant) are the only exotic, non-Standard Model ingredients in our Universe, then yes: we can expect that our Universe will someday, in the far future, reach equilibrium.
In this scenario — where everything agrees with the Standard Models of cosmology and particle physics — then there will be no novel transitions or releases of energy in the far future beyond what’s already known: stellar death, gravitational wave emission, black hole decay via Hawking radiation, etc. We won’t live to see it, but the Universe will approach an equilibrium state, resulting in what we call the heat death of the Universe. It will become cold, isolated, empty, and filled with an extremely low-energy bath of radiation, from which no further energy can be extracted. As entropy is maximized, an equilibrium state will eventually be achieved.
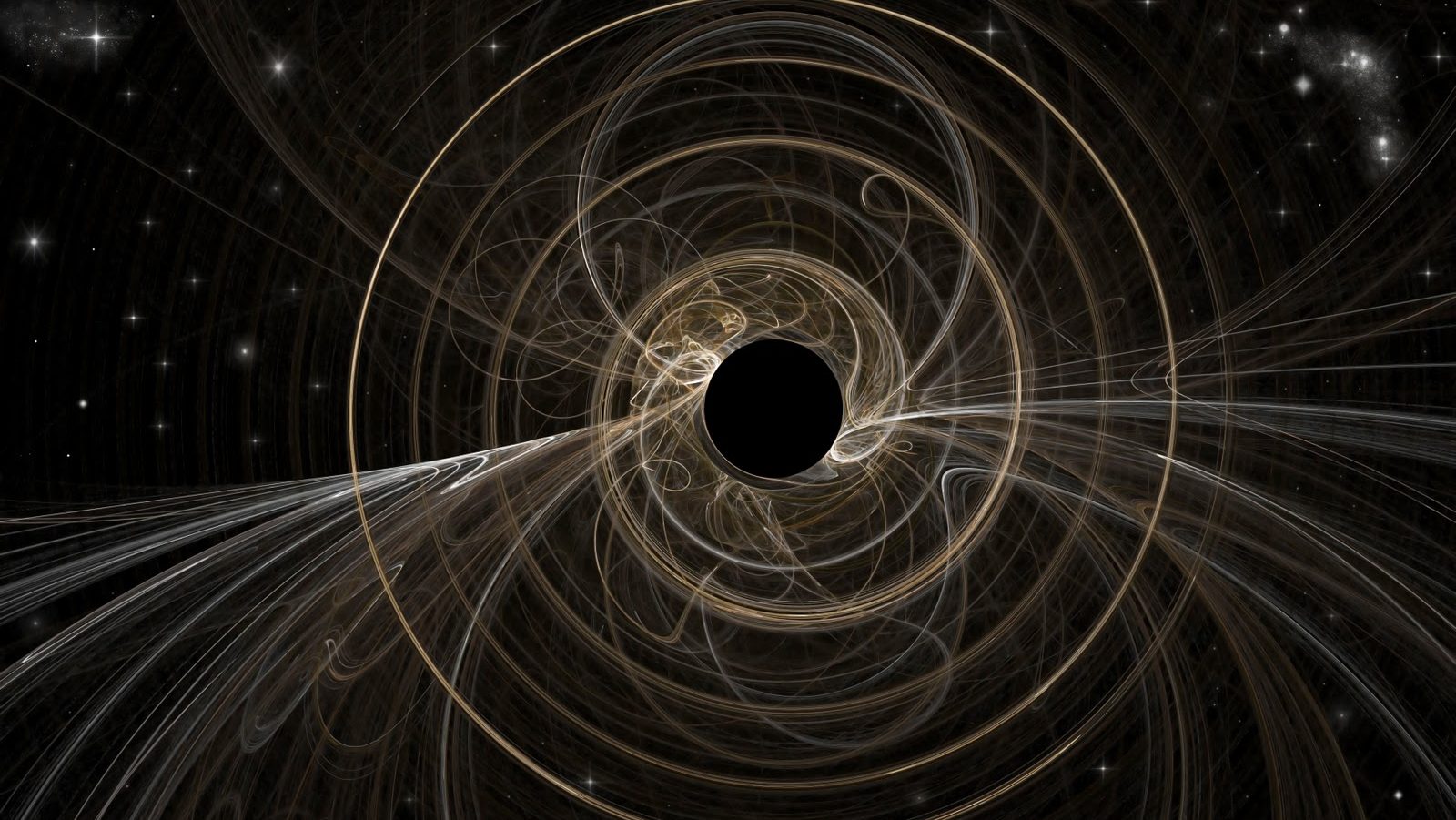
But we have to keep in mind that what we think is true today may turn out to be superseded, down the road, by a scientific truth that paints a picture that’s a better approximation of reality than we have today. Some of the things that appear true today:
- that dark matter doesn’t bind together, interact, or release energy,
- that dark energy doesn’t evolve and won’t decay,
- that black holes simply collapse, live a long time, and then decay back into quanta via Hawking radiation,
- and that there are no new fundamental interactions, forces, particles and/or reactions over what is known at present,
may turn out to be false when more knowledge is obtained.
We have to keep our minds open to all possibilities that haven’t yet been ruled out by observation and experiment, and must continue to challenge the assumptions that are too easy to let pass without scrutiny: that our best answer today will continue to be our best answer in the future. Our Universe appears headed toward equilibrium right now, but that doesn’t necessarily mean that’s where it will wind up. As Carl Sagan so wisely reminded us many years ago:
“At the heart of science is an essential balance between two seemingly contradictory attitudes: an openness to new ideas, no matter how bizarre or counterintuitive they may be, and the most ruthless skeptical scrutiny of all ideas, old and new. This is how deep truths are winnowed from deep nonsense.”
Our Universe may someday achieve a heat death and a state of maximum entropy: a true state of thermal equilibrium. But as long as there are new measurements to make and new questions to ask and explore about phenomena whose nature is not fully understood, there will always be the tantalizing possibility that there’s a whole lot more out there, just waiting to surprise us.
Send in your Ask Ethan questions to startswithabang at gmail dot com!