This Is How We Will Successfully Image A Black Hole’s Event Horizon
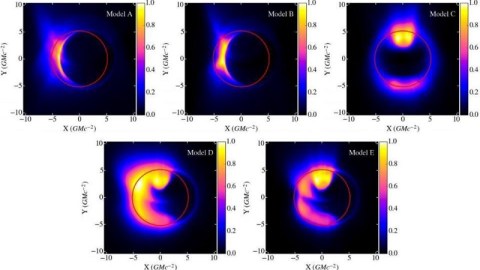
As the Event Horizon Telescope prepares to release its first results, we can expect not just one, but two black hole images.
What does a black hole actually look like? For generations, scientists argued over whether black holes actually existed or not. Sure, there were mathematical solutions in General Relativity that indicated they were possible, but not every mathematical solution corresponds to our physical reality. It took observational evidence to settle that issue.
Owing to matter orbiting and infalling around black holes, both stellar-mass versions and the supermassive versions, we’ve detected the X-ray emissions characteristic of their existences. We found and measured the motions of individual stars that orbit suspected black holes, confirming the existence of massive objects at the centers of galaxies. If only we could directly image these objects that emit no light themselves, right? Amazingly, that time is here.
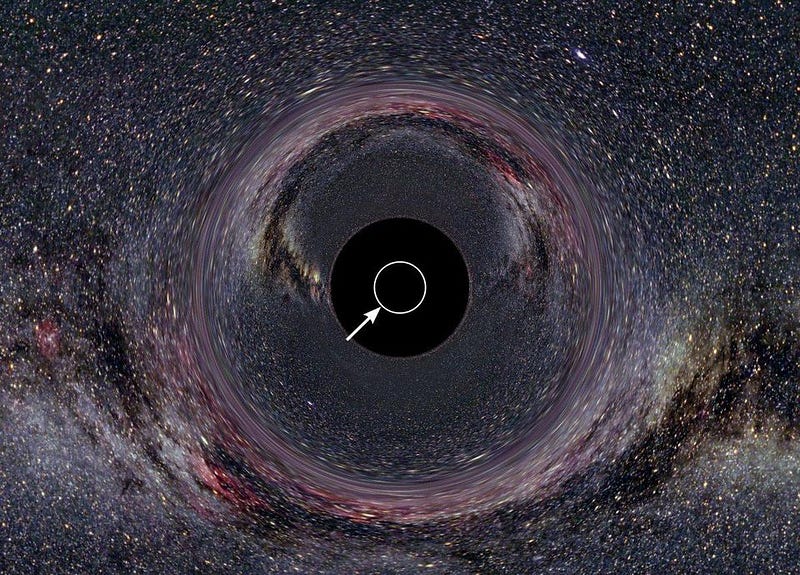
In theory, a black hole is an object that cannot hold itself up against gravity. Whatever outward forces there are — including radiation, nuclear and electromagnetic forces, or even quantum degeneracy arising from the Pauli exclusion principle — must be equal and opposite to the inward force of gravity, or collapse is inevitable. If you get that gravitational collapse, you will form an event horizon.
An event horizon is the location where the fastest speed attainable, the speed of light, is exactly equal to the speed necessary to escape from the gravity of the object inside. Outside of the event horizon, light can escape. Inside the event horizon, light cannot. It’s for this reason that black holes are expected to be black: the event horizon should describe a dark sphere in space where there should be no light detectable of any type.
We see objects in the Universe that are so consistent with the expectations for a black hole that there are no good theories, at all, for what else they might be. Furthermore, we can calculate how large these event horizons should both physically be for a black hole (proportional to a black hole’s mass) and how large they should appear in General Relativity (about 2.5 times the diameter of the physical extent).
As viewed from Earth, the largest apparent black hole should be Sagittarius A*, which is the black hole at the center of the Milky Way, with an apparent size of approximately 37 micro-arc-seconds. At 4 million solar masses and a distance of around 27,000 light years, it should appear larger than any other. But the second largest one? That’s at the center of Messier 87, over 50 million light years away.
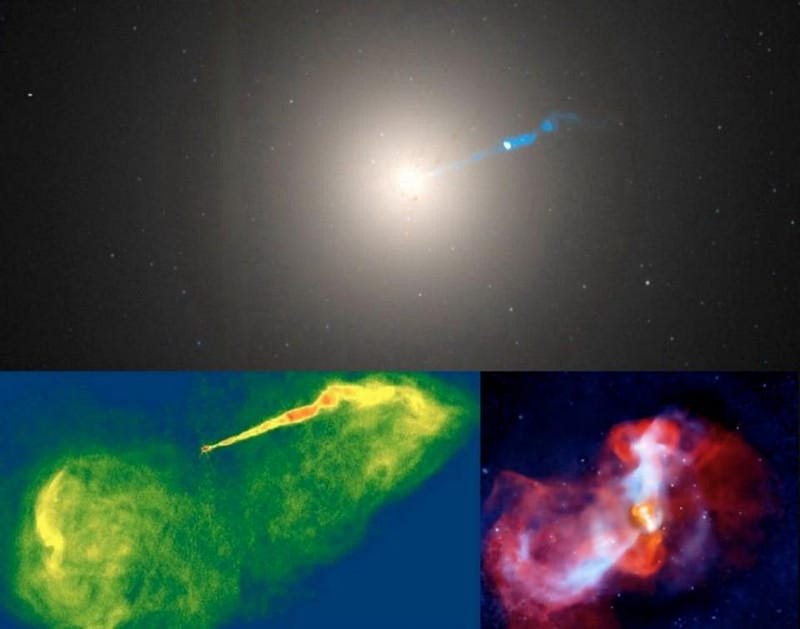
The reason that black hole is so huge? Because even at that incredible distance, it’s over 6 billion solar masses, meaning it should appear as roughly 3/4ths the size of the Milky Way’s black hole. Black holes are well-known for emitting radiation in the radio portion of the spectrum, as matter accelerates around the event horizon, but this gives us a brilliant way to attempt to view it: through very-long-baseline interferometry in the radio portion of the spectrum.
