Astronomers discover a great galactic genocide 11 billion years ago
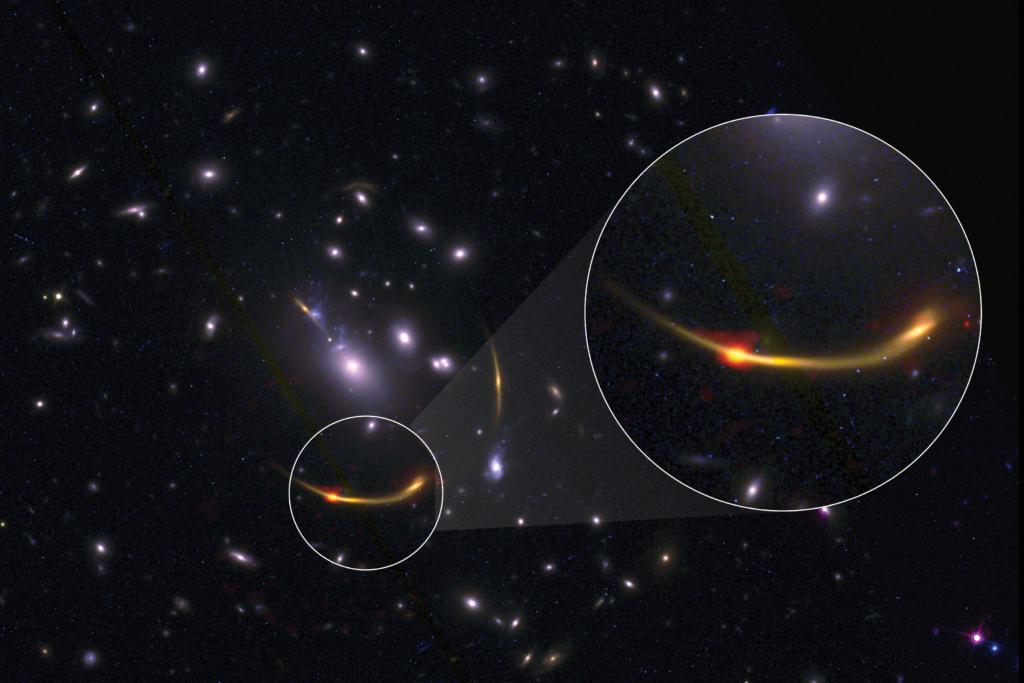
- The universe formed most of its stars when it was just two or three billion years old, with star-formation dropping ever since.
- New observations show that fully half of the most massive galaxies lost all their star-forming material by ~3 billion years of age, and they never got it back.
- This great galactic genocide poses an enormous puzzle for astronomers, who are still struggling to understand why.
Today, our view of the galaxies in the universe shows that they are full of a wide mix of stars. Most galaxies, much like our Milky Way, contain old stars, middle-aged stars, and young stars. They contain stars of all colors, from red to blue and everything in between. And, much like our Milky Way, most of the galaxies we see today are still forming stars at a slow but steady rate, with neutral gas contracting to trigger new episodes of star-formation, with the occasional galaxy undergoing a starburst, where enormous quantities of new stars are formed all at once.
But there are large numbers of galaxies out there — particularly toward the centers of large galaxy clusters — that contain only old stars, with practically no new ones. Their stars are almost all yellow, orange, or red, with practically no white or blue ones. Some of them, at the extremes, have not formed new stars in more than 12 billion years. It is as though some of the most massive, star-rich galaxies in the young universe suddenly “turned off” their star-formation, never to have it turn on again. In a groundbreaking new study, a number of these galaxies were discovered “turning off” for perhaps the final time, 11 billion years ago or more. Apparently, a great galactic genocide occurred just ~3 billion years after the Big Bang, and astronomers still do not quite understand how it happened.
