Meet the first star system to “solve” the 3-body problem
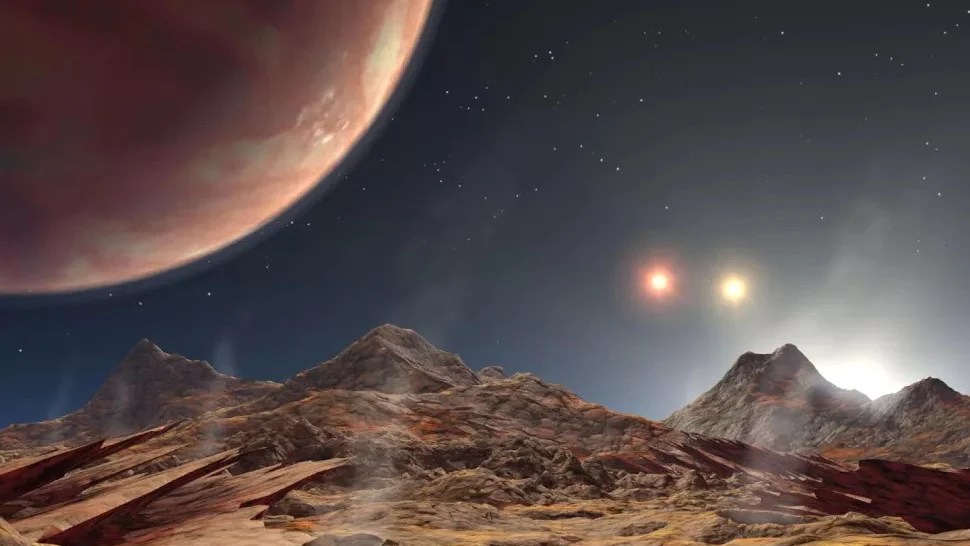
- It’s easy to have planets that orbit around a single star, and in a double star system, you can either orbit close to one star or far from both members.
- These configurations are stable, but adding a third star into the mix was thought to render the formation of planets unstable, as mutual gravitational interactions would eventually force their ejection.
- That wisdom got thrown out the window with the discovery of GW Orionis, which boasts multiple massive dust rings and possibly even more planets, all orbiting three stars at once.
How many stars can a planet successfully orbit before gravitational interactions compel it to be ejected? For a long time, we had only our own Solar System to look to for actual data, as we were the only star we knew of with planets around it at all. It wasn’t until the 1990s that we found planets orbiting other star systems. Even though stars come in singlets, binaries, trinaries, and even greater numbers of multi-star systems, we’d only ever found stars orbiting one — or, at most, two — stars. Even in systems with three or more stars, planets had only been found orbiting one or two of the stars at once, with the other star(s) always orbiting much farther out: at distances where they wouldn’t affect the inner planets’ stabilities.
Why was this the case? There were two main ideas that held sway for decades. One idea was that planets can orbit stars either closely, in which case their orbits are dominated by one central star (or a tight binary star) that supplies their gravity, or far away, as long as they’re far enough so that however many stars there are behave like a single mass. According to this idea, the only reason we hadn’t seen planets in wide orbits around trinary (or greater) systems was because it’s difficult to observe. But a rival idea was that wide planetary orbits around three or more stars would be fundamentally unstable due to the nature of the 3-body problem, and gravitational interactions would quickly kick out any such planets.
While this was contentious for a time, the mystery has been solved with the GW Orionis system: a still-forming trinary system now known, definitively, to house planets of its own. For the first time, planets in orbit around all three stars in the system have been found, suggesting such a configuration is not only possible, but relatively common. Here’s the story of what we’ve learned.
