This Is Why We Don’t Shoot Earth’s Garbage Into The Sun
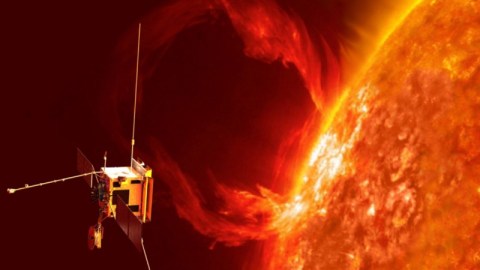
It would be the ultimate method for solving our pollution or hazardous/radioactive waste problems, but we’ll never do it. Here’s why.
Imagine our planet as it was for the first 4.55 billion years of its existence. Fires, volcanoes, earthquakes, tsunamis, asteroid strikes, hurricanes and many other natural disasters were ubiquitous, as was biological activity throughout our entire measured history. Most of the environmental changes that occurred were gradual and isolated; only in a few instances — often correlated with mass extinctions — were the changes global, immediate, and catastrophic.
But with the arrival of human beings, Earth’s natural environment has another element to contend with: the changes wrought upon it by our species. For tens of thousands of years, the largest wars were merely regional skermishes; the largest problems with waste led only to isolated disease outbreaks. But our numbers and technological capabilities have grown, and with it, a waste management problem. You might think a great solution would be to send our worst garbage into the Sun, but we’ll never make it happen. Here’s why.
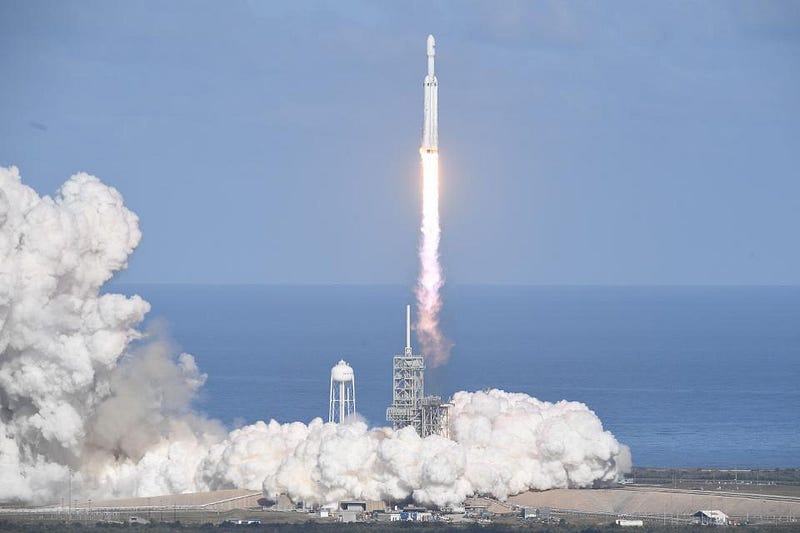
At present, there are a little more than 7 billion humans on the planet, and the previous century saw us at last become a spacefaring civilization, where we’ve broken the gravitational bonds that have kept us shackled to Earth. We’ve extracted valuable and rare minerals and elements, synthesized new chemical compounds, developed nuclear technologies, and produced new technologies that far exceed even the wildest dreams of our distant ancestors.
Although these new technologies have transformed our world and improved our quality of life, there are negative side-effects that have come along for the ride. We now have the capacity to cause widespread damage and destruction to our environment in a variety of ways, from deforestation to atmospheric pollution to ocean acidification and more. With time and care, the Earth will begin self-regulating as soon as we stop exacerbating these problems. But other problems just aren’t going to get better on their own on any reasonable timescale.