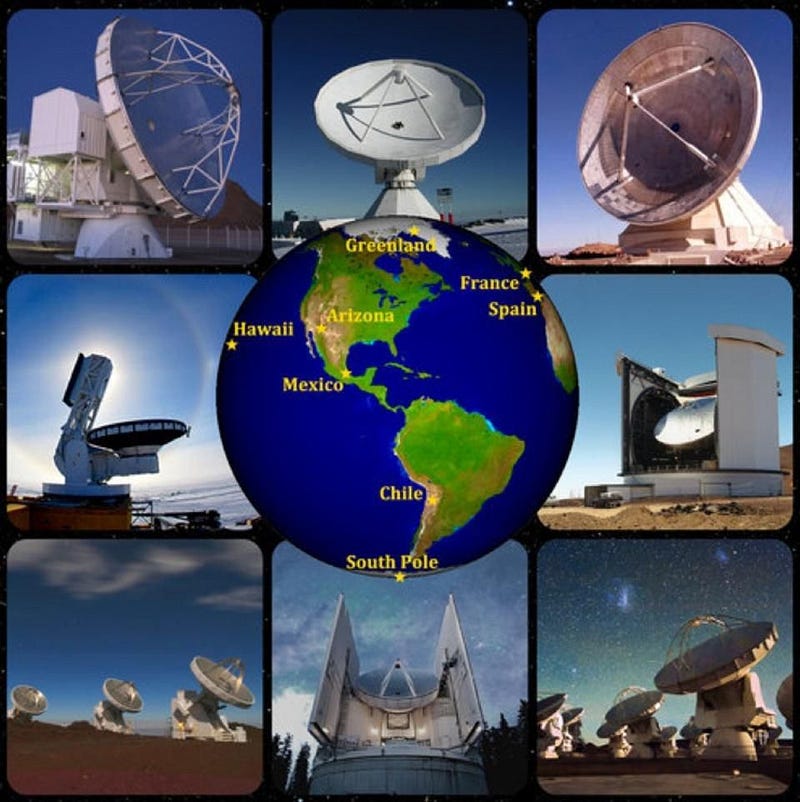
All we need, to make that happen, is an enormous array of radio telescopes. We need them all over the globe, so that we can take temporally simultaneous measurements of the same objects from locations up to 12,700 kilometers (8,000 miles) away: the diameter of Earth. By taking these multiple images, we can piece together an image — so long as the source we’re imaging is radio-bright enough — as small as 15 micro-arc-seconds in size.
The Event Horizon Telescope (EHT) is exactly such an array, and it’s not only been taking data from all over the world (including in Antarctica) for years, it’s already taken all the images necessary of Sagittarius A* and of Messier 87 you could hope for. All that’s left, now, is to process the data and construct the images for the general public to view.
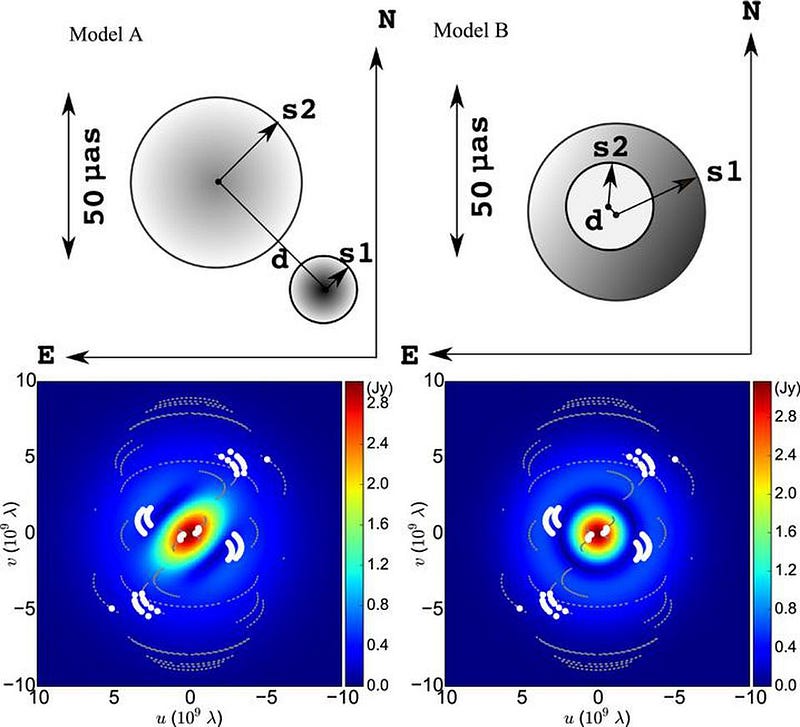
We’ve already taken the data necessary to create the first black hole images ever, so what’s the hold up? What are we poised to learn? And what might surprise us about what the Universe has in store?
In theory, the event horizon should appear as an opaque black circle, letting no light from behind it through. It should display a brightening on one side, as matter accelerates around the black hole. It should appear 250% the size that General Relativity predicts, due to the distortion of spacetime. And it should happen because of a spectacular network of telescopes, in unison, all viewing the same object.
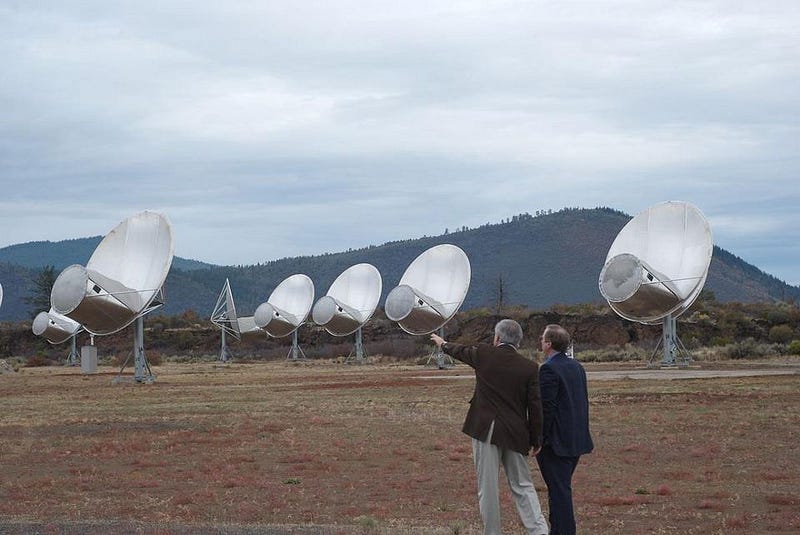
Normally, the resolution of your telescope is determined by two factors: the diameter of your telescope and the wavelength of light you’re using to view it. The number of wavelengths of light that fit across your dish determines the optimal angular diameter you can resolve. Yet if this were truly our limits, we’d never see a black hole at all. You’d need a telescope the diameter of the Earth to view even the closest ones in the radio, where black holes emit the strongest and most reliably.
But the trick of very-long baseline interferometry is to view extremely bright sources, simultaneously, from identical telescopes separated by large distances. While they only have the light-gathering power of the surface area of the individual dishes, they can, if a source is bright enough, resolve objects with the resolution of the entire baseline. For the Event Horizon Telescope, that baseline is the diameter of the Earth.
