Ask Ethan: Why is there something instead of nothing?
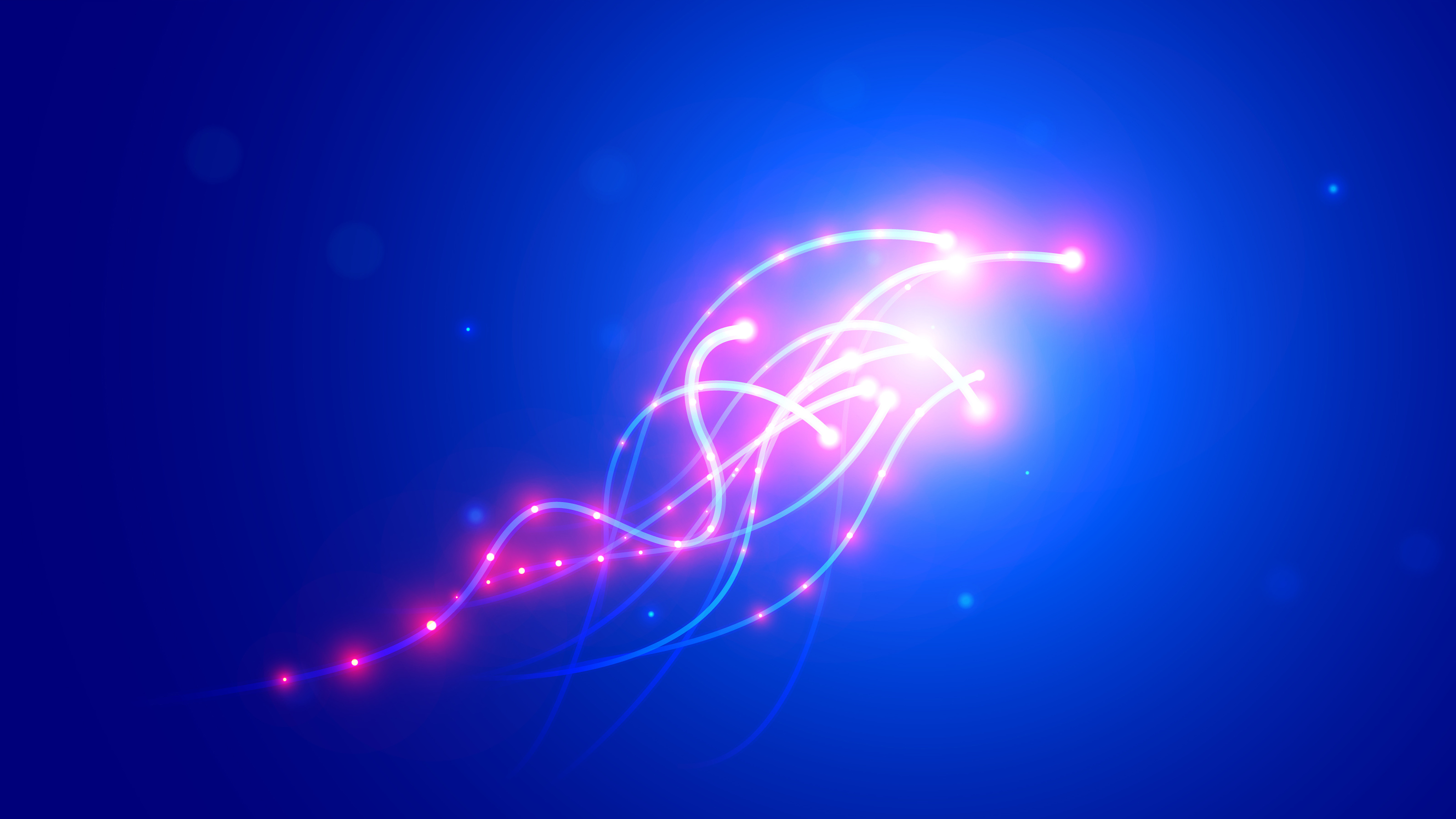
- It's a question that almost everyone has wondered at some point: given all the things that exist around us, in this world and in the great Universe beyond, what's the reason for why it all exists?