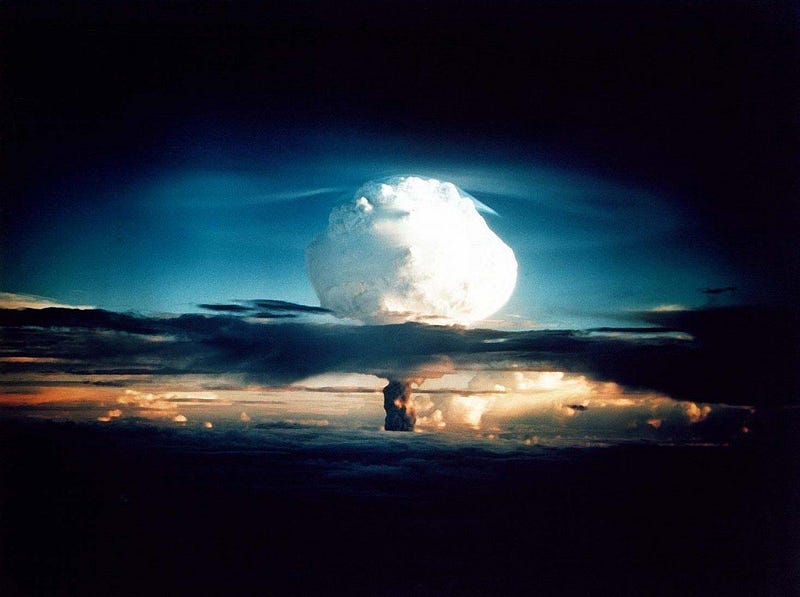
Some of what we’ve produced here on Earth isn’t merely a problem to be reckoned with over the short-term, but poses a danger that will not significantly lessen with time. Our most dangerous, long-term pollutants include nuclear by-products and waste, hazardous chemicals and biohazards, plastics that off-gas and don’t biodegrade, and could wreak havoc on a significant fraction of the living beings on Earth if they got into the environment in the wrong way.
You might think that the “worst of the worst” of these offenders should be packed onto a rocket, launched into space, and sent on a collision course with the Sun, where at last they won’t plague Earth anymore. (Yes, that was similar to the plot of Superman IV.) From a physics point of view, it’s possible to do so.
But should we do it? That’s another story entirely, and it begins with considering how gravitation works on Earth and in our Solar System.
Human beings evolved on Earth, grew to prominence on this world, and developed extraordinary technologies that our corner of the cosmos had never seen before. We all have long dreamed of exploring the Universe beyond our home, but only in the past few decades have we managed to escape the gravitational bonds of Earth. The gravitational pull exerted by our massive planet is only dependent on our distance from Earth’s center, which causes spacetime to curve and causes all objects on or near it — including humans — to constantly accelerate “downwards.”
There’s a certain amount of energy keeping any massive object bound to Earth: gravitational potential energy. However, if we move fast enough (i.e., impart enough kinetic energy) to an object, it can cross two important thresholds.
- The threshold of a stable orbital speed to never collide with Earth: about 7.9 km/s (17,700 mph).
- The threshold of escaping from Earth’s gravity entirely: 11.2 km/s (25,000 mph).