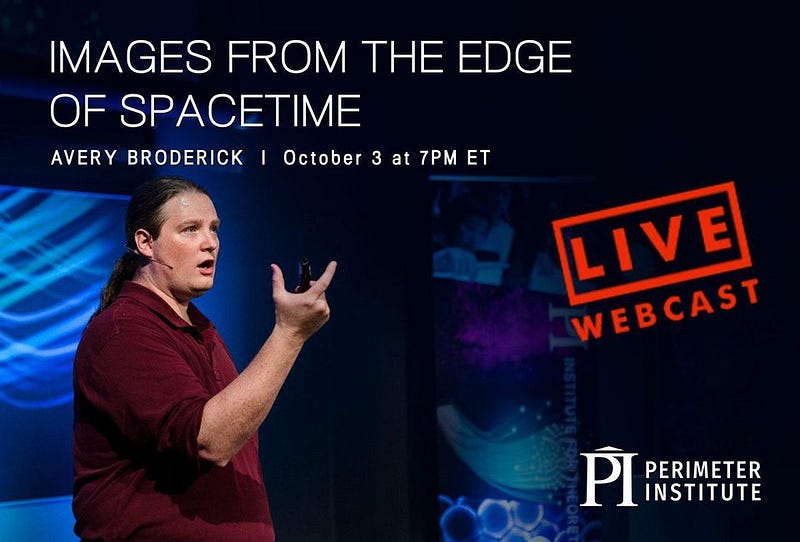
I’m so pleased that the Event Horizon Telescope, and imaging the event horizon of a black hole directly, will be the topic of October 3rd’s Perimeter Institute public lecture: Images from the Edge of Spacetime, by Avery Broderick.
The live blog is now complete, having aired initially at 7 PM Eastern Time (4 PM Pacific Time), and you can follow along by watching the video below. Watch the talk any time, and follow along with the live blog that follows!
(All updates, below, will have the timestamps in bold in Pacific time, with screenshots where appropriate from the lecture itself.)
3:50 PM: Welcome! Let’s start the Live Blog a little early, so we can give you a bit of background.
The biggest thing you have to realize, when it comes to imaging a black hole’s event horizon, is that we’re not looking for light, but the absence of light. When you look at a galaxy’s center, you’re going to see a ton of light, coming from all the matter located there. What the event horizon of a black hole gives you, spectacularly, is a shadow: a region wherein any light coming from behind it gets absorbed and swallowed. The key to imaging the event horizon is to see the light, behind the black hole, that emerges from around the horizon itself.
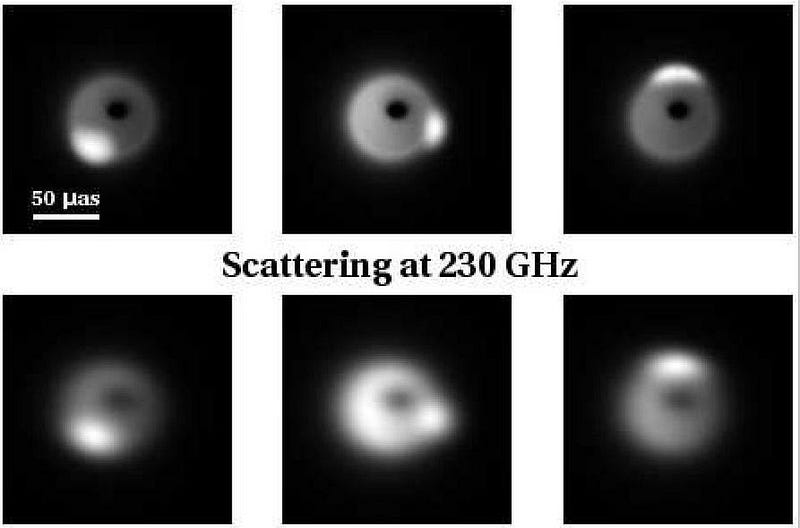
3:54 PM: What’s an incredibly exciting possibility, that hopefully we’ll get to hear more about in this lecture, is what we might see if something is flawed in Einstein’s theory of general relativity. Of course we expect Einstein to be right; general relativity has never led us astray yet, not in any experiment, measurement, or at any level of detail. But if the event horizon is a different size, opacity, or shape than what we predict, or doesn’t even exist at all, that could lead us to a revolution in physics. Quantum gravitational effects, for example, shouldn’t be important here. But if they are… well, that’s part of why we look!
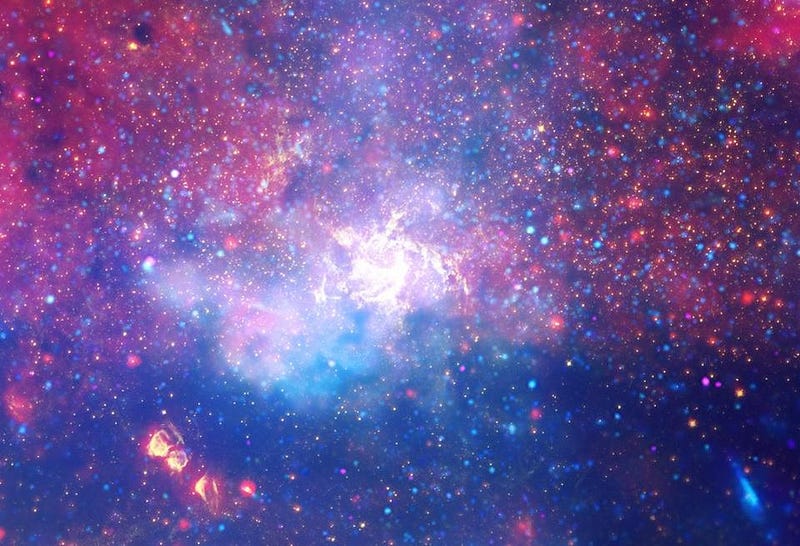
3:58 PM: I know we’re all hoping for an answer to the biggest question we have: what does the event horizon look like? That’s why we have the telescope array, after all, doing what it’s doing. But take a look at the multiwavelength image above. We have to see through all of that radiation, and prevent it from being a foreground contaminant, to image the event horizon of the black hole itself.
It’s important to appreciate how much of the Universe we have to see through, as though it were transparent (and it’s not 100% transparent), just to have a shot at the event horizon itself. Today, I’m hoping we learn exactly how we can do this, and why we’re so confident that the EHT will get us there. Remember, the Milky Way’s black hole, and all black holes, are radio-loud objects!