- We can trace the cosmic story of existence back in many ways: through time, to the Big Bang and even before, and to the processes that led to things becoming the way they are.
- And yet, there's always this nagging unanswerable question underlying each step we can answer: why is it this specific way, instead of all the other ways we could've imagined it? Here's as far as science has taken us.
Perhaps the most remarkable fact about the Universe, if you think about it on a truly fundamental level, is that it exists at all. And yet, not only does it exist, but there’s matter within it, which obeys the same rules everywhere and at all times, and assembles according to the physical laws governing reality to create, among other things:
- atomic nuclei,
- neutral atoms,
- molecules,
- stars and planets,
- galaxies,
- and a large-scale cosmic web.
Not only that, but in at least one relatively unremarkable corner of this Universe, a planet arose some 4.5 billion years ago where life survived and thrived, eventually giving rise to an intelligent, self-aware species that can ask deep questions about the Universe they inhabit.
In doing so, we’re also asking fundamental, deep questions about our own selves, as we’re just as much a part of this Universe as any inanimate objects that exist. That’s what led Steve Cordon to write in and ask one of the deepest questions of all:
“I’ve always wondered why is there something instead of nothing? I’ve read some books authored by a variety of cosmologists. I was curious as to your explanation on that as well. I look forward to your plausible explanation! As Carl Sagan was known to say, ‘Extraordinary claims require extraordinary evidence!'”
This is one of those questions, I’m sorry to say, that science not only doesn’t have a satisfactory answer for right now, but will probably never have one. Although there’s a lot we can say about this question, we face fundamental limitations by the very nature of the scientific enterprise. Here’s why.

How did we get to be here?
There’s an enormous difference between a “why” question, which science isn’t really well-equipped to answer, with a “how” question, which is the bread-and-butter of what science is good for. If we were to ask the question of why we’re all here, there isn’t a scientific way to approach this question: we can’t formulate a testable hypothesis and derive what sorts of things we can go out and measure to answer that. Even if we determine the underlying rules that reality follows, there’s a limit to what we can derive from them: we can derive physical consequences that stem from those rules and some set of initial conditions, but we cannot derive any sort of purpose behind those consequences using the tools of science.