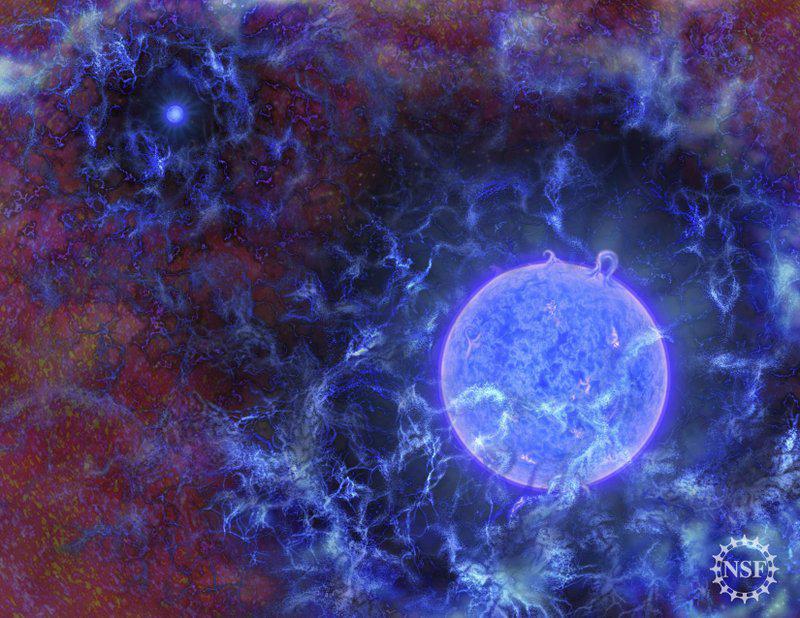
Credit: Nicole Rager Fuller / NSF
In the early stages of the hot Big Bang, there were not yet any stars. Matter needs considerable amounts of time to clump, cluster, and gravitate so that enough mass can gather in one place to form stars. The earliest galaxies appear a few hundred million years after the Big Bang, and the star-formation rate rises as:
- more and more matter infalls onto the overdense regions,
- star clusters and then proto-galaxies merge together, leading to larger collections of mass,
- and the earliest generations of stars inject heavy elements into the mix, allowing for faster cooling and a more rapid formation of new stars.
The universe continues to form stars at a faster and faster rate for the next two billion years or so, but then it reaches a maximum and steadily falls thereafter.
By the time we reach the present day, we see that many of our modern-day galaxies are what we call “red and dead,” meaning that there have been no new major star-forming events in billions of years. Once that much time passes, all the hot, young, blue stars will have completed burning through their fuel, ending their lives and leaving only the cooler, redder, lower-mass stars behind. From both the stellar populations remaining in nearby galaxies and observations of ultra-distant galaxies from the early universe, we learn that fully half of the most massive galaxies that are present ~11 billion years ago have already stopped forming stars, permanently.
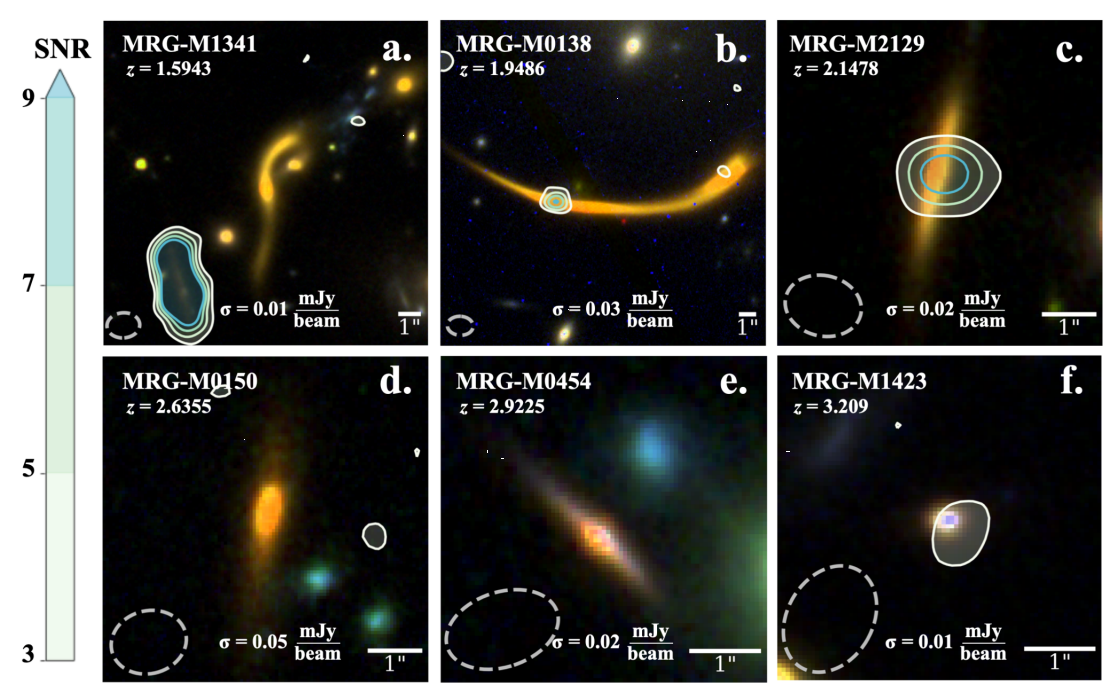
This, in and of itself, is a puzzle. In theory, at least in terms of what we would naively expect, there are three main reasons we would not expect star-formation to simply stop inside these massive galaxies, never to restart again.
