71 years earlier, this scientist beat Einstein to relativity
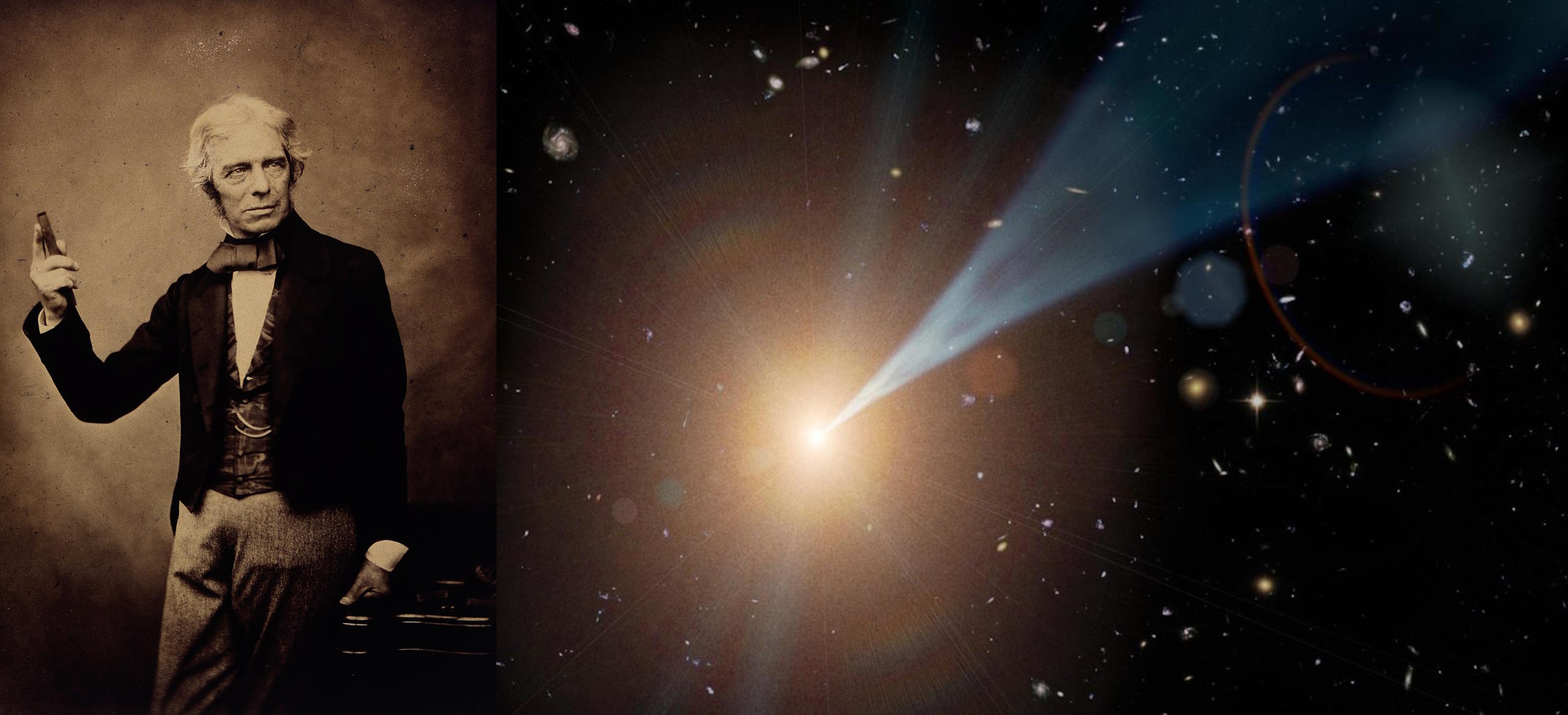
- The central pillar of relativity is that the speed of light is the same for all observers everywhere in the Universe, regardless of where they are or how fast they're moving.
- This principle of relativity was set forth by Einstein in 1905, but the groundwork was laid 71 years before by a much less well-appreciated scientist: Michael Faraday.
- Nearly a century later, Einstein himself credited Faraday's experimental demonstration of "Faraday's law of induction" as the key breakthrough behind relativity. It's hard to disagree.