The question of “how” we got to be here, on the other hand, is one that science can tell us literally everything about. Especially if we’ve already done the hard work to understand the laws of science, from the biological and geological and atmospheric evolution that’s occurred here on Earth to the chemical evolution that’s occurred through the differing environments of space and on Earth to the particle-based evolution that occurred inside stars and in the crucible of the Big Bang, we can put those pieces of the story together to understand precisely how this Universe gave rise to our Solar System, our planet, the origin of life, and after some 4-ish billion years, even a full-fledged human being.
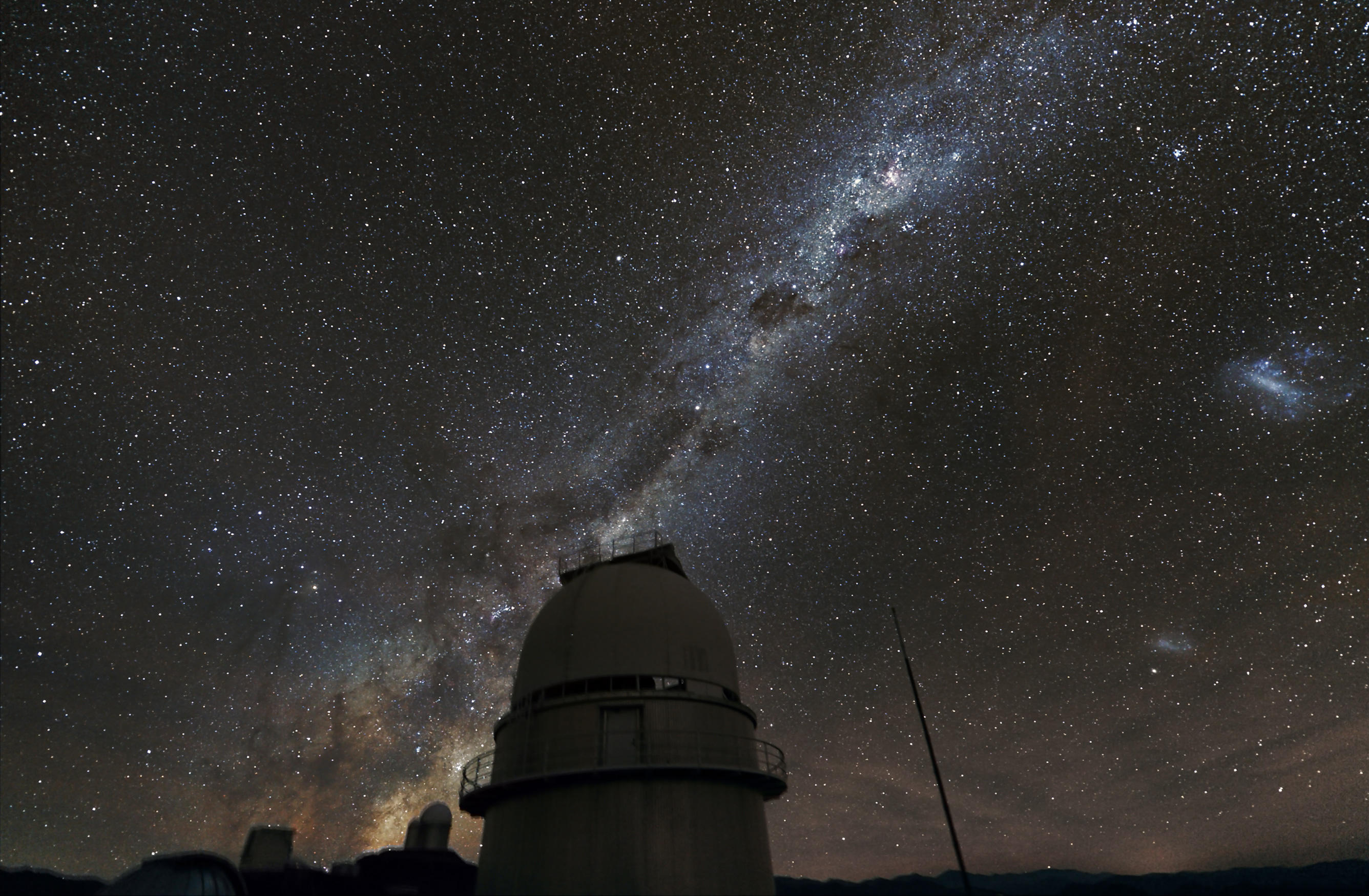
Although that story — of how we came to be the way we are — still has a few gaps in it as far as definitive knowledge is concerned, these are gaps that are forever shrinking as we accumulate more and more knowledge about:
- the laws and rules that govern the Universe,
- the past and present phenomena that have occurred here on Earth,
- and the great variety of possible outcomes, given similar starting conditions, that occur or could have occurred elsewhere in the cosmos.
We still don’t know how, specifically, life originated from non-life, how many planets once existed and were either destroyed or ejected at some point in our Solar System’s past, or what predecessor stars existed that enabled the Sun to form with the properties it possessed at its birth.
And if we step back and take a look at the bigger cosmic picture, it turns out that although there are some really fascinating details that we do profoundly understand, there remain gaps there, too: things that we know must have happened in our cosmic past at some point and in some way, but whose details — the specifics of “how” they occurred — remain obscure to us. Some of these cosmic unknowns are among the biggest questions in theoretical physics and astrophysics today.

The knowns
This isn’t to undersell what we actually do know, which is perhaps the greatest achievement of the entire enterprise of human civilization. The fact that we have asked the Universe so many questions about itself and how it works have revealed an incredible amount of information.
Biologically, we understand the inheritance of traits and the role that mutation and selection plays, leading to what we commonly know as Darwinian evolution. We understand that Darwinian evolution is not a fundamental law, but rather that inheritance is driven by genetics, which was further developed by Gregor Mendel. And Mendelian genetics, while profoundly important, itself is a consequence of the genetic code written down in every plant, animal, and fungus’s DNA, discovered by James Watson, Francis Crick, and Rosalind Franklin.