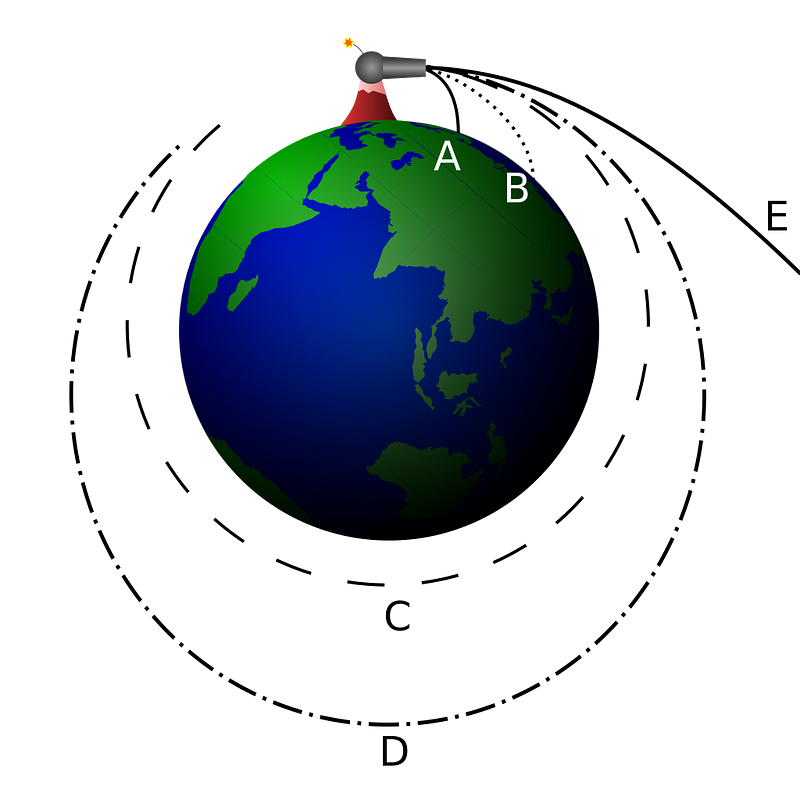
For comparison, a human at the equator of our planet, where Earth’s rotation is maximized, is moving only at about 0.47 km/s (1,000 mph), leading to the conclusion that we’re in no danger of escaping unless there’s some tremendous intervention that changes the situation.
Luckily, we’ve developed just such an intervention: rocketry. To get a rocket into Earth’s orbit, we require at least the amount of energy it would take to accelerate that rocket to the necessary threshold speed we mentioned earlier. Humanity has been doing this since the 1950s, and once we’ve escaped from Earth, there was so much more to see occurring on larger scales.
Earth isn’t stationary, but orbits the Sun at approximately 30 km/s (67,000 mph), meaning that even if you escape from Earth, you’ll still find yourself not only gravitationally bound to the Sun, but in a stable elliptical orbit around it.
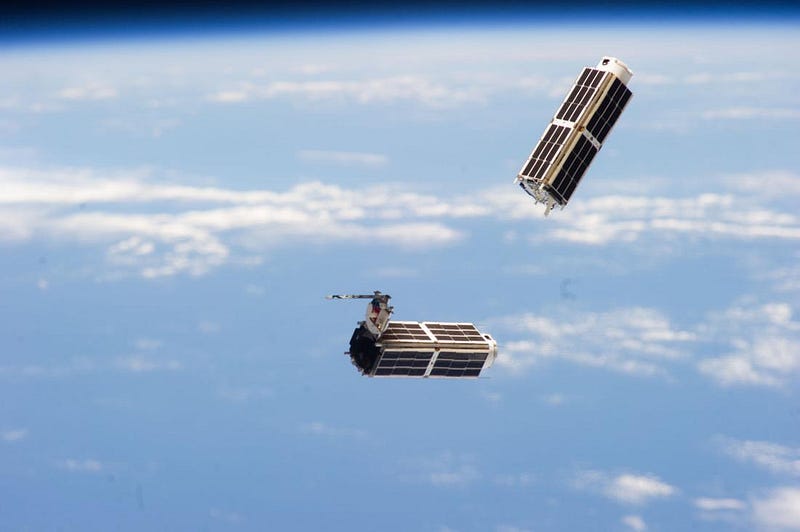
This is a key point: you might think that here on Earth, we’re bound by Earth’s gravity and that’s the dominant factor as far as gravitation is concerned. Quite to the contrary, the gravitational pull of the Sun far exceeds the gravitational pull of Earth! The only reason we don’t notice it is because you, me, and the entire planet Earth are in free-fall with respect to the Sun, and so we’re all accelerated by it at the same relative rate.
