Why didn’t black holes exist right after the Big Bang?
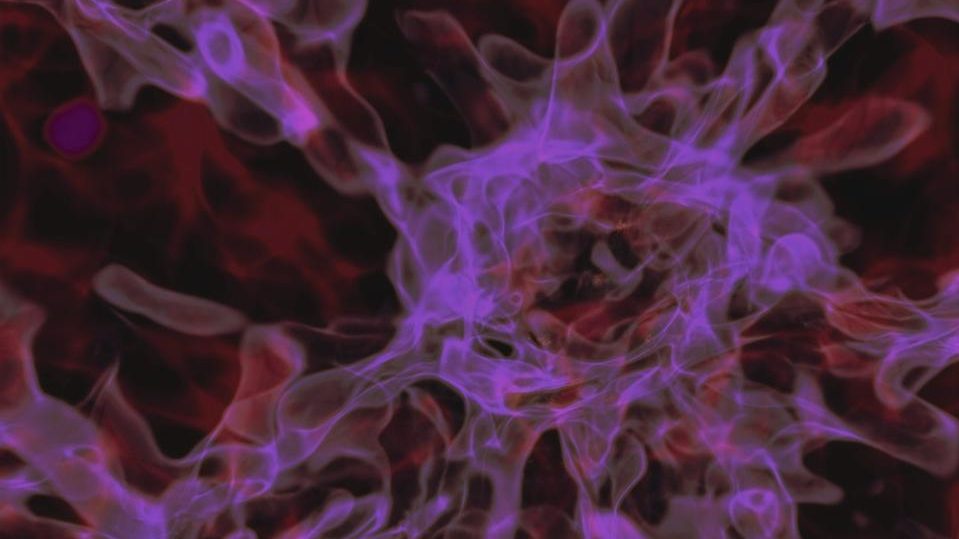
- Although we see no evidence of them, it’s possible that the Universe was born with black holes, or that they formed immediately after the Big Bang.
- This scenario, known as Primordial Black Holes, has substantial observational constraints, but it could be detected in the future by either the James Webb Space Telescope or LISA.
- However, there are even stronger theoretical reasons to expect they don’t exist. Unless something really, really exotic happened, the Universe can’t make them.
Whenever we think about the Universe, it’s fun to imagine what else might be out there beyond the limits of what we’ve discovered so far. But as vast as our imaginations are, we have no choice but to rein them in, as they’re constrained by all that we’ve already seen, measured, and observed taking place within it. At the same time, we have to keep our minds open to novel ways, no matter how exotic, those constraints can be evaded. After all, whatever cannot be ruled out must always be considered, no matter how unlikely or counterintuitive the possibility seems. Just because we know of one way in which things can occur, after all, doesn’t mean we know how everything actually did unfold.
One incredible example of a speculative but fascinating possibility concerns the black holes that exist within our Universe. Sure, we know that our Universe is full of them, and we know of at least three different ways to make them:
- from the core-collapse of a sufficiently massive star
- from the direct collapse of either a massive star or gas cloud
- from the collision of one compact object, like a neutron star, with another
While these are all mechanisms that can successfully make a black hole, they may not be exhaustive. There could be another way to do it: primordially. If the Universe were born with just the right conditions, it could have formed black holes in the early stages of the hot Big Bang, before any stars formed at all. Although it’s a fascinating possibility to consider, it’s extraordinarily unlikely given what we know today. Here’s why.

Credit: S. G. Djorgovski et al., Caltech; Caltech Digital Media Center
The first thing we have to recognize — and this is a big thing to admit — is that we know, to a remarkable degree of certainty, what the Universe was like in the very earliest moments of the hot Big Bang. The second thing we have to recognize is that we also understand the physics of how the overwhelming majority of the ingredients in the Universe behave: how they collide, interact with themselves and one another, and so on. When we combine these two pieces of information, we wind up with something spectacular: the ability to calculate how the Universe evolved during its early stages to astonishing precision, with very little that remains uncertain.