The year 1905 was known, in scientific circles, as Einstein’s “miracle year.” All in that same year, he published papers on Brownian motion, the photoelectric effect, his famed equation E = mc², and perhaps most notably, special relativity. But special relativity is one of those discoveries that seemingly came out of nowhere. Whereas everyone in physics was concerned about many of the apparent contradictions of Newtonian mechanics with a variety of recent experimental results, Einstein’s solution — that the speed of light was constant for all observers in all frames of reference — was truly revolutionary.
But how did he come upon that idea?
- Did it spring from the aftermath of the Michelson-Morley experiment, which failed to detect any motion of Earth through the alleged aether?
- Was it inspired by the work of Lorentz and Fitzgerald, who showed that lengths contract and time dilates near the speed of light?
- Or was it derived from the work of Maxwell, who unified electricity with magnetism and showed that electromagnetic waves propagated at the speed of light?
According to Einstein himself, it wasn’t any of these. Instead, it was an experiment performed by Michael Faraday back in 1831, demonstrating Faraday’s law of induction, that Einstein credits with being the key realization at the root of relativity.
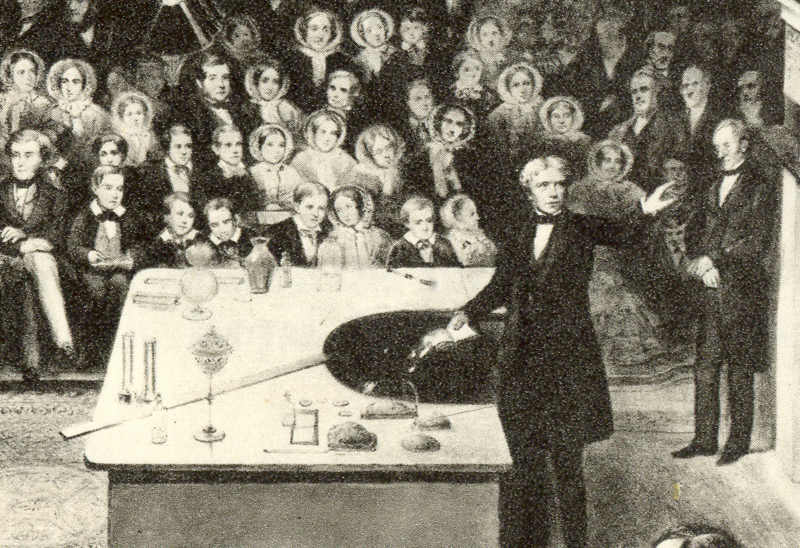
There have been many brilliant minds throughout history that were incredibly important to the development of science, but whose brilliance is underappreciated today. While Einstein, Newton, and Maxwell (in some order) are generally regarded as the three greatest physicists in history, their fame primarily arises because of the theoretical developments they undertook.
But arguably, even more important to the endeavor of understanding our physical reality are experiments. Choosing the right experiment to perform is an art all unto itself: experiments, after all, are our way of asking nature the key question of, “How do you work?” If we perform the right experiment, those experimental results will provide an information-rich, and potentially revolutionary, set of answers.
Today, many people look back on Michael Faraday — one of the greatest minds of the 19th century — with a lack of appreciation. Some dismiss him as a mere tinkerer for the most unceremonious of reasons: because his great successes were not based in equations or explicitly quantitative prediction. However, his intuition for setting up experiments in ingenious fashions led us to many of the greatest truths of nature that are fundamental to our picture of physical reality today.
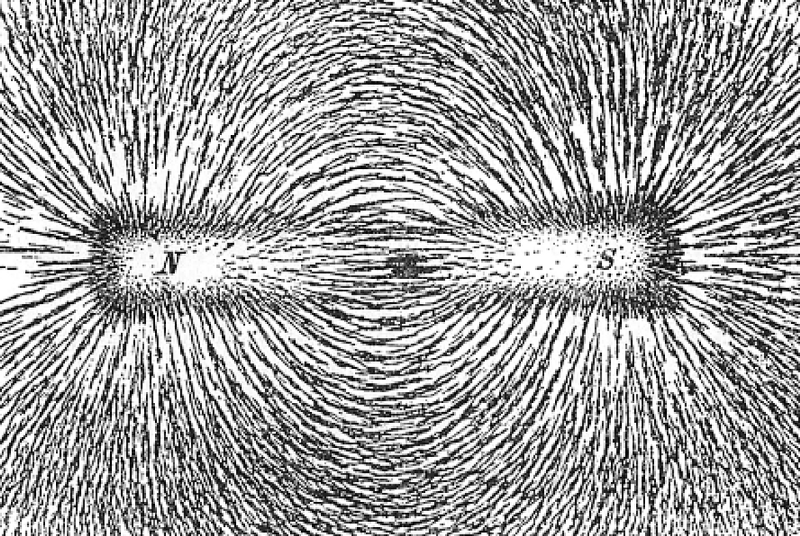
At a time when electricity was first being harnessed and its applications were still in their infancy, Faraday was revealing deep truths about the interconnected nature of electricity with magnetism. As difficult as it is to imagine, electricity and magnetism were originally — and for a very long time thereafter — treated as completely separate, independent phenomena.