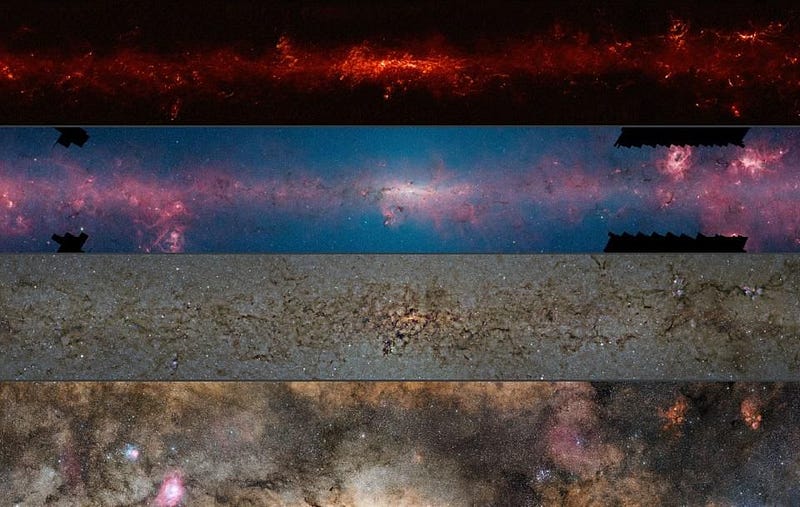
4:01 PM: Before the lecture starts, and it’s about to begin, here’s one last thing: this is the center of the Milky Way in four independent wavelengths. There’s a lot going on there, and we’re looking for an object that’s roughly the size of Jupiter’s orbit around the Sun. Are you not impressed at the ambition of the EHT? You should be impressed!!
4:04 PM: If you’re wondering why we don’t go for a closer black hole than the Milky Way’s center, because there are closer ones, it’s because the size of a black hole is dependent on its mass and its distance. Double the mass means double the radius; double the distance means half the radius. The second most massive black hole in the Milky Way that we’ve ever found is thousands of times less massive than the one at our galaxy’s center, but only about 10–20 times closer. This is why we go for bigger rather than closer!
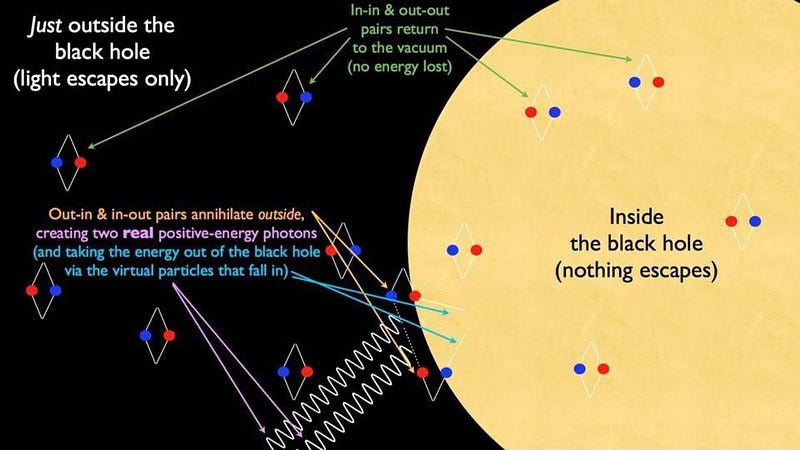
4:08 PM: “Black holes are objects into which things go, and don’t come out.” That is a solid definition of a black hole, which Avery gave… to first order. This should be true for every black hole in our Universe, but give it time. After about 10²⁰ years, perhaps a billion (or ten) times the age of our Universe, they’ll start to radiate, via Hawking radiation, faster than it can absorb any matter that surrounds it. They will shrink, and when they do, that will herald their disappearance.
On long enough timescales, things will come out, albeit not from inside the black hole, but from the curved spacetime outside of it.
4:10 PM: Avery says if you crush the Sun down to 3 km, it would become a black hole. Crush the Earth to 1 cm, and it’s a black hole. Crush a human, and it’s about 10^-11 times the width of a proton. (This is a correction to Avery’s number.)
And crush the Universe down to… approximately the size of the Universe itself, and it’ll be a black hole? Be careful here; the Universe is expanding, and full of dark energy, and that changes the equation tremendously. Our Schwarzschild solution, a great approximation for real black holes, no longer applies here. (I hope Avery gets this right when he gets there!)
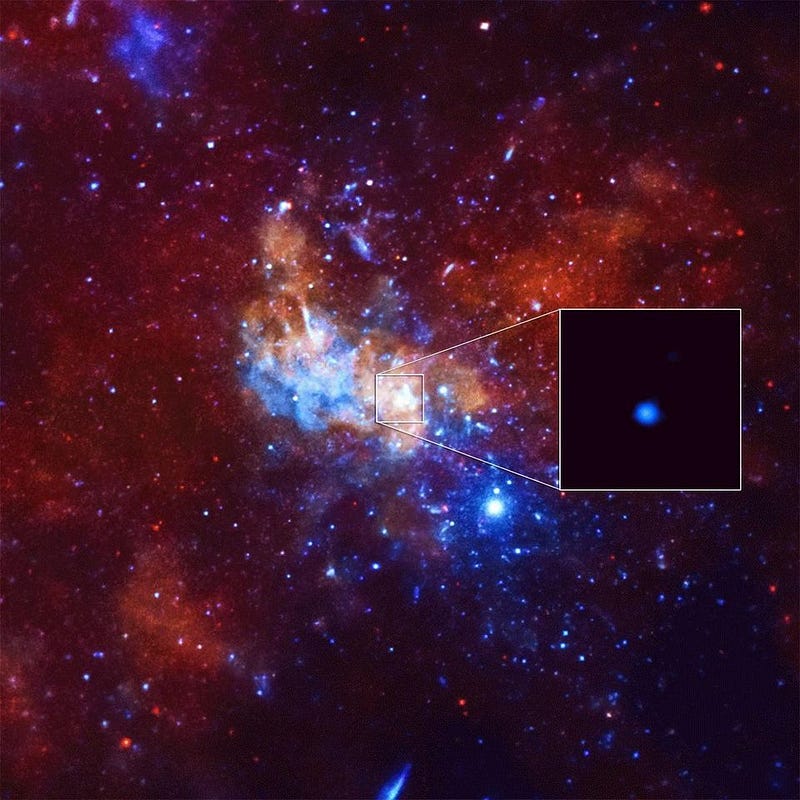
4:14 PM: Looking at supermassive black holes is fantastic; you get to see, in the radio, these massive lobes.
But the image above, that I’ve chosen, is in the X-ray! Black holes are powerful all across the electromagnetic spectrum. We can see their effects because, as Avery correctly notes, the matter expelled from the black holes changes their environments.