- Even though a large amount of star-formation can energize, ionize, and expel neutral matter from these galaxies, we fully expect that the dark matter halos around them will keep that matter gravitationally bound, where it will eventually fall back onto the galaxy and form new stars.
- Over time, even in an expanding universe, material from the intergalactic medium will fall onto these galaxies and cause them to grow, eventually leading to a restarting of star-formation once a critical density is reached.
- These galaxies do not exist in isolation but rather in close proximity to one another. Processes like minor and major mergers should lead to subsequent bursts of star-formation over their history, leading to new populations of stars.
It is clear from what we have seen that, for a large fraction of these early, massive, star-rich galaxies, that does not occur. Of course, the burning question on the minds of astronomers is: Why?
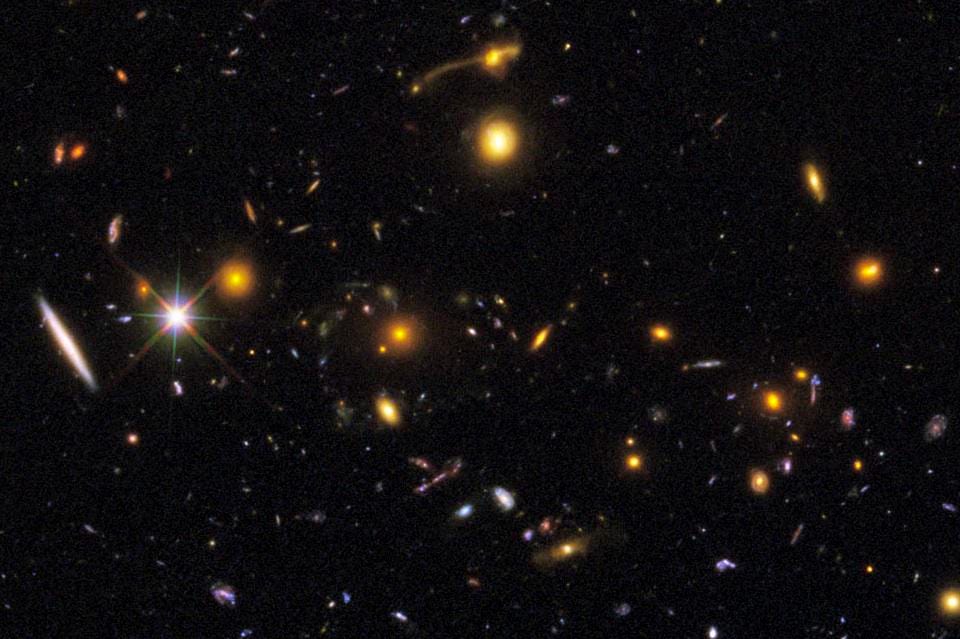
Ideally, what we would be able to do is use our most powerful observatories to look back at these very early galaxies right as star-formation reaches its peak and ceases. We would get to measure what was happening in terms of the stellar populations that were formed; the winds that arose from these hot, young stars as well as from the galaxy’s central black hole; and the surrounding matter, in terms of velocity, ionization, and density. By making direct observations of these galaxies at the earliest times and in great detail, we could finally understand how and why they permanently become “red and dead.”