But DNA is simply a molecule: assembled and synthesized together out of atoms through a series of chemical (and biochemical) reactions. And chemistry is just a consequence of atoms and molecules (and ions) reacting together in an environment with a temperature or energy gradient: a complex process, sure, but one whose interactions and reactions can be understood at a fundamental level.

We can go back further still: to the origin of the atoms that make up every molecule. Within the heart of every star, light elements are fused into heavier ones through incredibly energetic nuclear reactions. In a star like the Sun, hydrogen is fused in a chain reaction to build up helium, while carbon, nitrogen, and oxygen also play an important (but sub-dominant) role. In more evolved stars, helium is fused into carbon, and in very massive stars, elements like neon, oxygen, magnesium, silicon, sulfur, argon, and calcium are also produced in chain reactions, eventually leading up to the formation of the most stable elements of all: iron, cobalt, and nickel.
When the most massive stars die, they do so in catastrophic core-collapse supernova explosions, typically creating elements in abundance all the way up the periodic table to zirconium (element #40), but few beyond that. (Sun-like stars, in their final, giant phase, process pre-existing nuclei to create a smattering of elements from strontium, element 38, up through bismuth, element 83.)
When stars generally die, the remnants they leave behind include white dwarfs, neutron stars, and black holes. When white dwarfs and white dwarfs merge, they create a different kind of supernova (type Ia) that also produces a wide variety of elements. When neutron stars merge with neutron stars, they can produce a cataclysm known as a kilonova, where the majority of still-heavier elements are produced. All told, we can trace back the astrophysical origin of every element found in the periodic table using modern science.
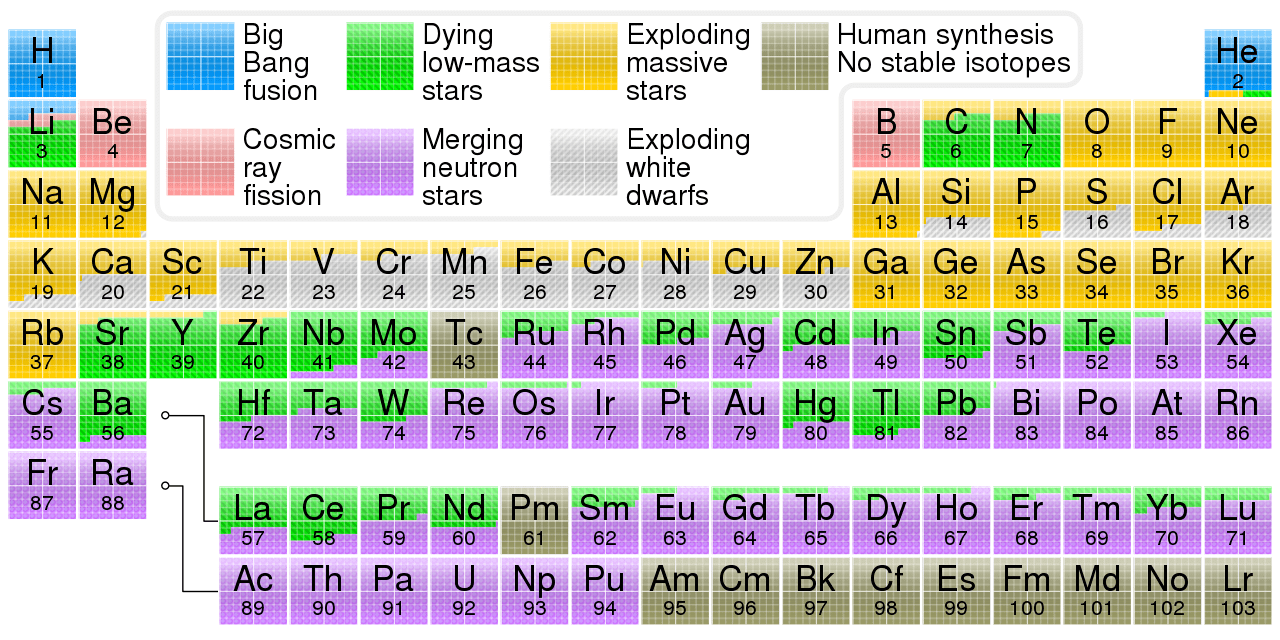
Going back to the beginning
In fact, this is one of the most important properties of science overall: the notion that, if you understand a physical system well enough, then simply by providing
- a set of initial conditions for that system
- plus the physical laws that govern it,
then we’ll be able to calculate that system’s properties, or at worst, a probabilistic set of outcomes for that system’s properties, at any arbitrary moment as far into the future as we like. This, in many ways, is the heart of what science is: our best and most accurate models of reality, and an attempt to account for all observable and measurable phenomena in the process.