4:17 PM: Avery makes the point that the Universe is complicated, but black holes are simple. And this is true, so long as you’re looking at their macro-properties. But there is a huge amount of theoretical motivation to assume that what a black hole is made out of matters! If you made a black hole out of 10⁵⁵ neutrons or 10⁵⁵ antineutrons, there should be a difference. Not in General Relativity, but in terms of information and quantum numbers.
Does this actually matter? We’re not sure, and the EHT won’t teach us that. There are many questions we ought to remember that physics has left to resolve, no matter what the answers the EHT (or any experiment) can give us.
4:20 PM: Avery brings up a fun acronym: ISCO. ISCO stands for innermost stable circular orbit. This is not the event horizon, but rather an orbit about three times the radius of the event horizon. There should be, therefore, an empty hole that’s in between ISCO and the event horizon, where no matter (stably) exists.
The innermost orbit for matter, and for photons, and for even the spacetime that starts to get dragged around (yes, this happens!), all affect what someone viewing the event horizon would actually see. Frame-dragging is a real effect in relativity, and cannot be ignored!
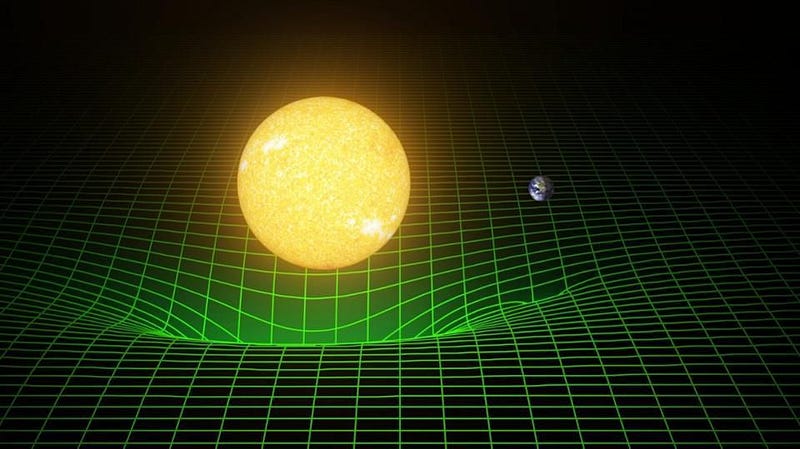
4:24 PM: I think this is a really important point that Avery only glosses over, but is a source of confusion for a lot of people in General Relativity. The curvature of spacetime is not determined by mass. Sure, no less a figure than Wheeler noted that matter tells spacetime how to curve; curved space tells matter how to move, but it’s more than that. The curvature of spacetime is determined by the presence, distribution, and density of both matter and energy. This includes energy of all forms: radiation, kinetic energy, and many quantities other than just mass.
Mass plays a major role, but it isn’t the only important thing as far as affecting spacetime goes.
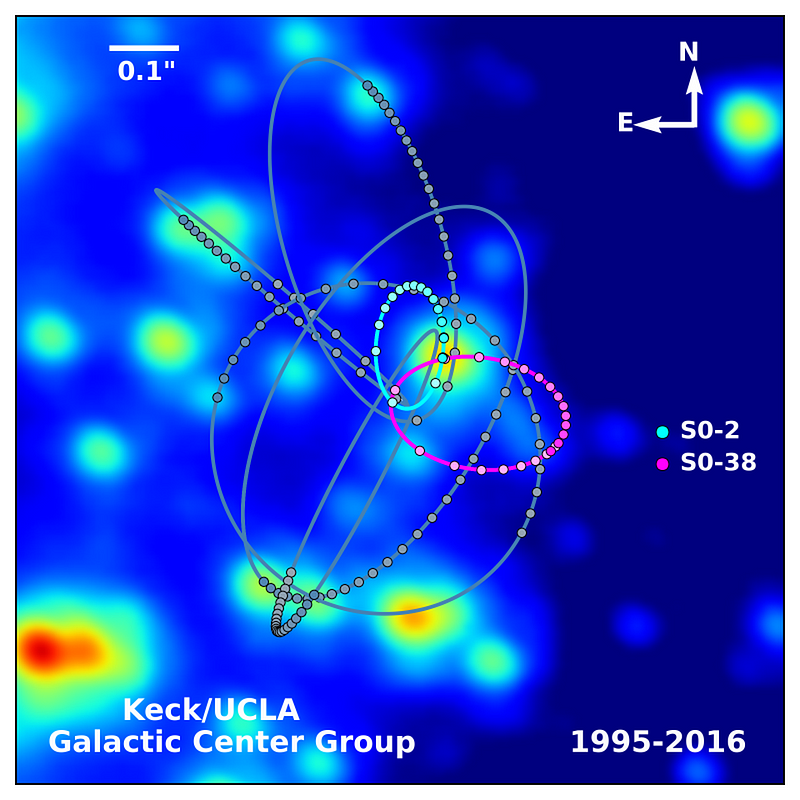
4:27 PM: I want to note something that Avery said at the 0:25 minute mark in his talk, asking if these objects with large masses and X-ray/radio emissions are, in fact, black holes? He then left the question hanging and didn’t answer.
But you know what? Except for crackpots on the internet, pretty much everyone now accepts that these objects are black holes, and it was Andrea Ghez’s group, at UCLA, that answered that question for us. You see stars, by looking in the infrared, orbiting a point of incredible mass, about 4 million solar masses. Yet no light (at least, in the infrared) comes from that mass.
Why? Because there is no explanation for it other than a black hole. That’s a black hole, folks, and with supreme confidence we can look for it with a telescope like the EHT.
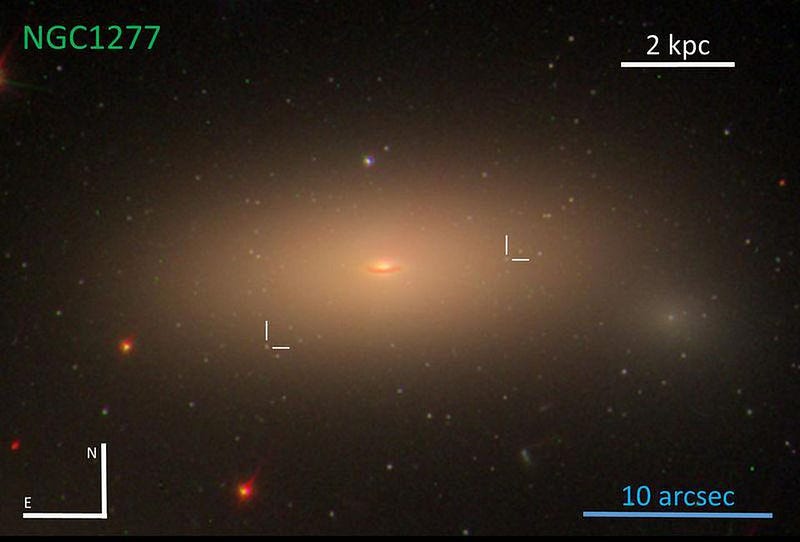
4:31 PM: There is a great graphic and a great conundrum in Avery’s talk. The largest black hole, as seen from Earth, is the one at the Milky Way’s center. The second largest is the one at M87. The fourth largest? The one at the center of Andromeda.