- Electricity was based on the notion of charged particles that could either be stationary (where they would attract or repel) or in motion (where they would create electric currents), with static electricity being an example of the former and lightning being an example of the latter.
- Magnetism was treated as a permanent phenomenon, where certain minerals or metals could be permanently magnetized, and the Earth itself was viewed as a permanent magnet as well, allowing us to orient ourselves relative to our magnetic poles via the use of a magnetized compass.
It was only in the early 19th century, with the 1820 performance of the famed Oersted experiment, that we began to understand that these two phenomena were linked together.
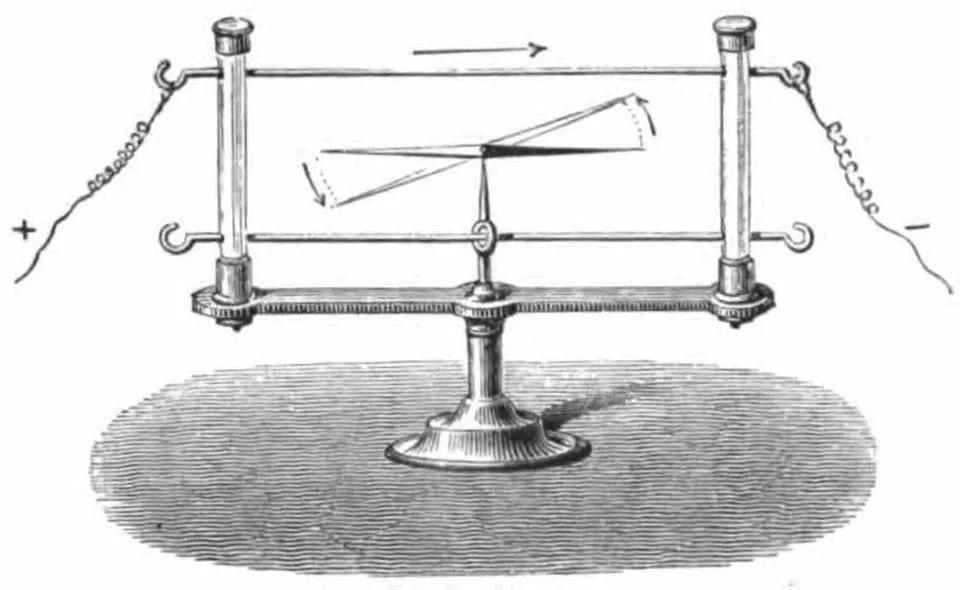
Imagine that you had a wire that carried an electric current through it: something we were just learning how to do in the early 1800s with the invention of the first voltage sources. Now imagine placing a compass needle — a permanently magnetized piece of metal — alongside that wire. What do you expect will happen?
What you’ll find is that the compass needle always deflects to align perpendicular to the current-carrying wire.
This was so poorly anticipated that the first time the experiment was performed, the needle was set up perpendicular to the wire initially, and no effect was observed. The expectation was that if the needle was going to respond at all, it ought to align with the electric current, rather than perpendicular to it.
It’s a very good thing for the development of science, in general, that tinkerers exist, because they were the ones who thought to do the experiment beginning with the needle already aligned with the wire. In doing so, they were able to observe the first link between electricity and magnetism: an initially-aligned magnet will deflect to instead align perpendicular to a current-carrying wire. The result of that experiment demonstrated something revolutionary: an electric current, or moving electric charges, generated a magnetic field. The next step, taken by Faraday, would prove to be even more revolutionary.