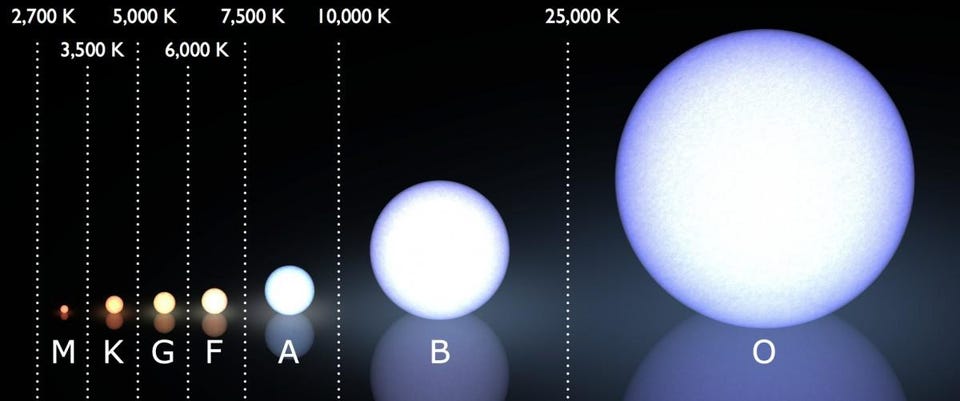
When it comes to the stars that exist in our Universe, a common refrain is that, “Our Sun is just a typical star.” In some ways, we’re well within the realm of what’s considered normal for a star, but typical is really not the right word when you compare it to the other stars we find both near and far.
When it comes to mass, you can either be much smaller or much larger than the sun and still fuse hydrogen into helium in your core: a key requirement for becoming a star. Stars with as little as 7.5% the mass of our Sun, or as much as hundreds of times as massive, all do this throughout the Universe, with the less massive stars being redder, cooler, and longer-lived, while the more massive stars are bluer, hotter, and short-lived. The shortest-lived stars might die after only 1 or 2 million years; the longest-lived ones can persist for at least tens of trillions of years: 1000+ times the present age of the Universe.
Compared to our Sun, the overwhelming majority of stars are redder and lower in mass. By these metrics, we aren’t typical at all. In fact, about 80% of all stars are red dwarfs: stars with no more than 40% the mass of our Sun. Although plenty of stars are brighter, bluer, and more massive than the Sun — particularly among the stars visible to the naked eye in the night sky — these stars are relatively rare. Putting together all that we know, only about 5% of all stars are more massive than our Sun; a full 95% are smaller, redder, longer-lived, and less massive than we are.