Once the Universe becomes filled with matter and radiation, for example, we know it expands and cools. As it does so, it also gravitates; charged particles collide with radiation; the Universe becomes less dense; the wavelength of each individual quantum of radiation gets stretched with the expanding Universe; and particles can fuse together and/or get blasted apart by interactions with others. The hot Big Bang is, in many ways, the crucible of creation, and we can observe the evidence for much that occurred early on from the relic signals we see today.
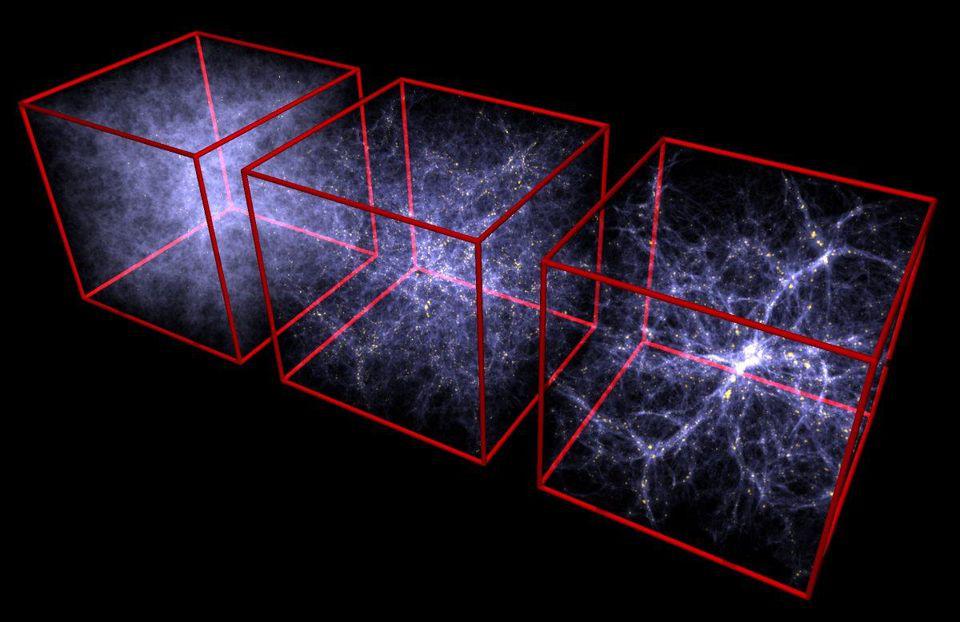
Credit: Volker Springel/MPE
Some of those signals are easy to predict, and many of those predictions have been borne out by observation.
- There’s the large-scale structure of the Universe — the cosmic web of how stars and galaxies group, clump, and cluster together — which requires a mix of dark matter and normal matter to explain, as well as a particular spectrum of initial, seed fluctuations that are needed to form the particular web we have today.
- There are the abundances of the light elements: the elements that existed before any stars were formed, that must have been created from an initial “soup” of protons and neutrons, through the process of nuclear fusion and other nuclear processes like radioactive decays.
- There’s the leftover glow from the Big Bang: the cosmic microwave background. It not only teaches us the temperature of the Universe, but also the extent to which the Universe has expanded throughout cosmic history, the density of photons that exist from the Big Bang at present, and how the energy was distributed among those photons.
On the other hand, there are certain other signals that only arise much later, rather than having been seeded by the early Universe. While they may or may not be easy to spot, it’s a much more challenging task to predict their properties. One of those signals is the existence, abundance, and appearance of the first supermassive black holes: the ones that reside at the centers of massive galaxies within our Universe.

(Credit: NASA/Spitzer/S-CANDELS; Ashby et al. (2015); Kai Noeske)
The factors that separate an “easy” signal to predict — like the three listed items above (which happen to be three of the four cornerstones of the Big Bang, along with the expanding Universe) — from a “hard” one are the circumstances under which it is created.