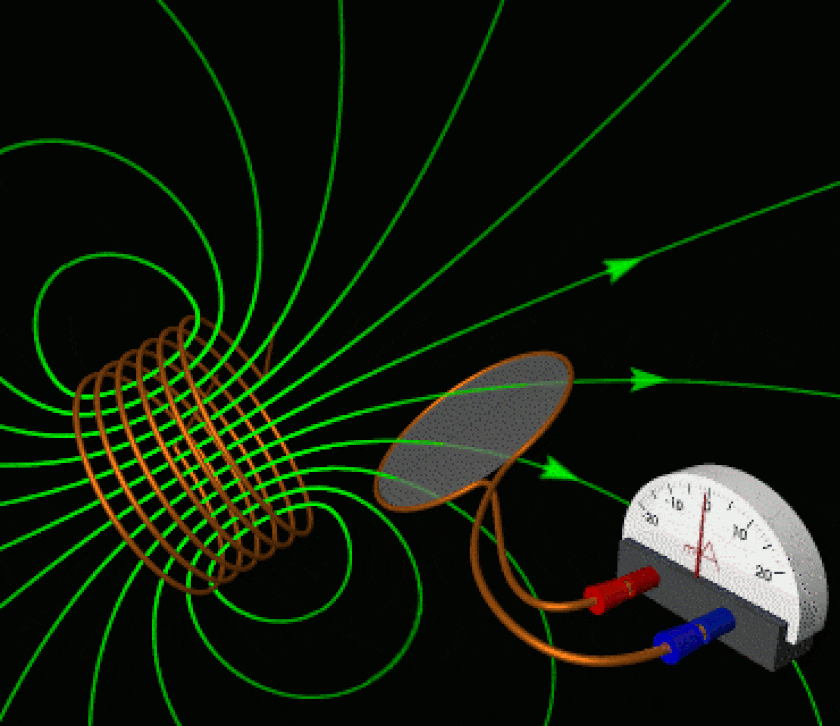
Most of us have heard of Newton’s third law of motion, which states that for every action, an equal and opposite reaction takes place. Whenever you push against an object with a certain amount of force, that object pushes back against you with an equal and opposite force. When the Earth pulls you down with its gravitational force, you pull back on Earth with an equal and opposite gravitational force.
But there are more examples of “actions” and “reactions” than just mechanical and gravitational forces.
Consider the following. We just saw, from the Oersted experiment, that a moving electric charge inside a wire (i.e., an electric current) is capable of generating a magnetic field. What would the equal-and-opposite setup of that scenario be? Perhaps, if one generated a magnetic field in just the right way, it could cause the generation of electric currents (i.e., the motion of electric charges) inside a properly-placed wire. Faraday, after tinkering with a variety of setups, finally found one that worked. He determined that if you changed the magnetic field inside a loop of wire by moving a permanent magnet into or out of it, that changing magnetic field would generate an electric current in the loop itself.
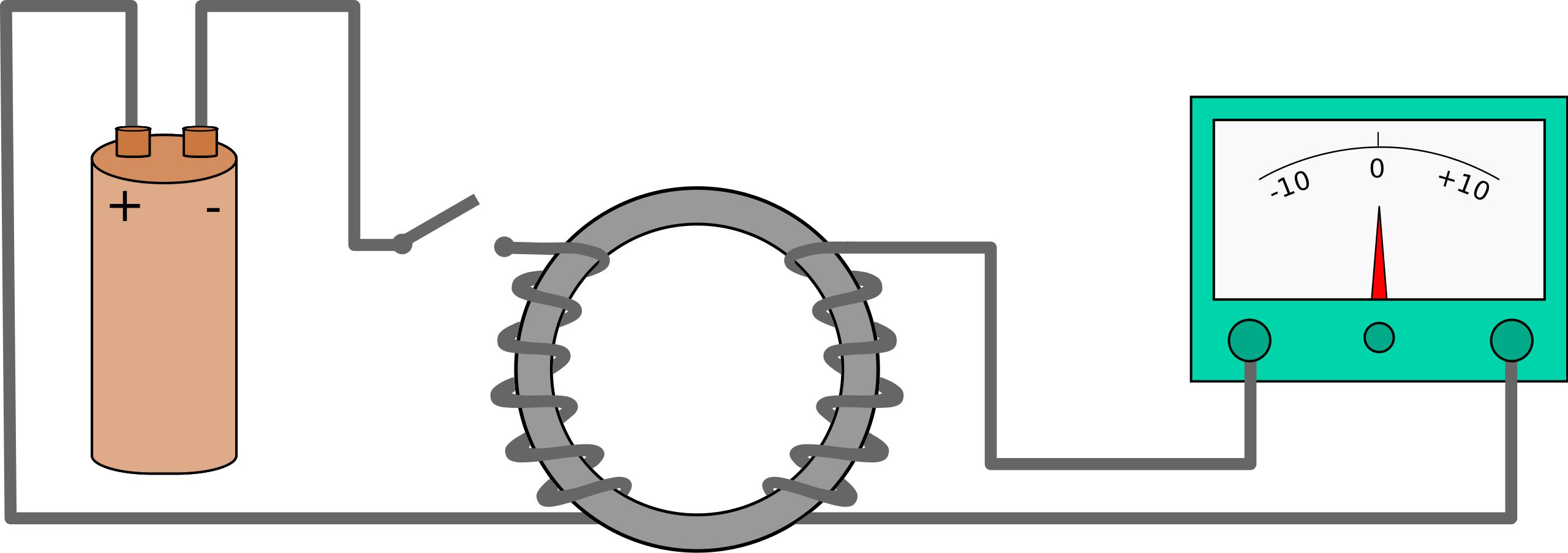