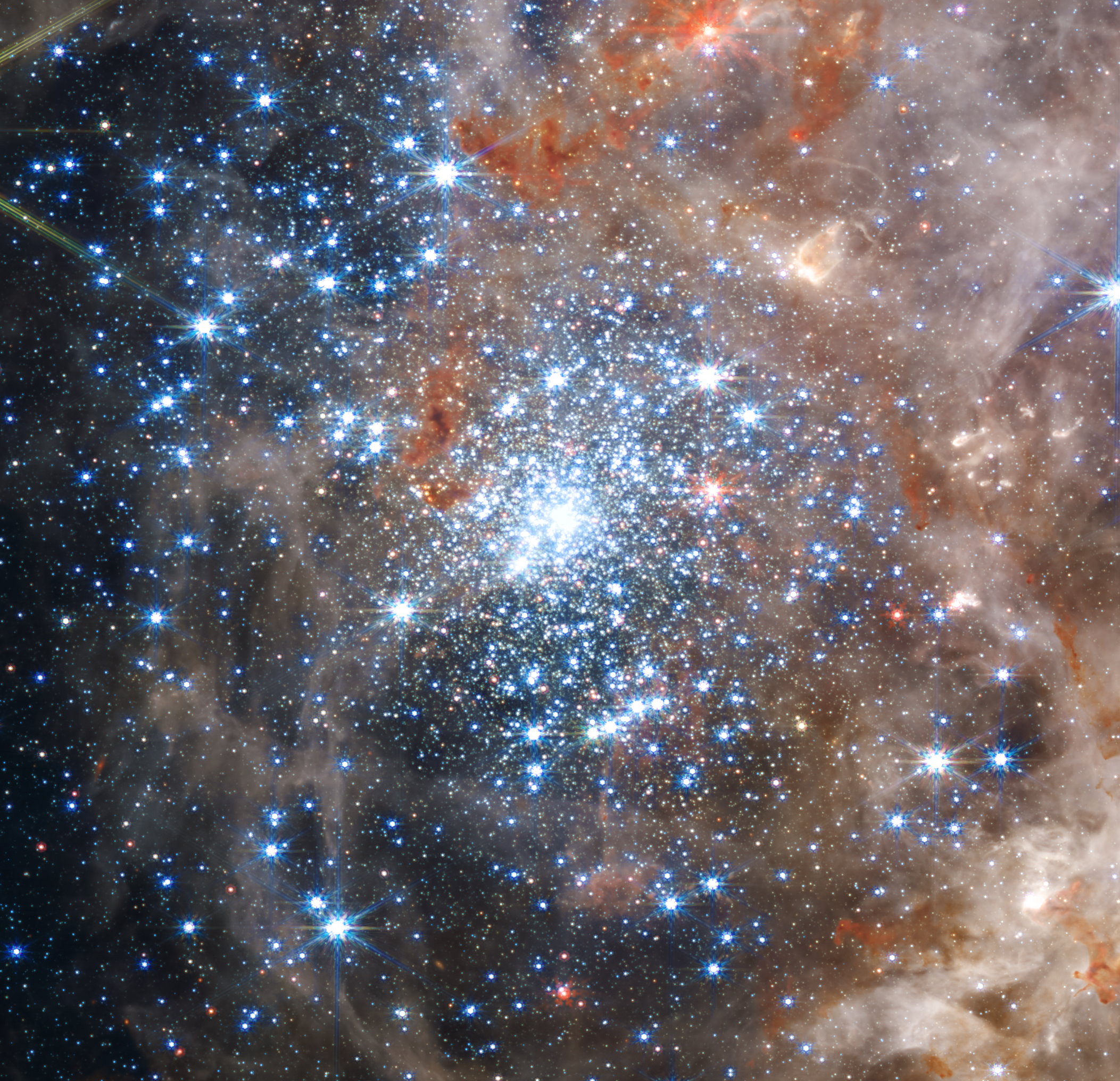
Similarly, when stars are born, they typically form in large, massive star clusters, triggered by the gravitational collapse of a molecular gas cloud. Instead of forming in isolation, typically hundreds, thousands, or even as many as millions of stars can form all at once, within just a few million years of one another: a blink of an eye in astronomical terms. A wide variety of star systems form inside, where only about 1-in-2 stars form in singlet systems, like our own. About 1-in-3 stars are found in binary systems, where two full-fledged stars dominate their solar system; 1-in-10 compose trinary systems, with three stars; 1-in-40 are in quaternary systems, and about 1-in-100 stars exist in systems with 5 or more stars, all bound together under their mutual gravitational pull.
These star clusters consist of star systems with widely varying masses, and over time, they gravitationally interact, causing lower-mass star systems to get ejected while higher-mass star systems sink to the cluster’s center. Over longer timescales — typically around a few hundred million years — these star clusters typically dissociate into individual stellar systems, populating the galaxy with the member star systems that initially were birthed within their home cluster.
But it isn’t simply true that these stars form and persist thereafter. Along with stars, something else forms along with them: planetary systems.
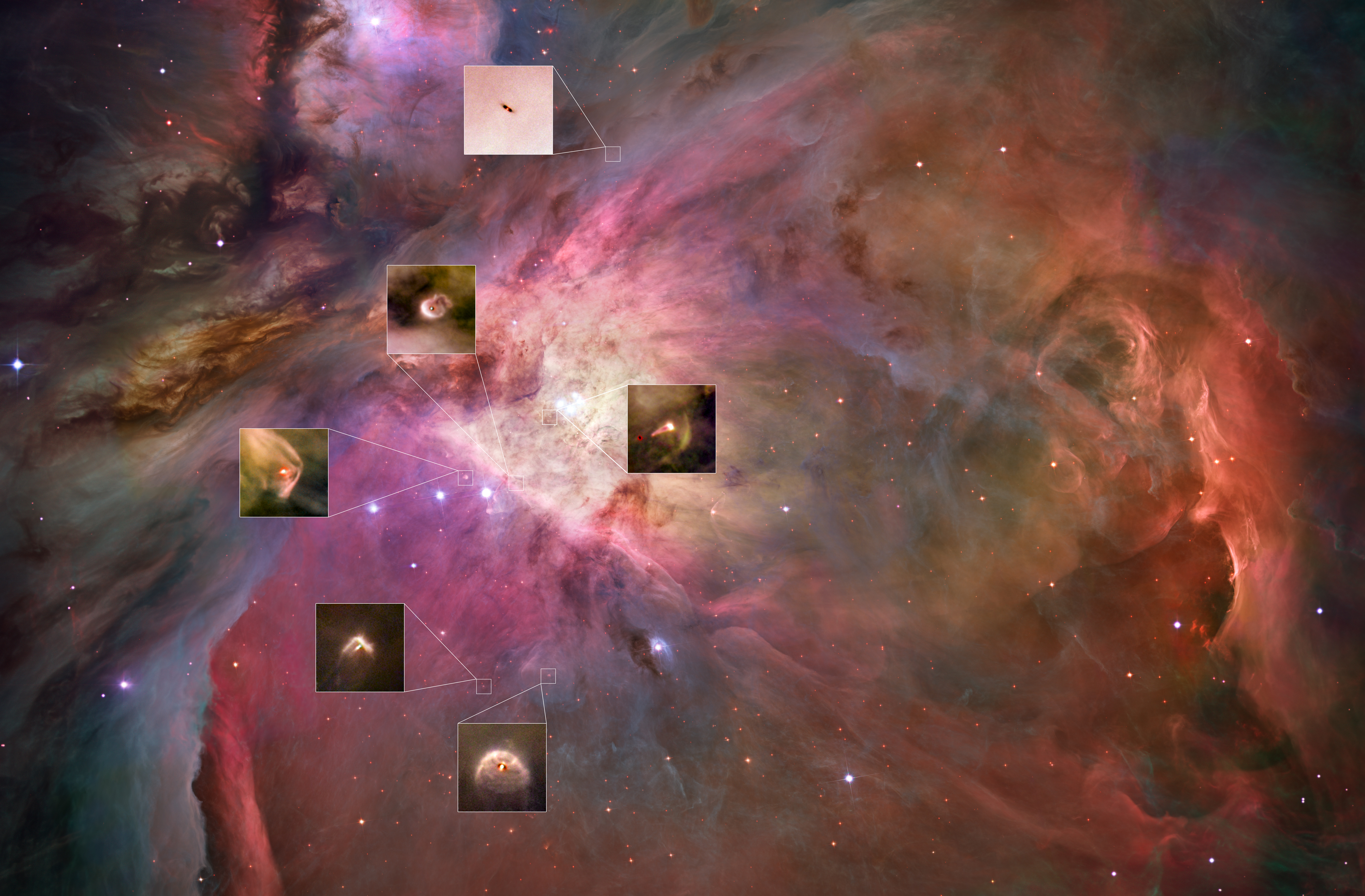
Beginning with observations from the Hubble Space Telescope, humanity began seeing, for the first time, these long-predicted planet-forming systems around young stars and protostars: protoplanetary disks. Rotating around young stars, these dusky disks develop instabilities, where some regions of the disks become slightly denser than others. Regions of greater density will preferentially attract more and more of the surrounding matter to them, eventually growing into protoplanets or even full-fledged planets, sweeping up the dust and creating gaps in the disk, leading to a series of dust rings instead.
When the definition of a planet was put forth, this idea of a massive body around a star “clearing its orbit” wasn’t arbitrary, but rather was related to this very scenario of planet formation in the first place. In theory, what should have happened in all of these systems was the following:
- protoplanetary disks develop instabilities,
- the largest of these instabilities grow and attract other masses,
- this eventually leads to the formation of a planet and a cleared orbit,
- with instabilities and overdensities that form later becoming either less massive planets or sub-planet objects,
- while the radiation and winds from the central star(s) blow the dust away over time,
- leading to a mature stellar-and-planetary system.
Although it took many years and numerous technological advances (in the radio and the infrared, in particular) for these features to be directly observed, we’ve now seen large numbers of protoplanetary disks with these characteristic gaps in them: evidence of newly forming solar systems.