Unfortunately, the current generation of observatories are not quite up to the challenge, as they are unable to measure those distant galaxies with the necessary resolution and light-gathering power to determine what is going on inside of them. But there is a way these observations suddenly become possible for a selection of these galaxies: through the enhancement effects of strong gravitational lensing. If there is a large enough collection of mass in between our line-of-sight and the background galaxy that we are attempting to observe, that light can be distorted, stretched out into multiple images, and — most importantly — magnified. With an ideal alignment, these background objects can appear tens or even hundreds of times brighter than they would in the absence of the intervening mass, which behaves as a gravitational lens.
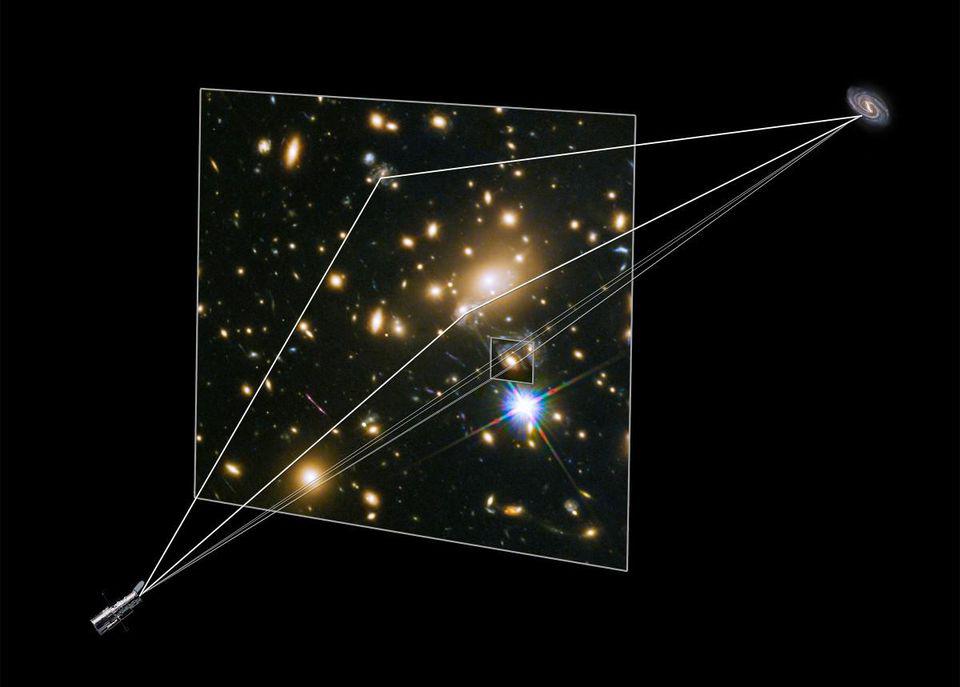
Credit: NASA & ESA
One of the most exciting developments in observational cosmology has been imaging campaigns dedicated to measuring, in multiple different wavelengths, the regions of space that ought to be gravitationally lensed by massive foreground clusters. Hubble’s Frontier Fields campaign was one of the earliest and most successful examples of this, but is being superseded in many ways by a more recent campaign: REQUIEM.
In particular, the REsolving QUIEscent Magnified (REQUIEM) galaxy survey, with joint observations by Hubble (in the optical and near-infrared) and ALMA (in the radio), allows us to first identify where massive, star-forming galaxies are at these early epochs, and then we can characterize them and their populations of gas. Previously, the only way to measure the presence of gas in galaxies this distant was by using a highly uncertain proxy: carbon monoxide tracing, and it often required the stacking together of data from different regions to extract some sort of average abundance.
However, with magnifications up to a factor of ~30 for a population of six galaxies in the REQUIEM galaxy survey, individual measurements of the presence (or absence) of dust in each individual galaxy at these great cosmic distances could finally be obtained.