Faraday first made this discovery way back in 1831, and was determined to reveal more and more precise details about how this relationship, between magnetism and electricity, actually worked. After tinkering with a setup involving just a few ingredients — wires that could be bent into various shapes, batteries, magnets, and pieces of metal — he successfully showed which effects occurred under a variety of conditions.
- When you change the magnetic field inside a loop or coil of wire, you induce an electric current that opposes the change in the field.
- If you put an iron ring around two loops of wire and run an electric current through one loop, you generate a current in the other loop.
- If you rotate a copper (conducting) disk near a bar magnet with an electrical lead, you could generate a constant electric current; this was the invention of the first electric generator.
- And if you move a coil of current-carrying wire into or out of the interior of a coil of wire with no current through it, it will create an electric current in the larger coil.
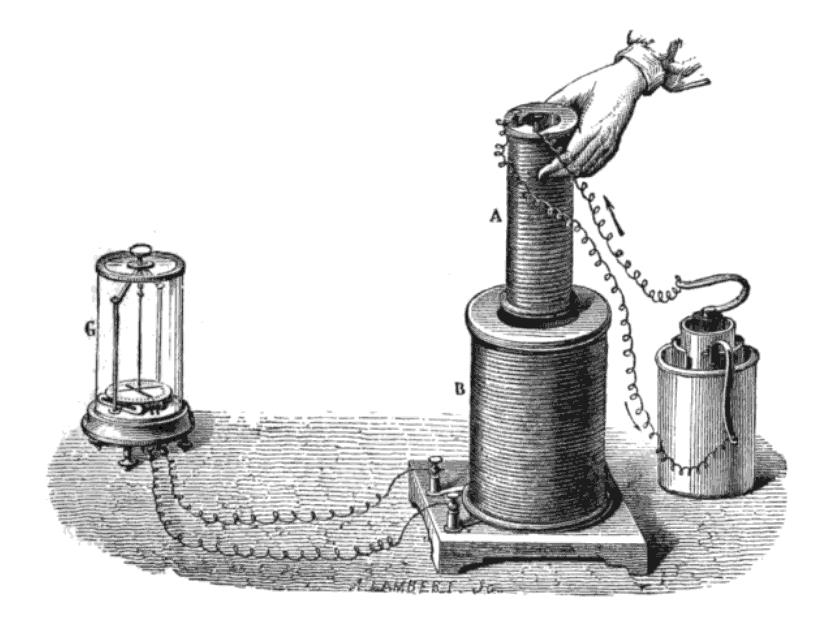
All of these phenomena could be encapsulated by a single physical rule, known today as Faraday’s law of induction. While most of his early experiments were performed in 1831 and 1832, the law of induction was put forth in essentially its modern form just a few years later: in 1834. And it was by thinking about this law of induction that Einstein first began to uncover what we know today as the principle of relativity.