We can use all we’ve learned to extrapolate backward in time, and learn what the Universe was like at earlier and earlier times. We can go back to a time before any stars had formed, and by applying the laws of physics, learn how stars, galaxies, and the cosmic web assembled. Moreover, we can learn what “seeds” of structure — or initial conditions — needed to be in place to give rise to what we observe at later times. We can go back still farther and learn about the formation of neutral atoms (and the prediction of the cosmic microwave background), the formation of atomic nuclei (and the prediction for the abundance of the light elements), the creation of a primordial neutrino background (and predict the observable signatures it leaves today), and back all the way to the Big Bang and even before, to the phase of cosmic inflation that preceded it and set it up.

The unknowns
But that still doesn’t tell us everything. For example, we know that, after the primordial soup from the hot Big Bang cooled off, we were left with a slight overabundance of matter compared to antimatter. It’s as though, during the earliest phases, for every 500 million antiquarks that were present, there were 500 million and one quarks that were present. For every 1.5 billion positrons that were present, there were 1.5 billion and one electrons that were present. And while we know some general rules that allow us to create a matter-antimatter asymmetry from an initially symmetric state, we have yet to unpack precisely how our Universe generated the matter-antimatter asymmetry that gave rise to our existence.
We don’t know what many of the specifics of cosmic inflation were, or how, precisely, it came to an end to create the hot Big Bang. We don’t know how “hot” it actually got during the hot Big Bang; we have an upper limit and a lower limit on the initial temperature, but there are some ~14 orders of magnitude in between those limits. We don’t know how the fundamental constants came to possess the values that they have, and how we came to have the four fundamental forces (and, seemingly, no others) within our Universe. And from a phenomenological point of view, we have an overwhelming amount of evidence for the existence of both dark matter and dark energy, but precious little evidence indicating what their true nature actually is.
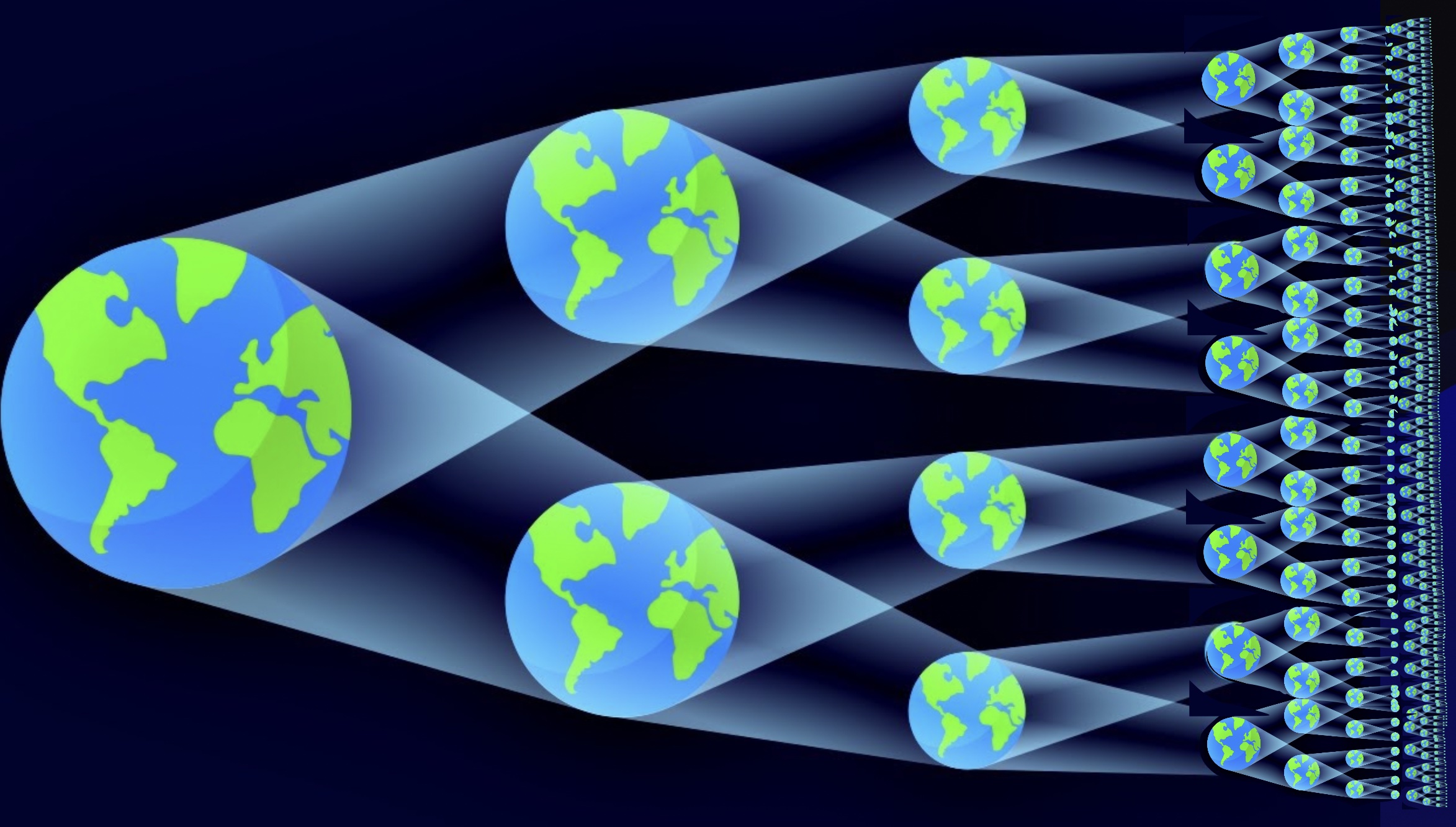
The bigger questions