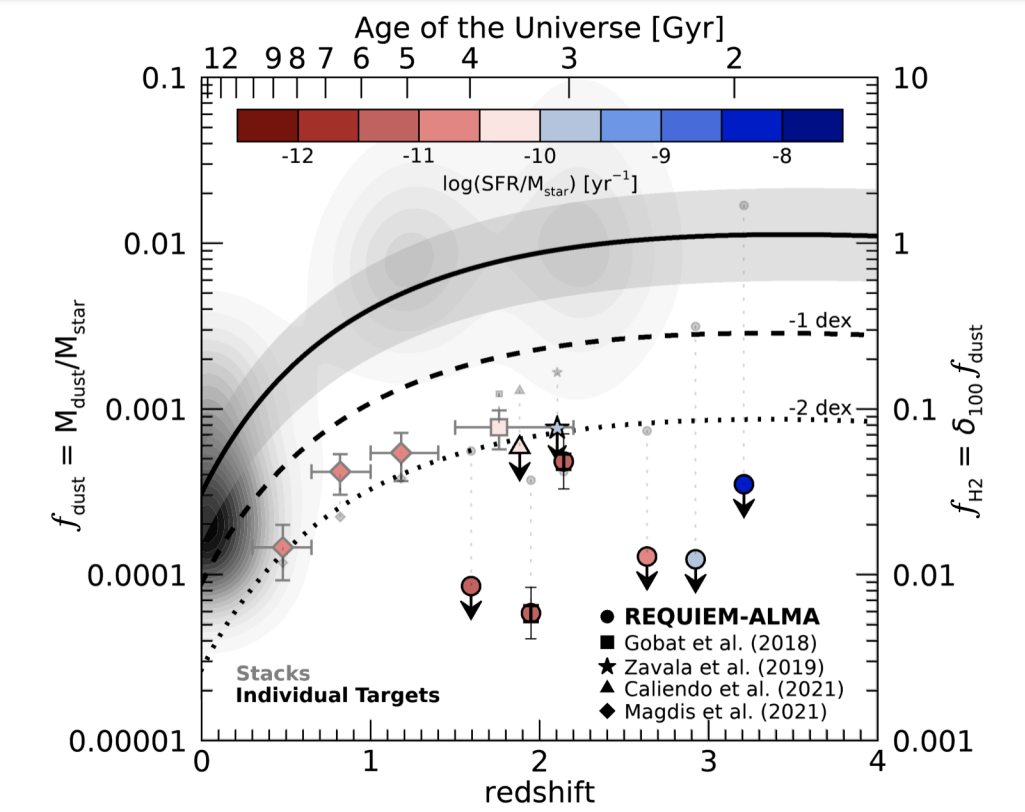
The combination of gravitational lensing and dust is an extraordinary one for a simple reason: Gravitational lenses magnify all wavelengths of light equally, since it is a purely gravitational effect, while dust emits light differently at different wavelengths, dependent on its temperature. The gravitational lensing effects allowed us to obtain these dust emission measurements for these distant, lensed galaxies, and astronomers then used a known and established procedure for translating those measurements into abundances of cold molecular gas: the material that forms new stars.
The researchers were then able to leverage their methodology to extract the amount of gas — specifically, of neutral molecular hydrogen gas, essential for the formation of new stars — that is present around these six galaxies, and they found something remarkable. One of these galaxies has about ~4.6 percent the gas of a normal galaxy at that distance, one has ~0.6 percent, and the other four have undetectably small amounts. Somehow, the gas and dust within these galaxies and in the surrounding vicinities have been almost entirely eliminated. Additionally, all six of these galaxies are hardly forming any new stars at all at present, with each one forming new stars at a rate lower even than the modern Milky Way, which forms less than one solar mass worth of new stars every year.
