Science uncovers the origin of the first light in the Universe
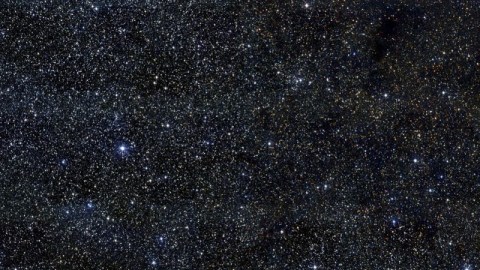
‘Let there be light’ isn’t just biblical. It’s science.
“By its very nature science knows no boundaries. Walling off any group, for any reason, from full participation damages the entire enterprise of science. We must be scientists without borders.” –Rocky Kolb
When we look out at the Universe today, highlighted against the vast, empty blackness of the sky are points of light: stars, galaxies, nebulae and more. Yet there was a time in the distant past before any of those things had formed, just after the Big Bang, where the Universe was still filled with light. If we look in the microwave part of the spectrum, we can find the remnants of this light today in the form of the Cosmic Microwave Background (CMB). But even the CMB is relatively late: we’re seeing its light from 380,000 years after the Big Bang. Light, as far as we know it, existed even before that. After centuries of investigating the origins of the Universe, science has finally uncovered what physically happened to “let there be light” in space.
Let’s take a look at the CMB, first, and where it comes from going way, way back. In 1965, the duo of Arno Penzias and Robert Wilson were working at Bell Labs in Holmdel, New Jersey, trying to calibrate a new antenna for radar communications with overhead satellites. But no matter where they looked in the sky, they kept seeing this noise. It wasn’t correlated with the Sun, any of the stars or planets, or even the plane of the Milky Way. It existed day and night, and it seemed to be the same magnitude in all directions.
After much confusion over what it might be, it was pointed out to them that a team of researchers just 30 miles away in Princeton predicted the existence of such radiation, not as a consequence of anything coming from our planet, solar system or galaxy itself, but originating from a hot, dense state in the early Universe: from the Big Bang.
As the decades went on, we measured this radiation to greater and greater precision, finding that it was not at merely three degrees above absolute zero, but 2.7 K, and then 2.73 K, and then 2.725 K. In perhaps the greatest achievement related to this leftover glow, we measured its spectrum and found it was a perfect blackbody, consistent with the idea of the Big Bang and inconsistent with alternative explanations, such as reflected starlight or tired light scenarios.
More recently, we’ve even measured — from the absorption and interaction of this light with intervening clouds of gas — that this radiation increases in temperature the farther back in time (and redshift) we look. As the Universe expands over time, it cools, and hence when we look farther back into the past, we’re seeing the Universe when it was smaller, denser, and hotter.
So where did this light — the first light in the Universe — first come from? It didn’t come from stars, because it predates the stars. It wasn’t emitted by atoms, because it predates the formation of neutral atoms in the Universe. If we continue to extrapolate backwards to higher and higher energies, we find some strange things out: thanks to Einstein’s E = mc2, these quanta of light could interact with one another, spontaneously producing particle-antiparticle pairs of matter and antimatter!
These aren’t virtual pairs of matter and antimatter, which populate the vacuum of empty space, but rather real particles. Just like two protons colliding at the LHC can create a plethora of new particles and antiparticles (because they have enough energy), two photons in the early Universe can create anything they posses enough energy to create. By extrapolating backwards from what we have now, we can conclude that within the observable Universe shortly after the Big Bang, there were some 1089 particle-antiparticle pairs at that time.
For those of you wondering how we’ve got a Universe full of matter (and not antimatter) today, there must have been some process that created slightly more particles than antiparticles (to the tune of about 1-in-1,000,000,000) from an initially symmetric state, resulting in our observable Universe having about 1080 matter particles and 1089 photons left over.
But that doesn’t explain how we wound up with all that initial matter, antimatter and radiation in the Universe. That’s a lot of entropy, and simply saying “that’s what the Universe began with” is a wholly dissatisfying answer. But if we look to the solution to an entirely different set of problems — the horizon problem and the flatness problem — the answer to this one just pops out.
Something needed to happen to set up the initial conditions for the Big Bang, and that “thing” is cosmic inflation, or a period where the energy in the Universe wasn’t dominated by matter (or antimatter) or radiation, but rather by energy inherent to space itself, or an early, super-intense form of dark energy.
Inflation stretched the Universe flat, it gave it the same conditions everywhere, it drove away any pre-existing particles or antiparticles, and it created the seed fluctuations for overdensities and underdensities in our Universe today. But the key to understanding where all these particles, antiparticles and radiation first came from? That comes from one simple fact: to get the Universe we had today, inflation had to end. In energy terms, inflation happens when you roll slowly down a potential, but when finally you roll into the valley below, inflation ends, converting that energy (from being up high) into matter, antimatter and radiation, giving rise to what we know as the hot Big Bang.
Here’s how you can visualize this. Imagine you’ve got a huge, infinite surface of cubic blocks pushed up against one another, held up by some incredible tension between them. At the same time, a heavy bowling ball rolls over them. In most locations, the ball won’t make much progress, but in some “weak spots” the ball will make an indentation as it rolls over them. And at one fateful location, the ball can actually break through one (or a few) of the blocks, sending them plummeting downwards. When it does this, what happens? With these blocks missing, there’s a chain reaction due to the lack of tension, and the whole structure crumbles.
Where the blocks hit the ground far, far below, that’s like inflation coming to an end. That’s where all the energy inherent to space itself gets converted to real particles, and the fact that the energy density of space itself was so high during inflation is what gives rise to so many particles, antiparticles and photons getting created when inflation ends. This process, of inflation ending and giving rise to the hot Big Bang, is known as cosmic reheating, and as the Universe then cools as it expands, the particle/antiparticle pairs annihilate, creating even more photons and leaving just a tiny bit of matter left over.
As the Universe continues to expand and cool, we create nuclei, neutral atoms, and eventually stars, galaxies, clusters, heavy elements, planets, organic molecules, and life. And through it all, those photons, left over from the Big Bang and a relic of the end of inflation that started it all, stream through the Universe, continuing to cool but never disappearing. When the last star in the Universe flickers out, those photons — long since shifted into the radio and having diluted to be less than one-per-cubic-kilometer — will still be there in numbers just as great as they were trillions and quadrillions of years prior.
Before there were stars, there was matter and radiation. Before there were neutral atoms, there was an ionized plasma, and when that plasma forms neutral atoms, those allow the Universe to deliver the earliest light we see today. Even before that light, there was a soup of matter and antimatter, which annihilated to produce the majority of today’s photons, but even that wasn’t the very beginning. In the beginning, there was exponentially expanding space, and it was the end of that epoch — the end of cosmic inflation — that gave rise to the matter, antimatter, and radiation that would give rise to the first light we can see in the Universe. After billions of years of cosmic evolution, here we are, able to piece together the puzzle. For the first time, the origin of just how the Universe “let there be light” is now known!
Ethan Siegel is the author of Beyond the Galaxy and Treknology. You can pre-order his third book, currently in development: the Encyclopaedia Cosmologica.