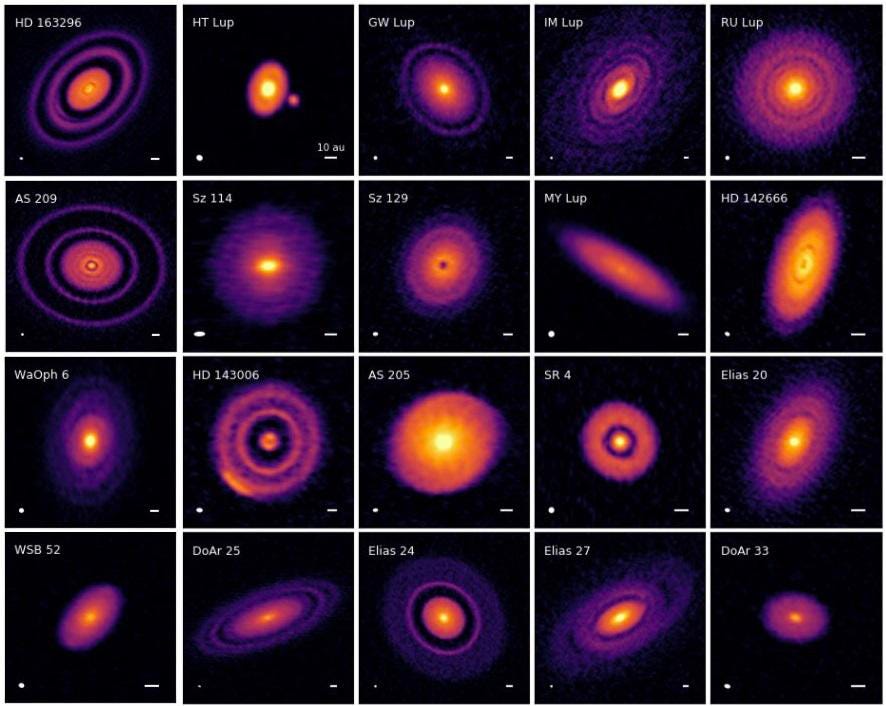
However, you can’t just form a planet anywhere you’d like, especially in the presence of more than one other mass. There’s a problem in physics when you have multiple masses orbiting one another: the fact that if you have more than two masses bound together, the mutual gravitational tugs exerted will lead to unstable orbits. Over time, planets can become:
- ejected,
- hurled into a star,
- or even thrust into orbits where they’ll collide with one another.
Simulations of our own Solar System indicate that this has happened multiple times in our ancient past. We believe we once possessed a fifth giant planet early on, one that was ejected long ago. We know that a Mars-sized body collided with Earth in the Solar System’s early days, giving rise to our Moon. We suspect that a large impact event occurred on ancient Mars, creating its lunar system. Far into the future, simulations indicate that there’s even about a 1% chance that at least one of the four inner planets will be ejected owing to gravitational interactions before our Sun’s core runs out of hydrogen fuel.
With multiple stars in your system, however, it should be forbidden to have planets that orbit any of the stellar members at approximately the distance that separates the stars from one another. In theory, there are only three places where planets can form in a multi-star system.
- You can have a planet orbit in close proximity to one star, where the gravitational force is dominated by that particular star.
- A planet can orbit far away from all of the stars, where the stars are so far away that they effectively behave as a single, point-like mass.
- A planet can orbit with some combination of the first two scenarios, where a planet orbits sufficiently away from some of the stars (like a binary pair), but where one or more stars is even more distant.
However, when it came to trinary systems, only two of these three scenarios were seen to play out.
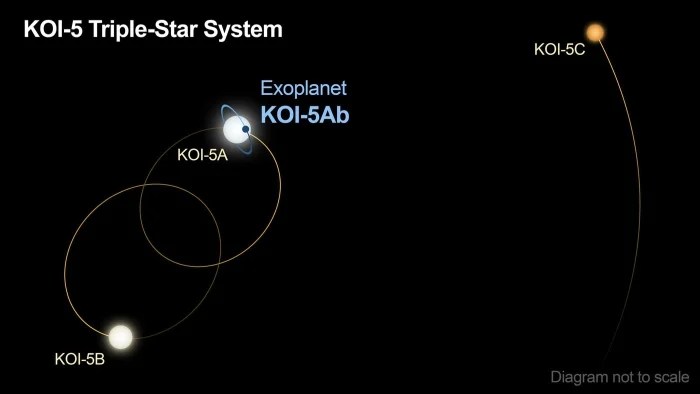
Singlet systems, in a sense, are the simplest: they’re similar to our Solar System. Planets can form anywhere, initially, and then the locations of additional planets are constrained by the presence and properties of the most massive ones. In binary systems, there are two classes of location where planets could stably form: close in to one star or the other, where the other star is much farther away, or at great distances from both stars, where they behave similarly to a single star with the combined mass of both stars together.
But for some reason, of the 32 trinary systems that were observed to possess exoplanets prior to 2020, all of them only had planets that were either:
- close in around one star, with the other two being much farther away,
- or were closely orbiting around a pair of binary stars, with the third (trinary) member much farther away.
Out of those 32, there were no known systems where a planet was found orbiting far out, beyond all three stars in a trinary system: the third theoretical possibility.