In the early Universe, an “easy” signal is one where the Universe only very slightly departs from what the average state is. If the Universe were created in an almost perfectly uniform state, with only slight 1-part-in-30,000 departures from that value, then so long as we know the properties of the particles that exist in the Universe sufficiently well, it is easy to calculate how those particles — and the overdense and underdense regions where they’re located — will evolve.
On the other hand, a “hard” signal is one where the Universe has large departures from the average values. It’s sort of like taking a double-pendulum and watching it swing. If you only move the pendulum a small amount away from its equilibrium value, you can predict how that pendulum will behave very accurately, even far into the future. But if you move the pendulum a large amount away from its equilibrium value, things quickly become chaotic, and predictions become far more difficult. In fact, in short order, we can only calculate a likelihood of possible outcomes, rather than any individual outcome, with any modicum of certainty.
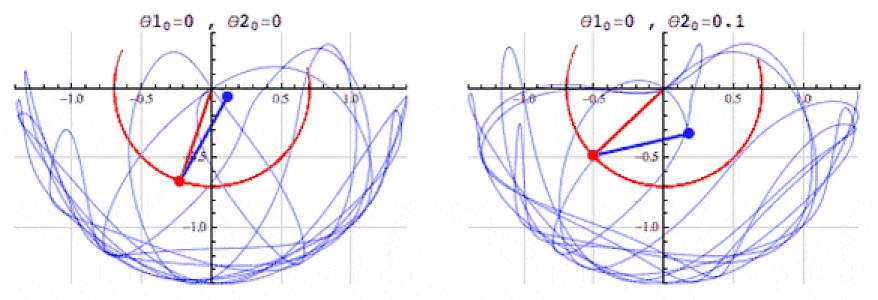
When it comes to the black holes that we observe, however, there may be a problem that primordial black holes could potentially solve. In the youngest galaxies and quasars, which we can measure when the Universe was less than 1 billion years old (and under ~7% of its present age), we still see black holes that are enormous: from many hundreds of millions to more than a billion solar masses. How black holes grew so huge so quickly remains a mystery.
Sure, it’s possible that they were created in one of the known, common ways that the Universe makes black holes. In the early stages of the hot Big Bang, for example, we know that on large cosmic scales the Universe began with the same amount of material, or the same energy density, in every location and in every direction, with deviations occurring at less than the ~0.01% level. It takes about 50 to 200 million years for such a small overdensity to gravitationally grow, accreting enough nearby matter, to lead to gravitational collapse and the formation of the first stars.
Those stars, some of which may be many hundreds or even thousands of times the mass of the Sun, can then form black holes very quickly. They can then merge together, grow through accretion themselves, and become the supermassive black holes we see today.