But the third largest is a weirdo: NGC 1277. It’s the size of the Milky Way, but appears to have a >10 billion solar mass black hole. This is controversial, but it’s a tantalizing possibility!
4:34 PM: Why is it so hard to resolve a black hole? Well, many reasons. We talked about resolution earlier, but that’s not the only one.
Not every galaxy is radio-loud, meaning you can’t see the shadow against the radio background if there is no background. (And so, sorry NGC 1277 fans, that’s out.) If a galaxy isn’t radio-transparent, because there’s too much foreground, it won’t be visible either. But if you’re limited by diffraction, which is the nature of your telescope, you can see the wavelength divided by your telescope’s diameter. You’d need a ~12 million meter diameter telescope to get the EHT’s resolution in the radio.
4:38 PM: So why does Avery, at the 0:36 mark in his talk, say you’d need a 5 km telescope, rather than a 12 million meter telescope, to see the black hole at the galaxy’s center?
Two reasons. Number one, the telescopes he’s talking about are optical/infrared, which have wavelengths that are around 1,000 times shorter than the radio wavelengths the EHT will look at. (This is good; the plane of the Milky Way, which includes the galactic center, is opaque to visible light!)
Number two, you want better resolution than the thing you’re trying to image. Otherwise, it’s just one pixel, and you can’t learn what you want to learn about an event horizon from just one pixel!
4:45 PM: His analogy with Fourier series isn’t really doing it for me. If you wonder, “how can you use multiple telescopes to get the resolution I need” to reconstruct an image, it is highly dependent on what you’re looking at. Always, more telescopes covering more area at more locations are better.
But if you only have two telescopes, you can still do incredible thing, like the Large Binocular Telescope Observatory (LBTO) did just a few years ago, when they imaged erupting volcanoes on Jupiter’s Moon, Io, while another of its moons (Europa) eclipsed it. Pretty incredible!
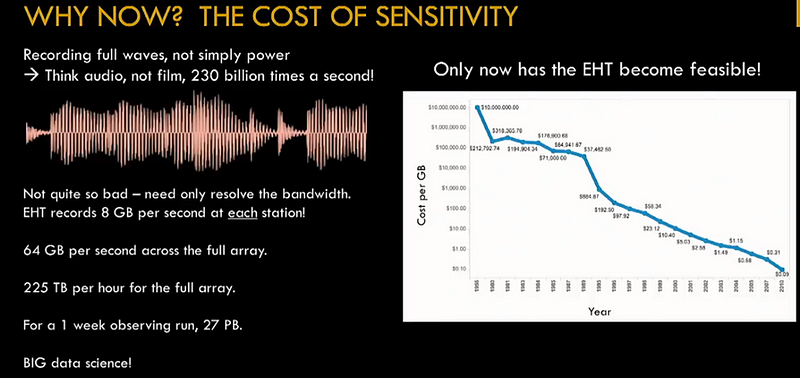
4:49 PM: So what took us so long to build the EHT? After all, we’ve had telescopes and planet Earth for a really, really long time, and we’ve been capable of taking these images. But it requires a whole lot of data. Writing down enough (and the right kinds of) data, fast enough, and then bringing them together with enough computational power to analyze them, is only now, for the first time, possible. If we had tried even a decade ago to build and run the EHT, it would not have been possible.
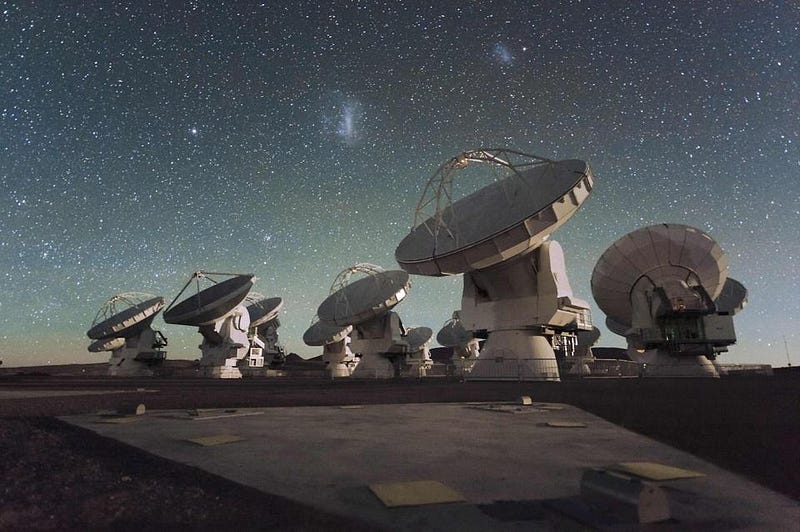
4:51 PM: Avery says the biggest advance was the addition of ALMA to the EHT array. And ALMA is so, so fantastic. A piece of the array is shown, above, but check out below, where ALMA has taken some pretty spectacular, high-resolution images of… well, planets forming around young stars, like nothing else ever has, even up through today.