However, there was one intriguing system that showed potential: GW Orionis. Located about 1,300 light-years away, GW Orionis is a young trinary star system that still has its protoplanetary material surrounding it. Its member stars are at least a few hundred thousand years old, but no more than 1.3 million years old, making it a system that should be exhibiting early signs of planet formation.
Our knowledge of what was going on in this system suddenly exploded just a few years ago when it was observed with the Atacama Large Millimeter/submillimeter Array (ALMA), the most advanced network of radio telescopes ever built on Earth, and humanity’s best tool for imaging protoplanetary disks directly. Since it became fully operational in 2013, ALMA has revealed incredible details about a large number of young planet-forming systems. When it imaged GW Orionis, it found something truly spectacular: the largest and most impressive system of dust rings ever seen to date.
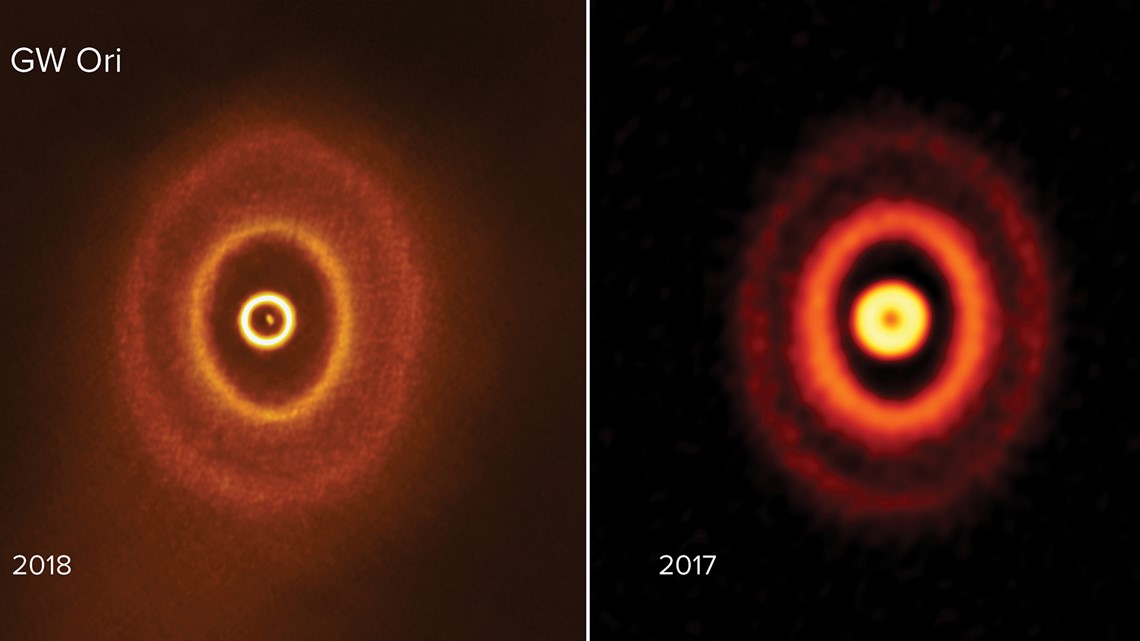
In fact, a simple visual inspection of ALMA’s dust map of the GW Orionis system suggests that there may be planets present in the gaps seen in the dust. However, there’s more to the system than meets the untrained eye, as you may have suspected.
For one, the three stars are set up with two stars orbiting one another at approximately the Earth-Sun distance (about 1 A.U.), with the third star farther away at approximately the distance of Saturn (about 10 A.U.). The dust, however, extends for a great distance: up to approximately 400 times the Earth-Sun distance, or about eight times the full extent of our Solar System’s Kuiper Belt.
Furthermore, the disk is broken up into three rings that each:
- are comparable in mass to large gas giant planets,
- are all tilted and misaligned with respect to one another,
- and that display a total of at least three gaps, one between each of the three rings plus an innermost gap that corresponds to the orbit of the third member of the trinary system.
Could this truly imply a slam dunk of a detection that fills in the missing population of exoplanets in trinary systems? Has GW Orionis shown us that we can indeed have trinary systems where not only planets, but also dust rings, that stably orbit well outside the orbits of all three stars?