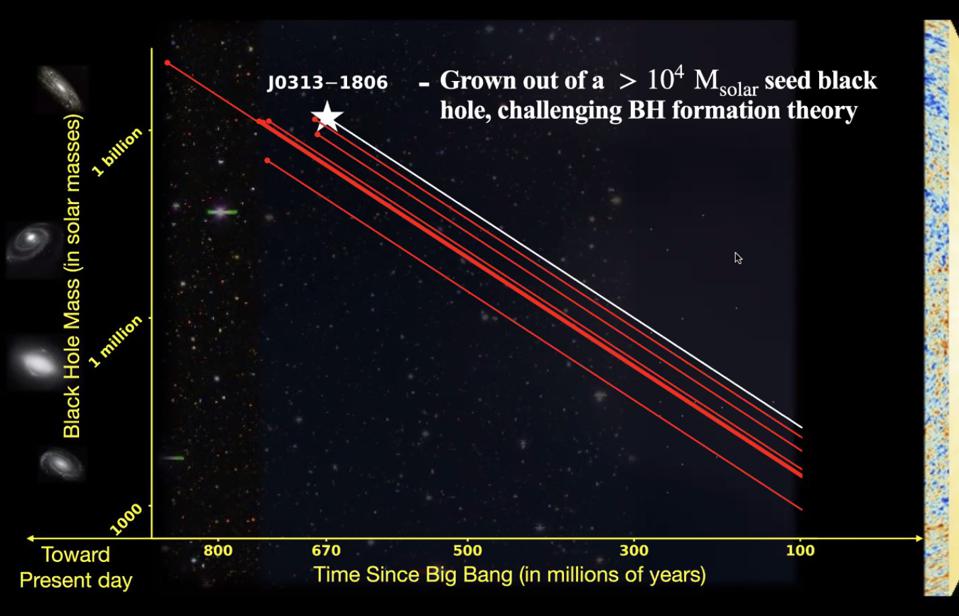
Credit: F. Wang, image taken at AAS237
But even this is a challenge. If you don’t want to invoke something exotic — some new type of physics going on above and beyond what we presently know — you have to presume that something is lacking in our present understanding of these objects. For example:
- black holes are forming earlier and/or more ubiquitously than we currently realize
- they’re merging together more prolifically than we realize
- they’re growing more rapidly than we presently think they’re capable of
All of these are possible, both individually and in combination; it’s far too premature to state that, “it’s impossible for the Universe to make these objects without resorting to new physics.” But we have to recognize that there are many unsolved mysteries to the Universe and certain components to the Universe that are only somewhat understood today.
One of the ideas that could potentially solve some of these problems, and explain how these supermassive black holes got so big so quickly, is the notion that the Universe may have actually formed black holes at an extremely early time: before any stars formed. This is a tremendous leap, but one that could potentially be tested in the very near future.
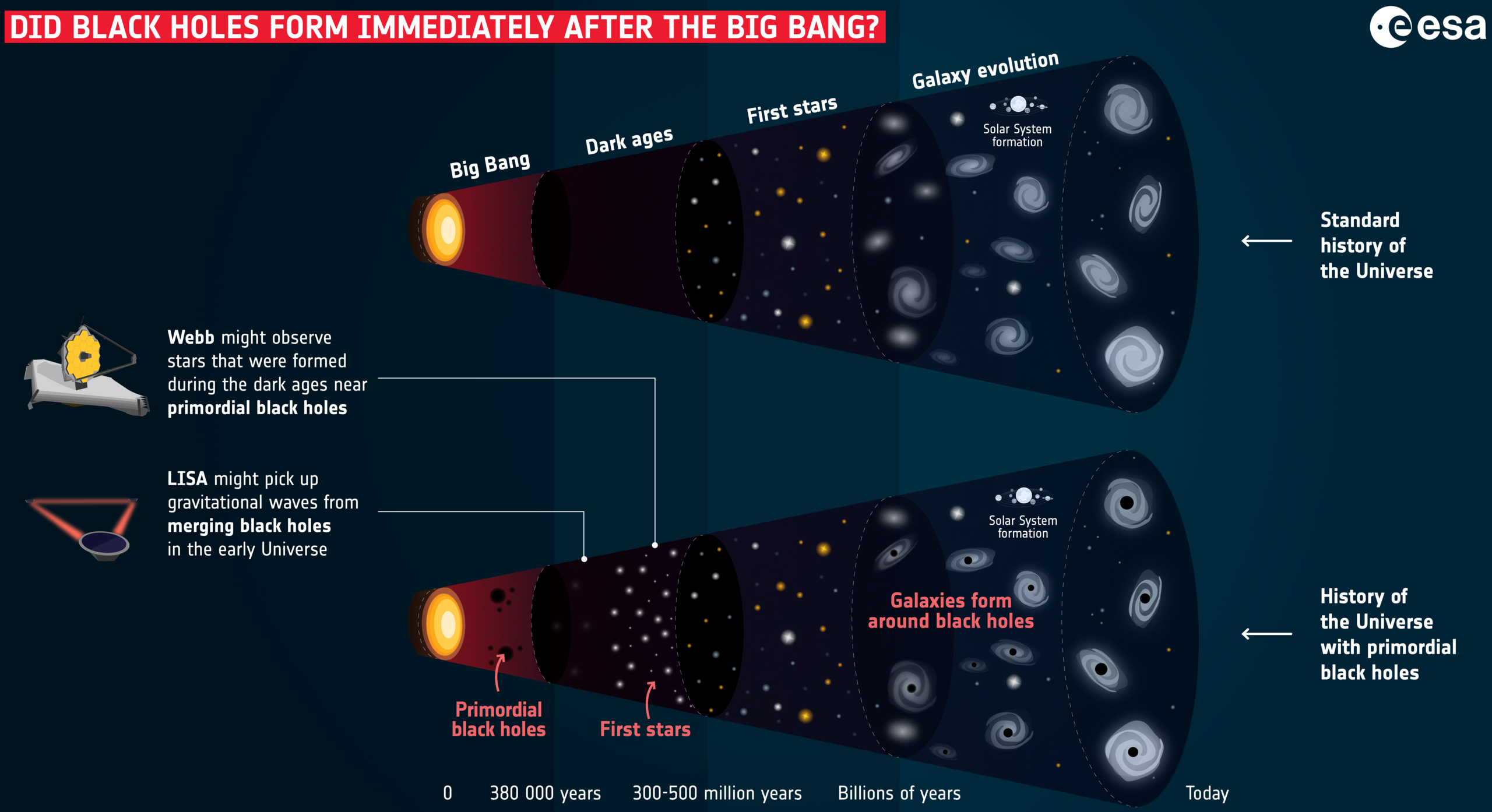
Credit: European Space Agency