If we were in space and managed to escape from Earth’s gravity, we’d still find ourselves moving at approximately 30 km/s with respect to the Sun, and at an approximate distance of 150 million km (93 million miles) from our parent star. If we wanted to escape from the Solar System, we’d have to gain about another 12 km/s of speed to reach escape velocity, something that a few of our spacecraft (Pioneer 10 and 11, Voyager 1 and 2, and New Horizons) have already achieved.
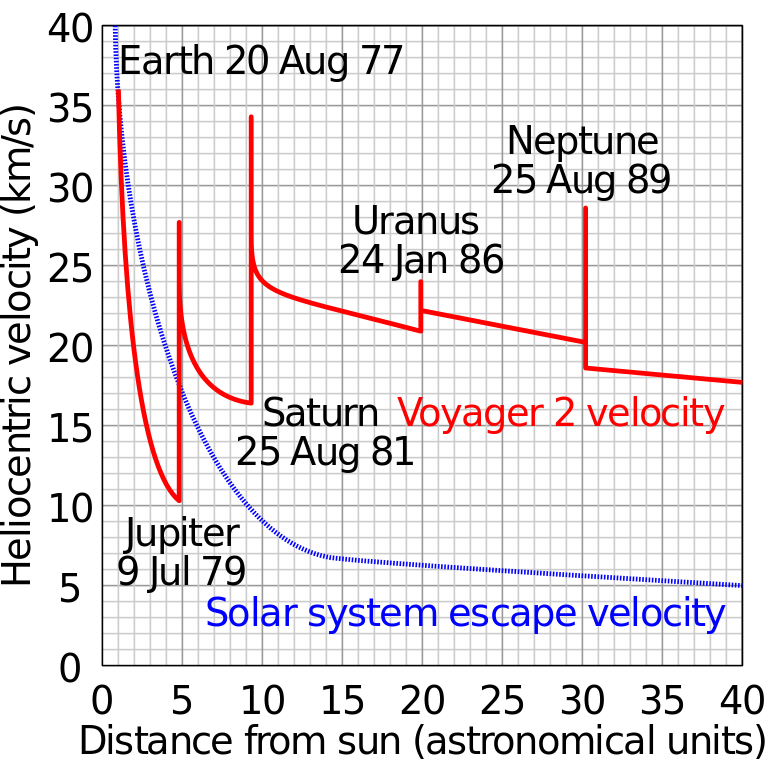
But if we wanted to go in the opposite direction, and launch a spacecraft payload into the Sun, we’d have a big challenge at hand: we’d have to lose enough kinetic energy that a stable elliptical orbit around our Sun would transition to an orbit that came close enough to the Sun to collide with it. There are only two ways to accomplish this:
- Bring enough fuel with you so that you can decelerate your payload sufficiently (i.e., have it lose as much of its relative speed with respect to the Sun as possible), and then watch your payload gravitationally free-fall into the Sun.
- Configure enough fly-bys with the innermost planets of our Solar System — Earth, Venus and/or Mercury — so that the orbiting payload gets de-boosted (as opposed to the positive boosts that spacecraft like Pioneer, Voyager, and New Horizons received from gravitationally interacting with the outer planets) and eventually comes close enough to the Sun that it gets devoured.