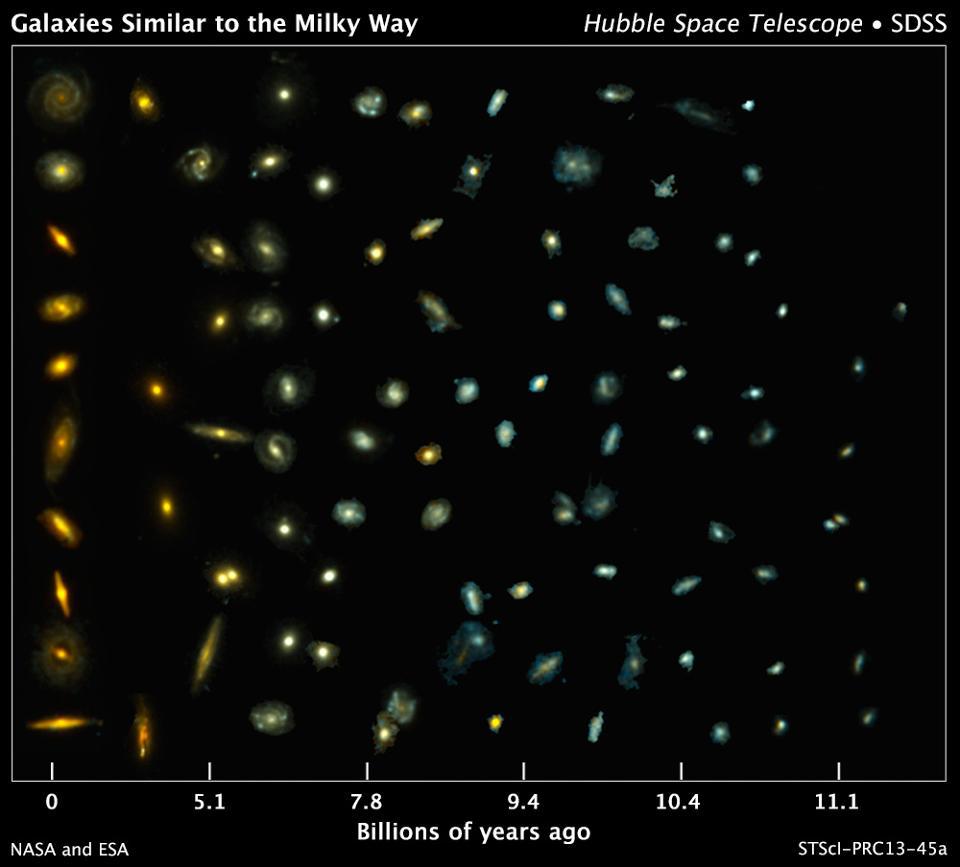
This discovery is remarkable for two reasons: (1) the impressive technical achievement that allowed it; and (2) a complete lack of cold, interstellar gas has never been observed this early in the universe. The star-formation rate and total gas presence in five of these six galaxies are below one percent of what is typical, and these galaxies are not rarities but rather are representative of approximately half of the most massive galaxies from when the universe was only about three billion years old. When we extrapolate this study’s implications to the full population of massive galaxies that existed at these early time, we learn that fully half of them have already ceased forming stars by the time the universe is three billion years old — that is, approximately 11 billion years ago.
The implications are astounding. Previously, gas was assumed to be slowly consumed by the host galaxy over time, but that cannot be the case. These massive galaxies not only lose their gas reservoir, the material needed to form future generations of stars, but it appears that they never get it back again: not from gas re-falling onto them, not from future mergers or infall, nothing. As the researchers state in their article,
“Our new measurements confirm that the cold interstellar medium was already rapidly depleted at high redshift in at least some galaxies, not slowly consumed until the present day.”
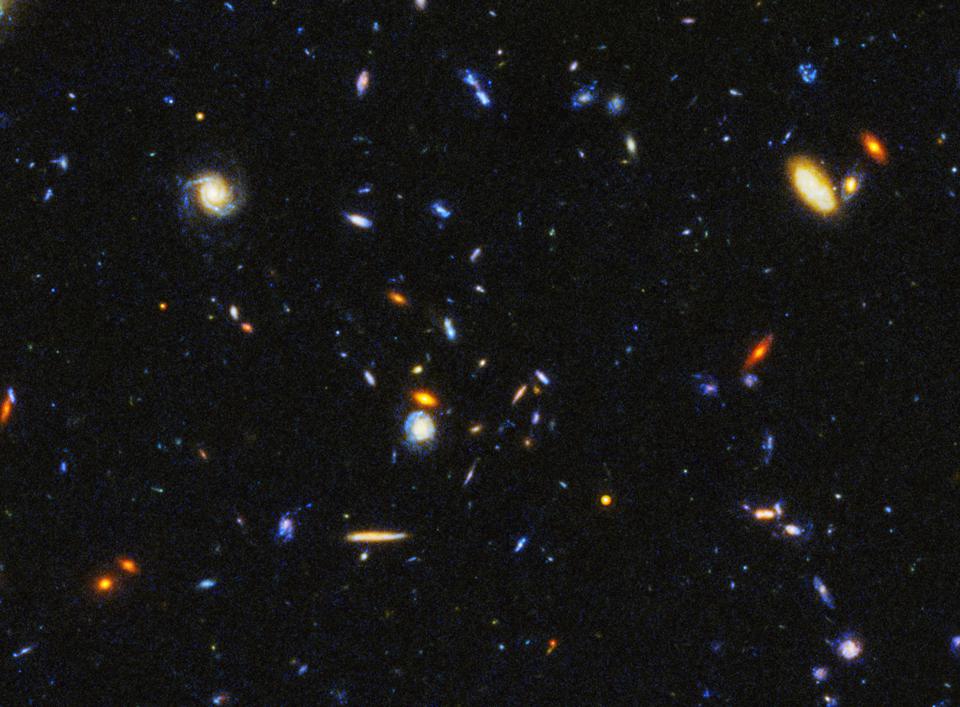
Last year, it was announced that scientists had found a new record for the earliest galaxy that had completely stopped forming stars: just 1.5 billion years after the Big Bang, or an estimated 12.3 billion years ago. In combination with previous studies, this result demonstrates that, at least for a large fraction of massive galaxies that existed in the early universe, many of them:
- initially form stars rapidly,
- then stop forming them early on,
- and then never turn on again.