Here’s how to picture it for yourself in almost the exact same fashion that Einstein did. Consider the following two setups, both involving a coil of wire and a permanently magnetized bar magnet.
- You have a fixed, stationary coil of wire, and a bar magnet that you can move into or out of the coil of wire. You move the magnet into the coil at a constant speed, and watch the electric current appear in the coil.
- You have a fixed, stationary bar magnet, and a coil of wire that you can freely move onto or off of the magnet. You move the coil onto the magnet at a constant speed, and watch the electric current appear in the coil.
If you think about these two scenarios without considering relativity, you would imagine that each of these two experiments would be governed by very different phenomena from one another.
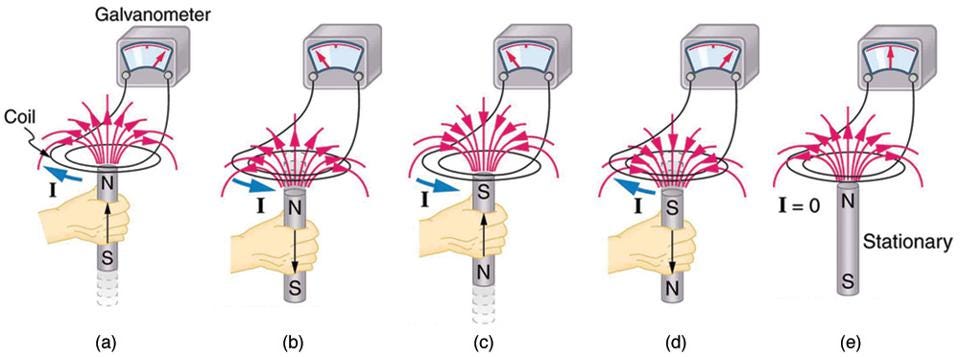
In the first scenario, you move the magnet into a stationary, conducting coil. As you move it, the magnet begins to see an electric field arise, and that field must contain a certain amount of energy, as all electric fields do. Because there’s an electric field, electric charges are now compelled to move, producing a current in the conductor that’s dependent on the energy of the electric field generated by the moving magnet. This scenario corresponds to the first setup, above.
In the second scenario, where you instead keep the magnet stationary and move the conducting coil down onto the magnet, there would now be no electric field arising around the magnet. What happens, instead, is that you get a voltage (or electromotive force) arising from within the conductor, which doesn’t have a corresponding energy inherent to it at all. This scenario corresponds to the second setup, above.
However, experimentally, both of these setups must be equivalent. In both scenarios, a magnet moves into a coil of wire at the same speed, where they produce the same electric currents of the same magnitude, intensity, and direction in the coils of wire. And it was this realization, more than any other, that led Einstein to the principle of relativity.
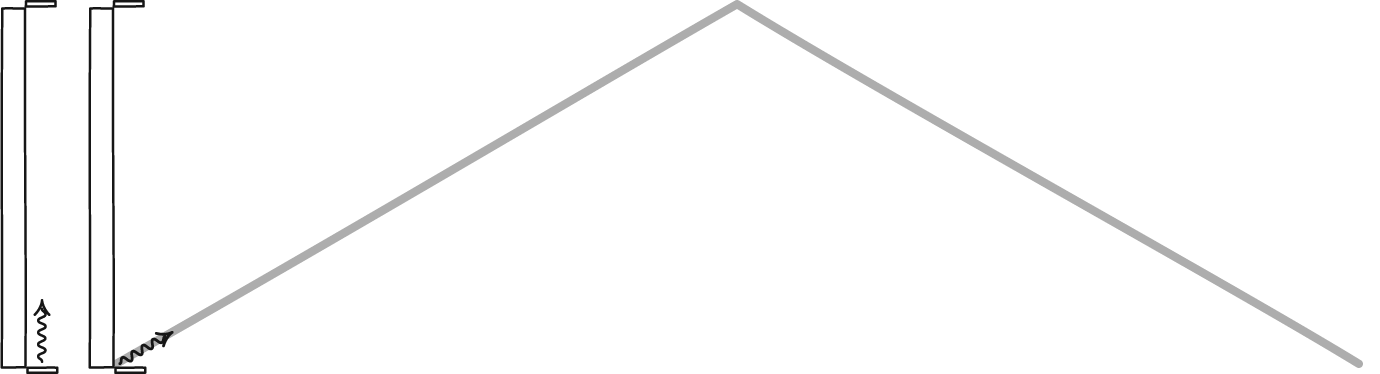
The principle recognizes, first and foremost, that there is no such thing as a state of absolute rest. If two things are in motion relative to one another, then it doesn’t matter if “thing 1” is moving and “thing 2” is stationary or vice versa; the physical reality that exists is independent of which point-of-view we adopt. The principle of relativity dictates that all observers, regardless of how fast or in what direction they’re moving, will see the same laws governing reality as one another. This applies to the laws of electricity and magnetism, the laws of mechanics and gravitation, and to any fundamental laws that remained to be discovered at that time.