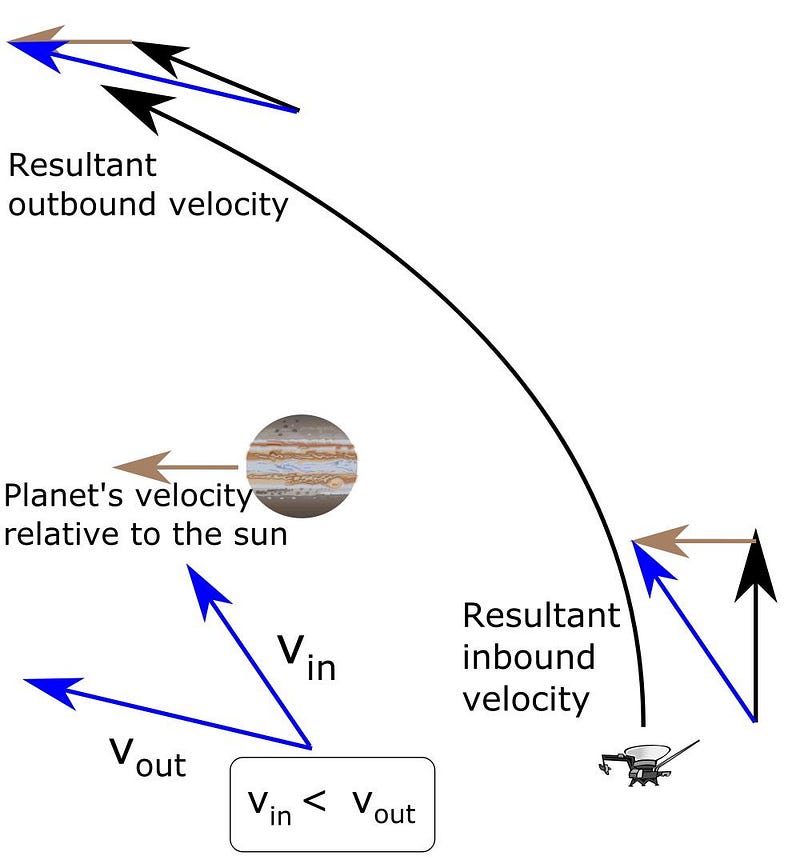
The first option, in reality, requires so much fuel that it’s practically impossible with current (chemical rocket) technology. If you loaded up a rocket with a massive payload, like you might expect for all the hazardous waste you want to fire into the Sun, you’d have to load it up with a lot of rocket fuel, in orbit, to decelerate it sufficiently so that it’d fall into the Sun. To launch both that payload and the additional fuel requires a rocket that’s larger, more powerful and more massive than any we’ve ever built on Earth by a large margin.
Instead, we can use the gravity assist technique to either add or remove kinetic energy from a payload. If you approach a large mass (like a planet) from behind, fly in front of it, and get gravitationally slingshotted behind the planet, the spacecraft loses energy while the planet gains energy. If you go the opposite way, though, approaching the planet from ahead, flying behind it and getting gravitationally slingshotted back in front again, your spacecraft gains energy while removing it from the orbiting planet.
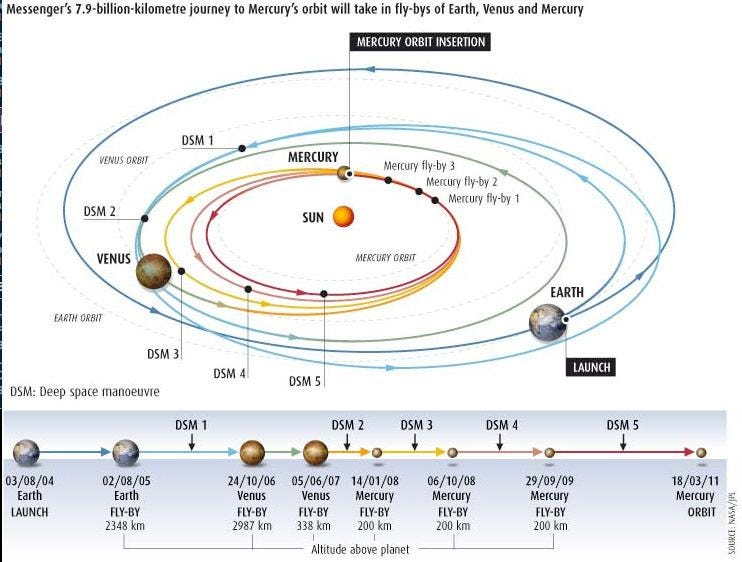
Two decades ago, we successfully used this gravitational slingshot method to successfully send an orbiter to rendezvous and continuously image the planet Mercury: the Messenger mission. It enabled us to construct the first all-planet mosaic of our Solar System’s innermost world. More recently, we’ve used the same technique to launch the Parker Solar Probe into a highly elliptical orbit that will take it to within just a few solar radii of the Sun.