This is well-supported observationally now, but theoretical models struggle to explain it and reproduce the observed populations of galaxies.
The evidence, as it is coming in, doesn’t seem to point to the expected scenario of star-formation occurring in multiple bursts and eventually turning back on for nearly all of these galaxies in at least some capacity. Instead, it appears that galaxies really do, in great numbers, “burn through” all of their potentially star-forming material, become gas depleted very early on, and remain that way — as far as we can tell — for the remainder of cosmic history.
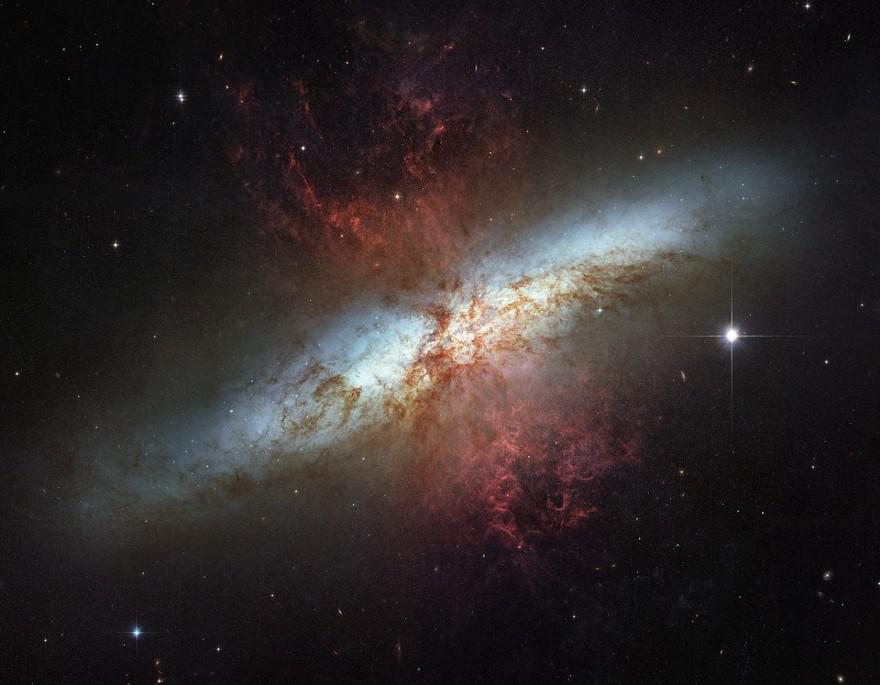
What is the key, then, to the cessation of star-formation in these early galaxies? It is that their gas reservoirs become depleted and do not get replenished. Previously, it was thought that some sort of inefficiency might arise after long periods of star-formation that simply caused it to stop, like a feedback effect or some type of back-reaction. Those ideas may play a role in some galaxies, but they cannot explain what we observe. The gas reservoirs clearly drive star-formation in these galaxies, and star-formation ceases because these reservoirs are somehow drained away and do not get replenished.
The next question, of course, is why does this happen? Is it because there are stellar winds from the massive, hot, blue stars that blow this neutral matter away? Could the feedback from active supermassive black holes at the centers of galaxies be responsible? Could there even be effects happening in the halos of these galaxies, or could mergers (which, themselves, would have to resolve relatively rapidly) be what is ejecting this gas and putting an end, permanently, to star formation?