When we talk about relativity today, we almost always discuss the Michelson-Morley experiment, which showed that the speed at which light travels doesn’t change regardless of whether you orient it with the Earth’s motion around the Sun (at ~30 km/s, or approximately 0.01% the speed of light) or perpendicular to that motion, or even at any arbitrary angle with respect to the Earth’s motion. The speed of light is observed to always be a constant, regardless of how we move.
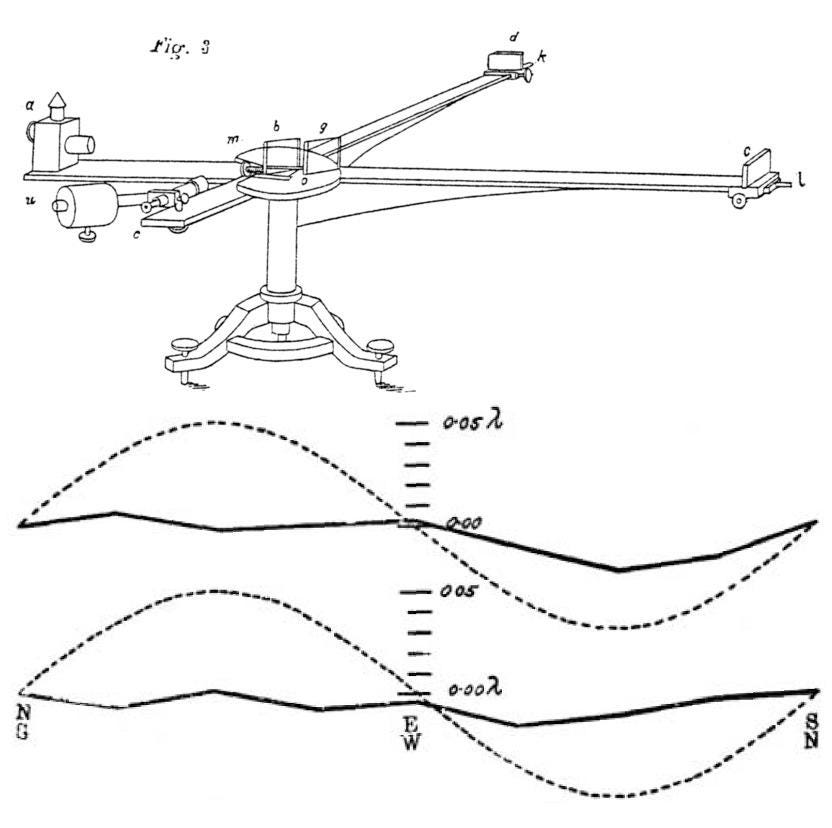
But it was the genius of Faraday’s law of induction, which demonstrated the experimental equivalence of two setups that seem so different on the surface, that first showed how unimportant the absolute motion of a system is in determining a physical outcome. Only relative motion within the system matters, not the point-of-view or frame-of-reference that you adopt. It was only a small step from Faraday’s remarkable work on induction in the 1830s to the Einsteinian revolution of 1905.
Perhaps Faraday himself even realized this, as many of his experiments demonstrate a deep understanding of the relative motion of charged particles and the universality of electricity and magnetism as a single, interrelated phenomenon. A few years before his death, he delivered a lecture before the Royal Society on “The Various Forces of Matter and their Relations to Each Other,” which contained a number of prescient philosophical moments that suggested, at the very least, that he had thought about the universality of physical laws in any frame of reference. Alas, Faraday died without ever publishing written material on the subject, so we’ll never know. But perhaps if Einstein can credit Faraday as his inspiration for the development of relativity, perhaps we all ought to remember his scientific legacy, too.