A carefully calculated set of future trajectories is all that’s required to reach the Sun, so long as you orient your payload with the correct initial velocity. It’s difficult to do, but not impossible, and the Parker Solar Probe is perhaps the poster child for how we would, from Earth, successfully launch a rocket payload into the Sun.
https://www.youtube.com/watch?v=hKjcbmlAaYY
Keeping all this in mind, then, you might conclude that it’s technologically feasible to launch our garbage — including hazardous waste like poisonous chemicals, biohazards, and even radioactive waste — but it’s something we’ll almost certainly never do.
Why not? There are currently three barriers to the idea:
- The possibility of a launch failure. If your payload is radioactive or hazardous and you have an explosion on launch or during a fly-by with Earth, all of that waste will be uncontrollably distributed across Earth.
- Energetically, it costs less to shoot your payload out of the Solar System (from a positive gravity assist with planets like Jupiter) than it does to shoot your payload into the Sun.
- And finally, even if we chose to do it, the cost to send our garbage into the Sun is prohibitively expensive at present.
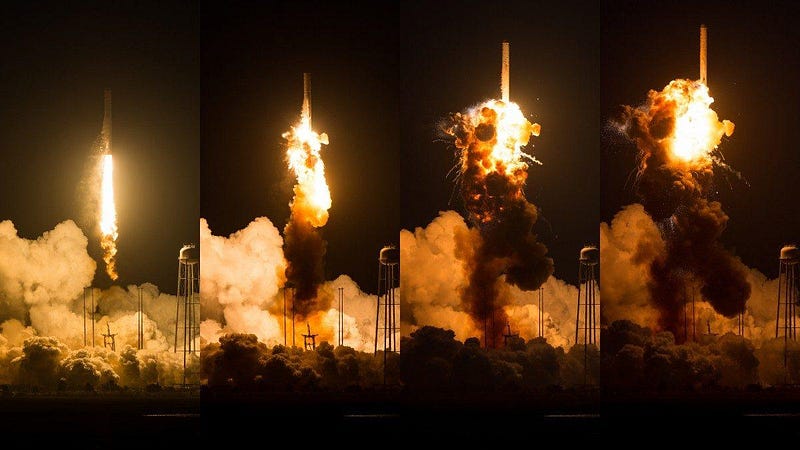
The most successful and reliable space launch system of all time is the Soyuz rocket, which has a 97% success rate after more than 1,000 launches. Yet a 2% or 3% failure rate, when you apply that to a rocket loaded up with all the dangerous waste you want launched off of your planet, leads to the catastrophic possibility of having that waste spread into the oceans, atmosphere, into populated areas, drinking water, etc. This scenario doesn’t end well for humanity; the risk is too high.
Considering that the United States alone is storing about 60,000 tons of high-level nuclear waste, it would take approximately 8,600 Soyuz rockets to remove this waste from the Earth. Even if we could reduce the launch failure rate to an unprecedented 0.1%, it would cost approximately a trillion dollars and, with an estimated 9 launch failures to look forward to, would lead to over 60,000 pounds of hazardous waste being randomly redistributed across the Earth.
Unless we’re willing to pay an unprecedented cost and accept the near-certainty of catastrophic environmental pollution, we have to leave the idea of shooting our garbage into the Sun to the realm of science fiction and future hopeful technologies like space elevators. It’s undeniable that we’ve made quite the mess on planet Earth. Now, it’s up to us to figure out our own way out of it.
Ethan Siegel is the author of Beyond the Galaxy and Treknology. You can pre-order his third book, currently in development: the Encyclopaedia Cosmologica.