If the Universe were born without black holes, which is the standard picture, then we’d have to wait for gravitational collapse to occur and for stars to either form (or, possibly, to be just on the cusp of forming) before the first black holes would arise. Black holes would form in tandem with the first stars and galaxies, and then gravitational growth would proceed from there.
On the other hand, if the Universe were born with these black holes, things would proceed differently. These black holes would behave as extra-strong gravitational seeds, pulling matter into their vicinity from a very early time. The first stars that form would form around these black holes; the environments around the black holes would cause them to grow rapidly; galaxies would form around these black holes; etc.
These two scenarios are so thoroughly different that both the James Webb Space Telescope, with its infrared capabilities, as well as LISA, with its gravitational wave capabilities, would be able to discern one from the other. If much-larger-than-allowed black holes “anchor” the earliest stars that we see, Webb would detect their influence; if massive black holes are spotted merging prior to the formation of stars, LISA would discover them.
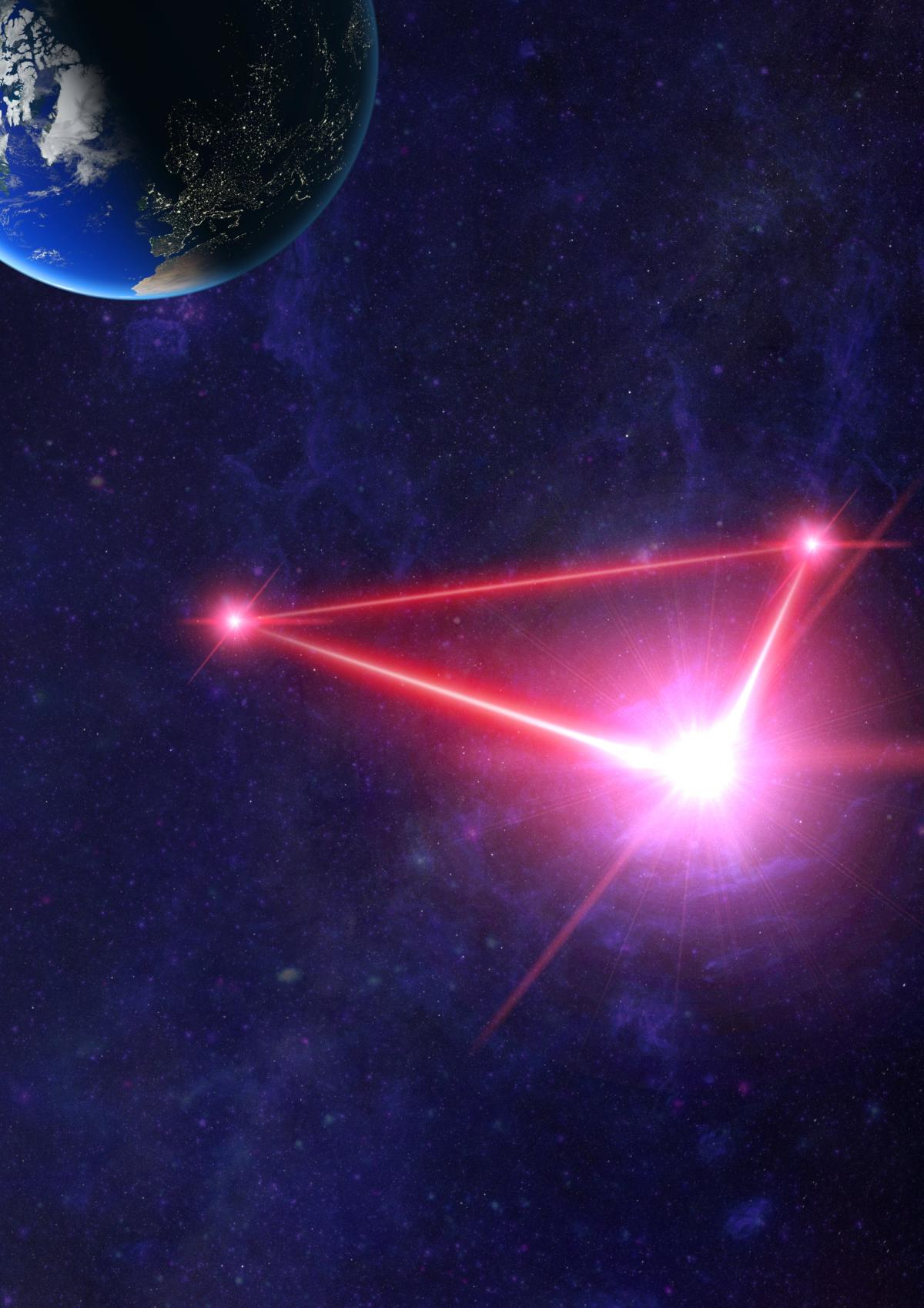
Credit: NASA/JPL-Caltech/NASAEA/ESA/CXC/STScl/GSFCSVS/S.Barke (CC BY 4.0)
However, we cannot just hand-wave a scenario like this into plausibility; we need to understand how structures grow (and also how they don’t grow) in the conditions that existed back in the very early Universe. And when it comes to the physics of cosmic structure formation, this is precisely what we’ve been doing since the 1970s, when the ideas of primordial black holes were first taken seriously and the consequences of their existence were worked out.
When a Universe is filled with matter and radiation, the matter will attempt to gravitationally collapse, but the radiation will resist that gravitational collapse an important way.
When the matter density increases in a region of space, radiation will preferentially flow out of that region, decreasing the overall energy density. When radiation contains more energy than matter does over the entire Universe as a whole — which it does for the first ~9,000 years after the hot Big Bang — this leads to plasma oscillations, which can be seen even today as the “wiggles” in the cosmic microwave background. Over longer timescales, these oscillations will cause the structure on small cosmic scales to be washed out; it’s the larger cosmic scales, which require much longer timescales, that remain and drive the evolution of the cosmic structure we see today.