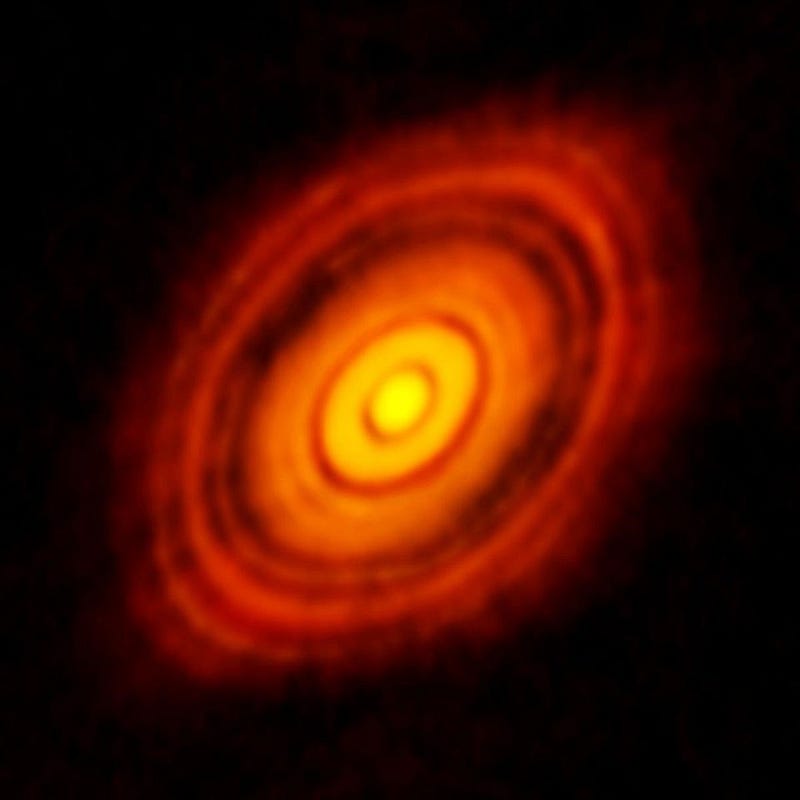
4:53 PM: And now, at last, at the 0:51 minute mark of the talk, we get the real reason why all this analysis takes so long. There are different atmospheric phase delays that include calibration, calculation, mistakes and re-calculation, of 27 Petabytes of data, from all the different stations.
Computational time is often a joke, but that’s the hold up. He has no images to show, because there are no final-version, mistake-free images available. Early 2019, maybe, is what he says we can look forward to for the first images.
4:54 PM: Be patient, EHT fans! Be pleased they are taking the time to get it right!
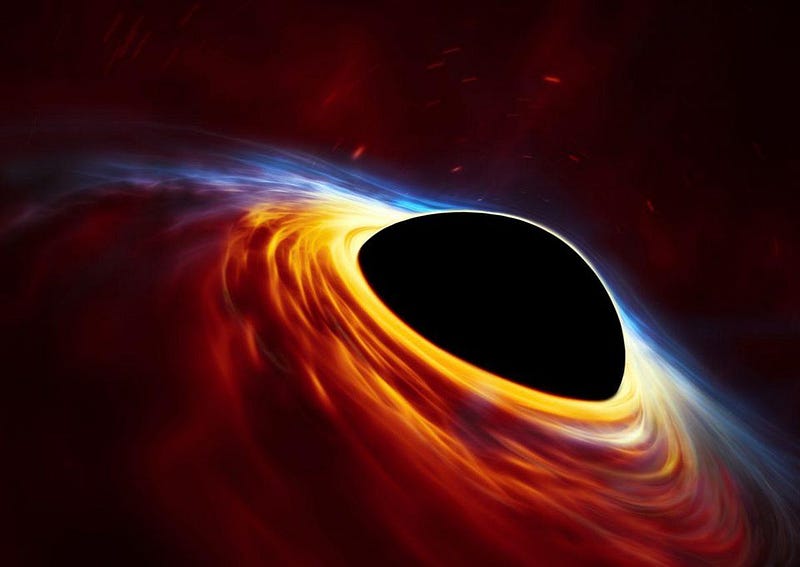
4:58 PM: Avery has just made an argument for why black holes must exist, and the objects at the centers of the Milky Way and M87 must be one. (Or, two, more accurately.) If you have stuff that falls onto a central accreting body, it will heat up and shine. But if they run into a hard object that doesn’t have an event horizon, it will heat up and shine upon impact. If you had impact emission, it would show up.
There was no emission, which should theoretically show up in the infrared. The lack of this would push above the infrared limits, and it’s not there!
Bam!
And therefore, black hole. It can’t be large and cool, and isn’t hot enough to be a non-black-hole. QED.
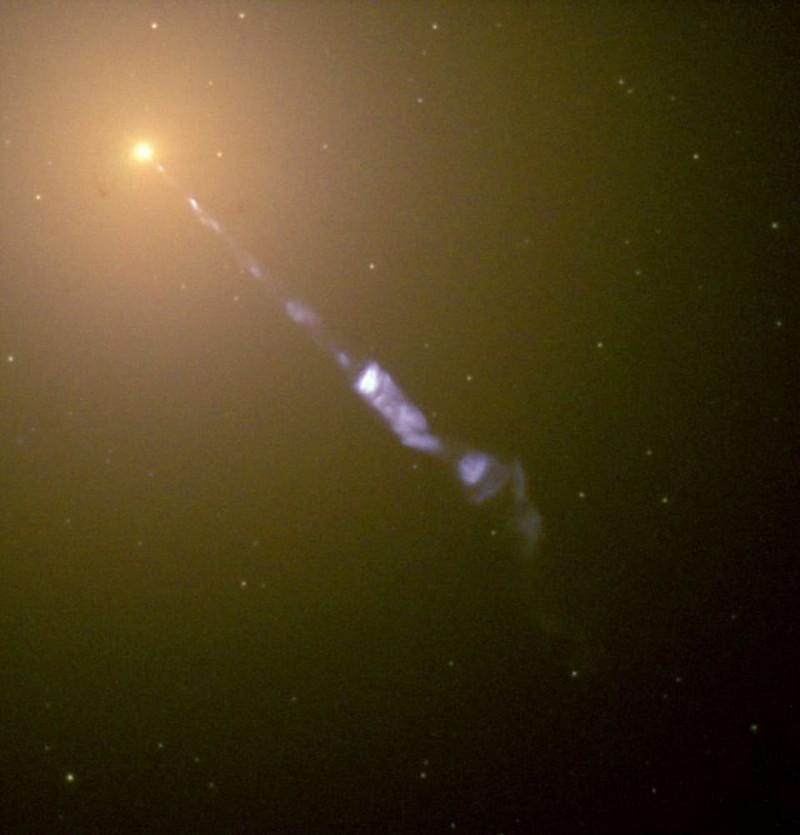
5:02 PM: So how do you measure the mass of a black hole? You measure the gas orbiting the central black hole; you measure the stars orbiting it. But you get two different numbers, and they disagree. They disagree by about a factor of 2 for M87, and (although most people don’t remember) they used to disagree for the Milky Way in the early 2000s. From X-rays, we estimated around 2.5–2.7 million solar masses, but from stars, we estimate 4 million solar masses.
Who’s right? My bet is on stars because the observations have fewer assumptions to translate into a mass, but the EHT should teach us which (if either) is correct!
5:04 PM: Avery contends these are the two black holes you’d want, ideally, to test black holes. They’re different; one is small and close, the other is large and farther; one is active with a big jet (M87) while the other is quiet; both have a large enough angular size to be resolved with a telescope the size of our planet, etc. And these are good arguments. But I’d still rather have a stellar mass black hole that happened to be within just a few light years to try out. Any help, Alpha Centauri?