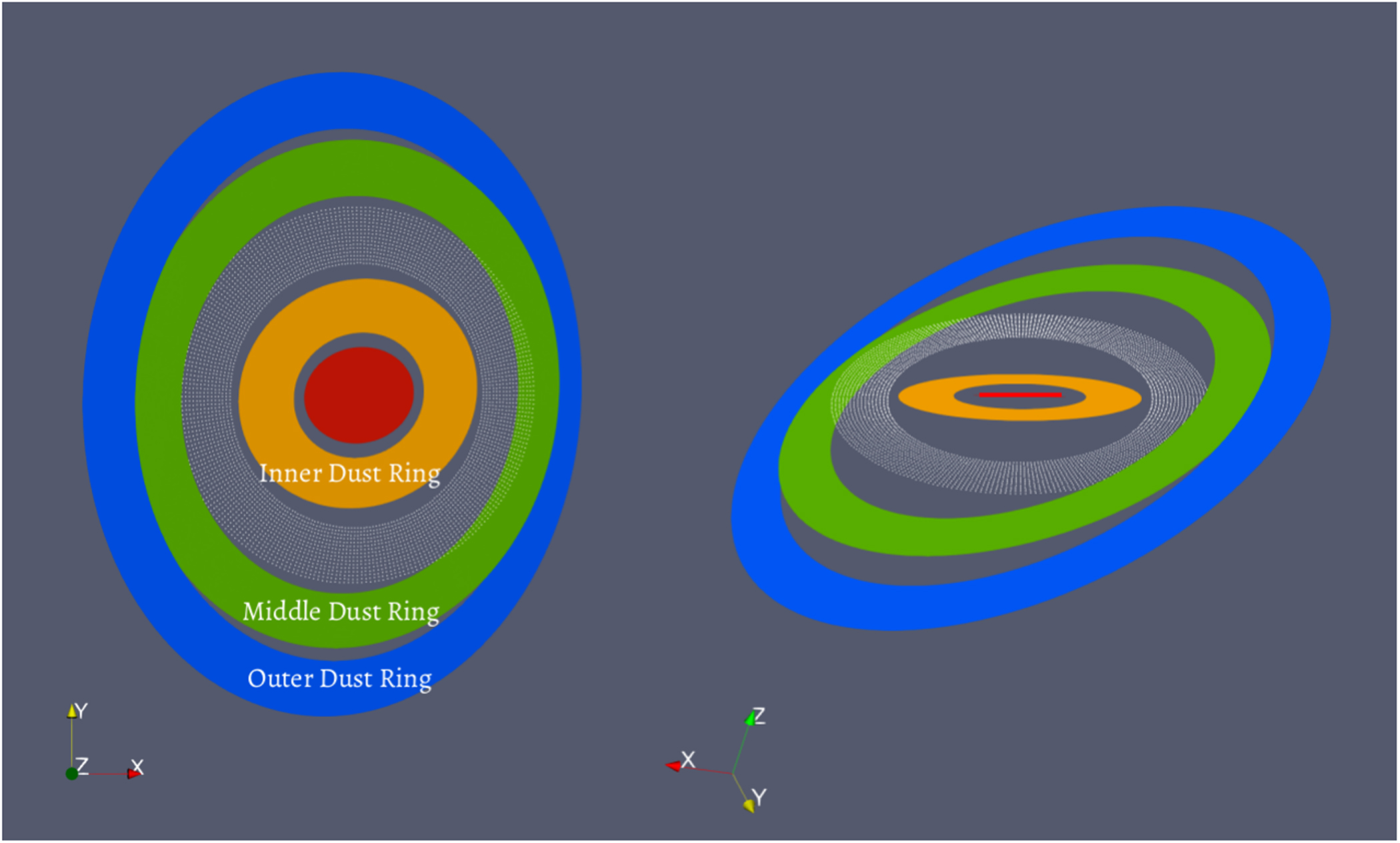
That’s what the initial observations suggested, but in astrophysics, we don’t want to be satisfied with suggestive evidence; we want to be certain. Sure, copious amounts of dust, summing up to about twice the mass of Jupiter, are found orbiting within these three rings that are located outside of these three young, relatively concentrated stars. Yes, the fact that there are major gaps between these rings suggests that they were carved by planets orbiting in between them, but that’s not the only explanation. In fact, the fact that the dust rings are misaligned with one another, as well as with the inner disk that contains the trinary stars themselves, compels us to ask another hard question.
That question is simply: why are the disks misaligned?
One theory is that the three stars themselves must be misaligned; the orbit that the two innermost stars have, orbiting one another, must be inclined at a significant angle to the third, outermore star. Stars that orbit at severe inclinations to one another can exert torques on both interior and exterior matter, suggesting that even earlier in this system’s history, back when the disk remained continuous, it should have been warped.