Credit: NASA/ESA and the COBE, WMAP, and Planck teams; Planck Collaboration, A&A, 2020
If you want to form a primordial black hole, you can’t do it by growing something from a small seed. Instead, you have to start with an enormous seed: something that’s approximately ~68% greater in density than the average. When you compare what we see — which is a large-scale amplitude of approximately ~0.003%, which slowly decreases as we go to smaller scales — it simply cannot admit the creation of primordial black holes.
Unless, that is, we invoke something exotic: something that causes the Universe to have been one particular way, and then it changes all at once, allowing for a large departure from the standard scenario.
This universally requires some sort of phase transition. This could include the phase transition:
- at the end of inflation
- at the electroweak scale (electroweak symmetry breaking)
- during the formation of protons and neutrons (the QCD phase transition)
- during some yet-undiscovered transition
However, this needs to be remarkably tuned to produce a “spike” in the Universe at one particular mass scale, where at one particular mass value, you get the right amount of primordial black holes. At all other scales, you get a negligible amount. If they existed at a wide variety of mass scales, many different observations would have spotted them already.
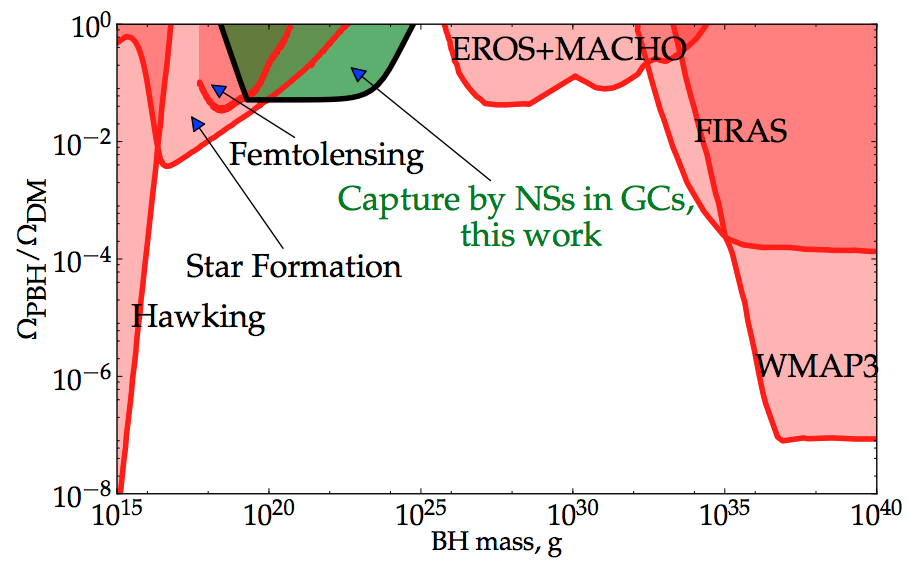
This doesn’t mean we should dismiss the idea of primordial black holes entirely. But it does mean that if we want to cook up a scenario where they’re cosmologically important, these are the obstacles we need to overcome. Interestingly enough, there’s one scenario that no one has yet developed that could be very interesting for their creation: the idea that there was an early form of dark energy that abruptly decayed away. This has been suggested as a potential resolution for why different methods of measuring the expanding Universe yield results that differ by about 9%, but it could also serve double duty: to create large fluctuations at one particular mass scale, potentially leading to an abundance of primordial black holes of a specific size.
Because we know the Universe had a form of energy inherent to space itself during cosmic inflation, and that it has a much lower (but still positive and non-zero) amount of it today in the form of dark energy, it’s plausible that there was some intermediate, in-between state for a time. Transitioning from that in-between state to the state we live in today could potentially generate a narrow spectrum of primordial black holes that evades our current constraints, while solving an astrophysical issue that has remained mysterious until now. In the end, only the data will decide. But with Webb slated to begin science operations in late spring or early summer, we just might get our answer sooner than anyone could have reasonably hoped.