(This is the first Perimeter talk I’ve seen that hasn’t been properly budgeted for time, BTW, so I’m sorry if any of you watching are bummed that it’s gone over.)
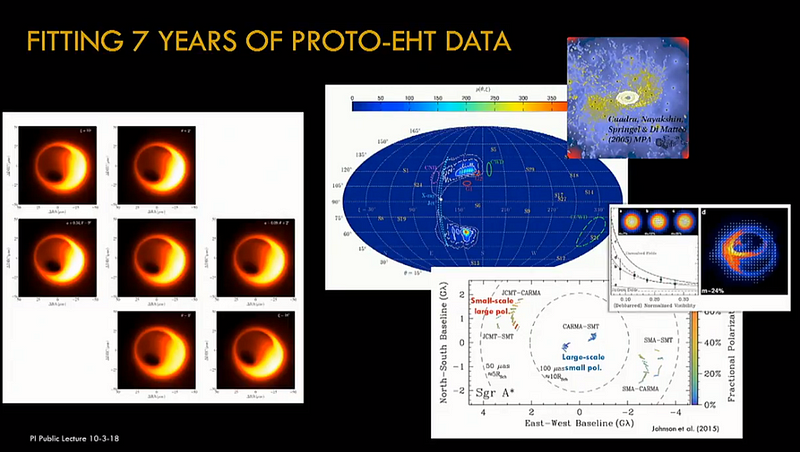
5:08 PM: Avery is talking about the early proto-EHT data, that took these first observations, and showed it was consistent with our models of black holes within General Relativity. But there is really so little that we get; we get info about mass, a little bit about rotation, and a little bit about the surrounding environment. Until we can see the horizon itself, and know its shape, we are very limited in what we can constrain.
Even Avery is disappointed with what we can say with Proto-EHT data.
5:10 PM: What will be very, very cool, that Avery is telling, is that there will be movies, not just images, that are interesting. On timescales of decades, black holes will jitter, similar to how Brownian motion works. Atoms and molecules bounce off of tiny particles under a microscope; that’s Brownian motion. Well, for the black hole at the galactic center, stars orbit and move closer-or-farther from the central black hole, and they gravitationally push it around!
5:12 PM: I would like to point out that this is why it’s so important to make your observations simultaneously in time to one another; you cannot reconstruct a single image from interferometry if you’re not looking at the same object anymore. Like Heraclitus said, “you can’t step into the same river twice.” Well, you can’t look at the same black hole twice, apparently.
That’s deep.
5:13 PM: Okay, for those of you watching, I’m just going to say that if you’re 73 minutes into a 60 minute talk, and you’re just now mentioning things like the “Bardeen-Petterson effect,” someone should start playing the “wrap-it-up” music.
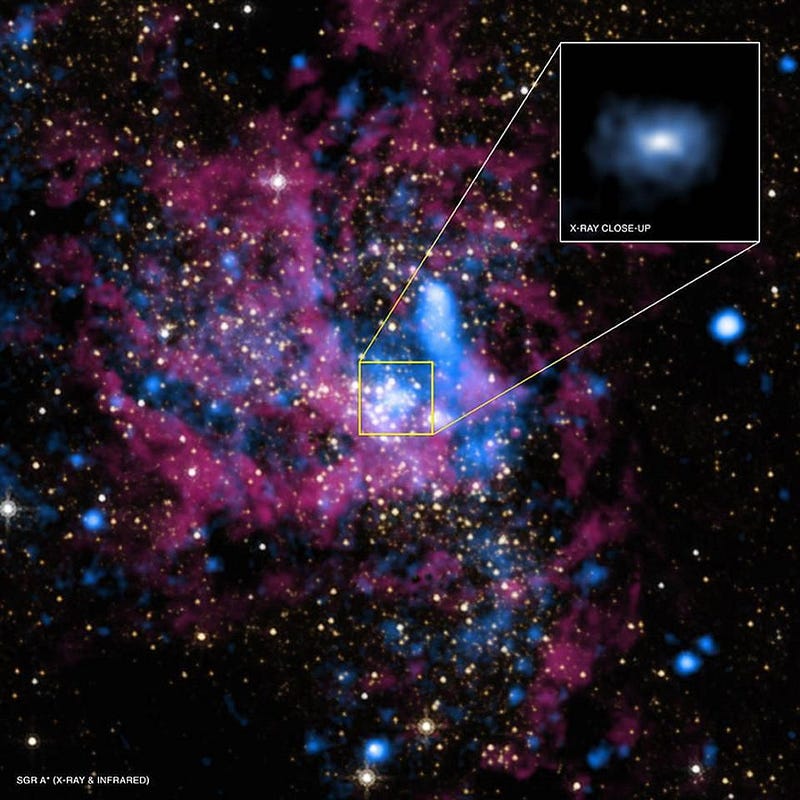
5:17 PM: Okay, this last thing is cool enough that I should mention here: flares at the center of the Milky Way’s black hole. They happen, and they typically last minutes.
But why? Are they turbulent features in the accretion disk? Or are they arising from the infall of matter, like “hot blobs” in the accretion flow, that flare out when they get accelerated and devoured?
Models of both are continuously being improved, and based not on the event horizon itself, but the luminous signals that come out around the outside of the event horizon, we might be able to tell them apart. Why does our black hole flare? The EHT might teach us.
5:20 PM: So, if you’ve made it this far, you probably watched the whole thing. So how do you sum it up?
- Black holes are real.
- We can see their effects and learn about it indirectly.
- They should have event horizons.
- The EHT should create an image of them with the data we have.
- It will take lots of time.
- And if we observe the light from outside of them, we might learn more about the environment of these black holes, and what causes transient events like flares.
And that’s the end! Q&A time!
5:22 PM: Fun question: what gets ejected from a black hole? What are these jets made out of? Where do they come from?
Avery gives the real answer: we don’t know. We think they’re filled with protons, nuclei, etc., and that’s Avery’s first answer. But they could be just electromagnetic (light) radiation. (Avery says that; most scientists, as I understand it, deem that incredibly unlikely.)
The follow-up is what is the jet’s effect on the black hole? While Avery is assuming equal-and-opposite bipolar jets, that assumption isn’t necessary. It’s like asking what effect a fly has as it splats on your semi truck’s windshield. It’s negligible.
5:25 PM: Avery’s final question is what made him want to study black holes? And the answer is… Star Trek! There’s no better way to end a live-blog than that, so live long and prosper, everyone, and I’ll see you next time!
Ethan Siegel is the author of Beyond the Galaxy and Treknology. You can pre-order his third book, currently in development: the Encyclopaedia Cosmologica.