It leads to a question that almost everyone — physicists and non-physicists alike — has asked themselves at some point or other: did things have to turn out this way, or could they have been different? If you were to run the clock all the way back to the earliest state we can say something physically sensible about, the inflationary state with the same initial conditions ours possessed, would you get the same Universe with the same:
- fundamental constants,
- laws of physics,
- hot Big Bang,
- sets of species of particles and antiparticles,
- matter/antimatter asymmetry,
- and dark matter and dark energy,
that our Universe possesses? Would you get the same structures, from atoms to stars to the cosmic web? The same types of planets? The same chemical and biological outcomes?
On some of these accounts, we’re pretty certain the answer is no. There are too many possible outcomes and too many interacting particles for us to expect that, for instance, human beings would exist anywhere else in the Universe, or would come to exist in a Universe that started off with identical initial conditions to our own. A great many properties that our Universe possesses, from the cosmic microwave background to the cosmic web, would certainly be similar in a statistical sense to the ones our actual Universe possesses, but we’re certain they wouldn’t be identical. The laws of physics, after all, are known to not be fully deterministic in an inherently quantum Universe.

But what about the fundamental constants? What of the laws of physics themselves? What about the number of forces, the types of particles and antiparticles that are allowed to exist, and the properties and existence of poorly-understood entities like dark matter and dark energy?
The truth is: we don’t know. We can postulate physical and mathematical extensions to what’s known to try to answer them, and under many of those extensions, there are indeed variations that are allowed. Those possibilities include:
- extra dimensions,
- grand unified theories,
- string theory (and other ideas concerning quantum gravity),
- theories of everything (of which string theory is one),
all of which allow for the existence of new particles, new interactions, and new phenomena, such as leptoquarks, superheavy bosons, and proton decay.
But more conservative scenarios, still fully consistent with everything we observe, don’t include these new phenomena, and don’t necessarily allow different sets of fundamental constants, new laws of physics, or novel forces and interactions. These are questions — big, profound questions — that science has yet to figure out.