All of these are still open questions to be decided with future observations and superior measurements. We have reached the stage where we are now accurately describing the origin and properties of “red and dead” galaxies; now, we need to figure out: how did they get to be this way, and how come they remain this way?
In the nearby, late-time universe, it is a combination of winds from the galactic center and a low-level of activity from supermassive black holes that keeps quiet, non-star-forming galaxies free of gas. The fact that half of all massive galaxies stopped forming new stars by the time the universe was only three billion years old, and that most of these galaxies never have another major episode of star formation, strongly suggests that perhaps this process started occurring more than 10 billion years ago and persists to the present day. The lack of dust — and, by inference, the lack of neutral, potentially star-forming gas — in even these ancient galaxies shows that something is not only depleting the gas at these very early times but is able to maintain their gas-depleted state indefinitely.
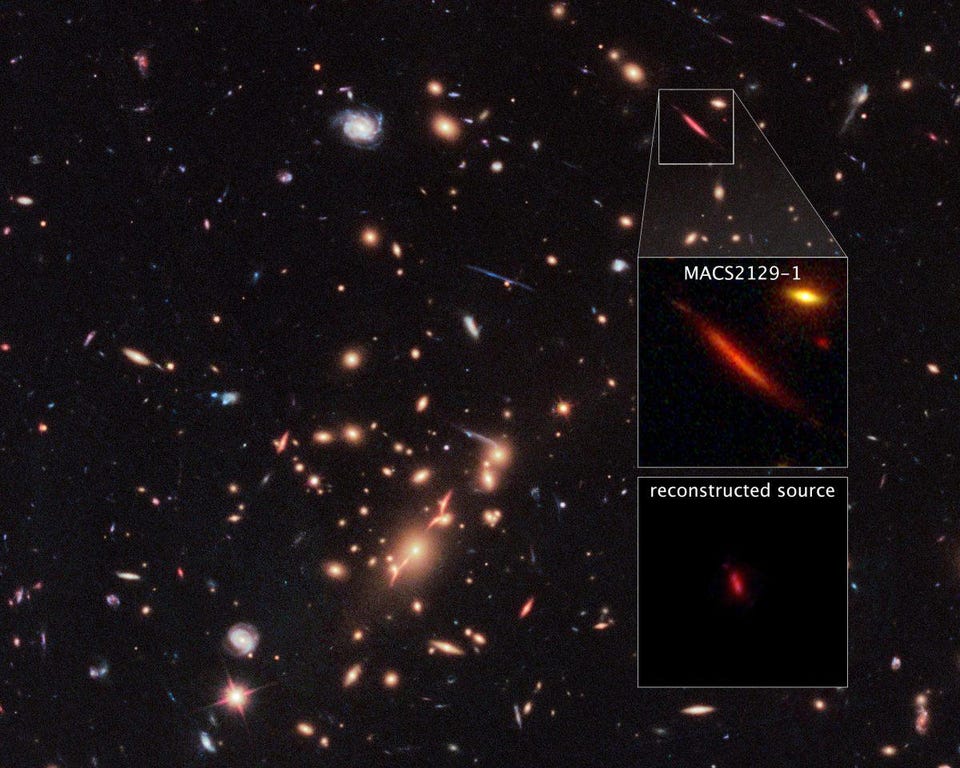
(Credit: NASA, ESA, and S. Toft (University of Copenhagen); Acknowledgment: NASA, ESA, M. Postman (STScI), and the CLASH Team)
Sure, mergers may occur, but when they do, they will lead only to a “frosting” of new stars, leaving the underlying stellar population largely unchanged. It is very rare to get a full, major episode of new star-formation in a red, dead galaxy, and these observations are the first to show us why: the gas is, and likely remains, very depleted. However, the jury is still out on the mechanism by which the gas stays away, and why these galaxies remain red and dead despite minor mergers and continued accretion.
Future generations of observatories undoubtedly will allow us to probe more of these early galaxies and their environments, as well as test our assumptions about the relationship between the presence or absence of gas and dust together. At present, the combination of gravitational lensing with multi-wavelength deep observations have just shown us that a great galactic genocide occurred very early on in the universe’s history — and that places us one step closer to figuring out the mystery of how the universe not only grows up, but how some of its properties stop growing entirely and simply die away.