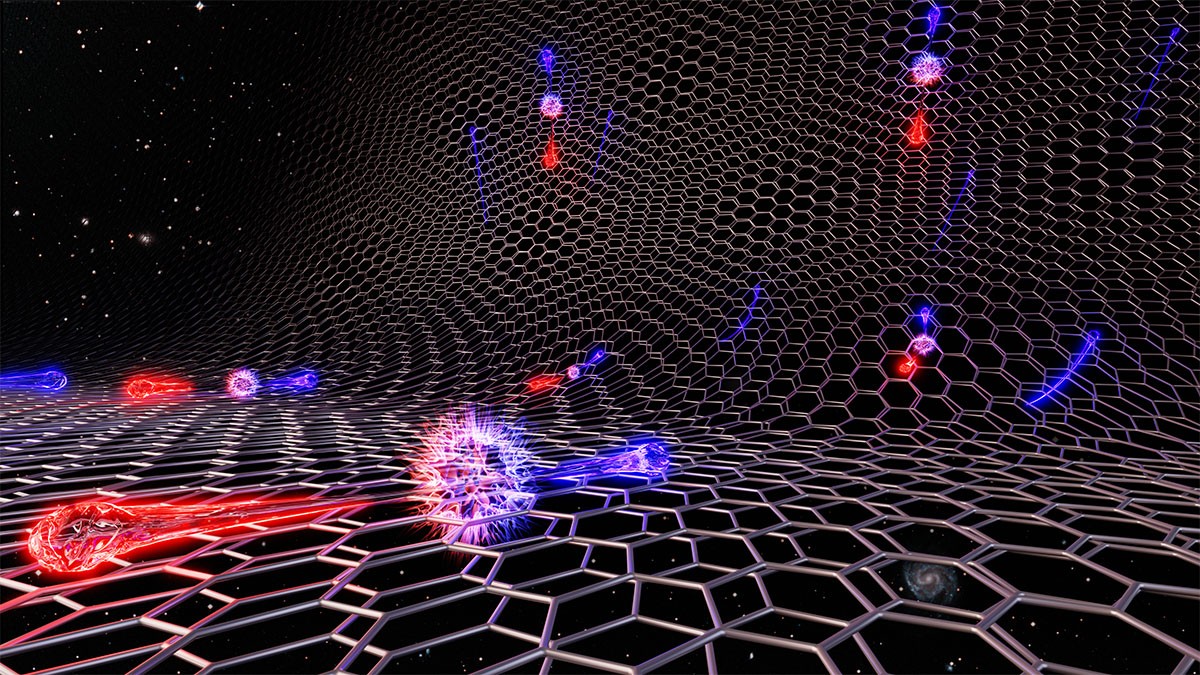
So why is there something instead of nothing?
We are certain that “something” exists. We are certain that if you take away the particles and antiparticles and photons and quanta in a region of space, that empty space will still exist. If you move far away from any sources of mass or energy and clear the space of all external electric, magnetic, and gravitational fields, and prevent any photons or gravitational waves from entering that space, that “physical nothingness” will still exist in that region. And in that region, certain things cannot be removed:
- there will still be quantum fields in the vacuum of that empty space,
- the fundamental constants and underlying laws of physics will still exist in that empty space,
- and there will still be a “zero-point energy” inherent to that space, and it will still possess a finite, positive, and non-zero value.
As far as we can tell, that’s as close to nothing as we can get within our Universe.
You might be able to imagine, in your mind, a state of pure nothingness that’s even more “nothing-like” than this, but that doesn’t represent anything physically real. There’s no experiment you can design that can create such a condition. The best we can say — assuming that we’re sticking to science and not moving into the realm of theology, philosophy, or pure imagination — is that the reason there’s something rather than nothing is that “nothing” cannot exist compatibly within our Universe. Of course, that leads back to the original question: why? And for that, dissatisfying though it is, science has no answer. The Universe is the way it is, and though we strive to understand it as best we can, we are compelled to be humble before the great cosmic unknown. The only advice I can give you is this: beware of anyone who claims to “know” the unknown. They may or may not be fooling themselves, but you certainly shouldn’t allow them to fool you.
Send in your Ask Ethan questions to startswithabang at gmail dot com!