Two earlier studies then reached opposite conclusions when it came to the gaps: one study concluding that a misalignment of these parent stars could not cause the gaps, while the other concluded that, in fact, they could. Who was right, and how would we tell?
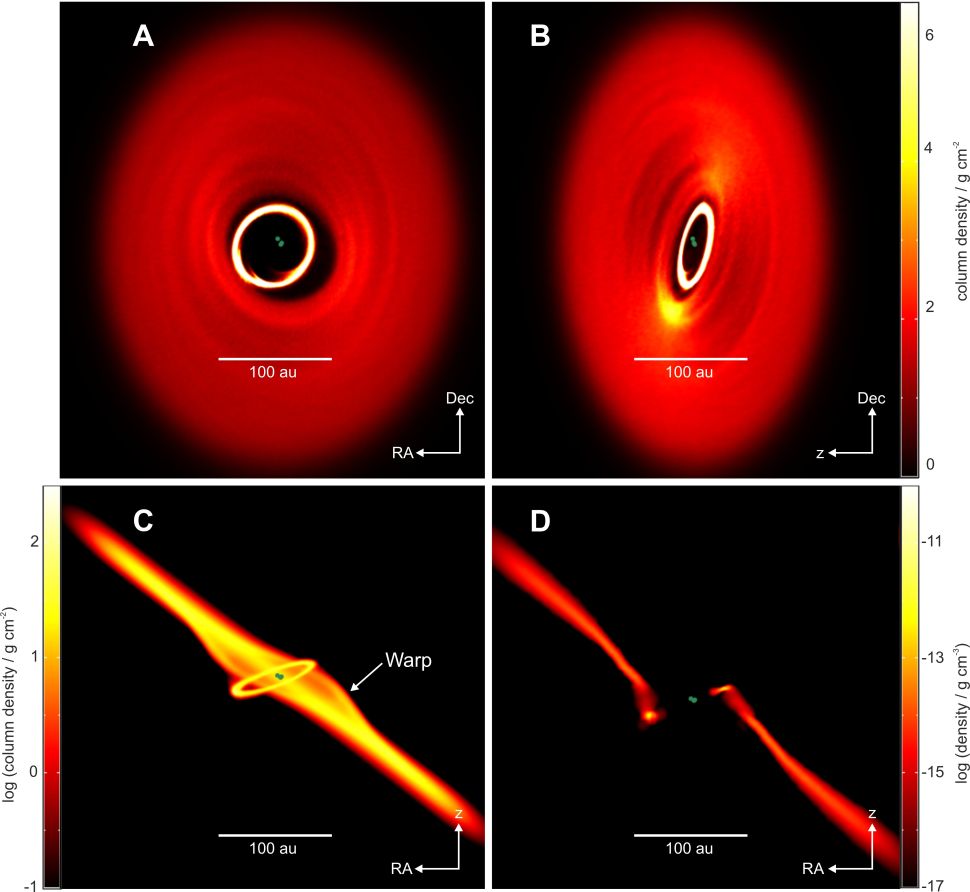
That’s why this study, from late 2021, was so important. It used a novel method — a set of fully three-dimensional hydrodynamical simulations, beginning from initial conditions that admitted both thin and thick dusty disks — to determine what sort of evolutionary tracks are possible for a system like GW Orionis. It also simulated these disks (either thick or thin) both with and without initial “seeds” for potential planets in between the disks, aiming to see whether any of these scenarios could reproduce the observations. And then, most importantly, when they found which parameters did and didn’t reproduce the observations, they went back to determine which solutions were physically compatible with GW Orionis itself.
This study, led by Jeremy Smallwood, showed that the only way to get these “breaks” in the disk without planets — so that you wind up with disconnected dust rings — required the assumption of unrealistic values for the density of the dust within the disk. If you restrict assumptions to realistic values, an initially continuous disk can bend and warp severely, but won’t break in the way GW Orionis appears to have broken.
However, even one initially massive planet can continuously open a gap within a disk. It’s very easy if the disk is thin, and it’s even possible if the disk is thick, as long as the planet is well-coupled to the surrounding gas. The multiple breaks, with gaps, therefore indicate the presence of what must be planets: the first exoplanets, and the first circumstellar dust rings, found to orbit around at least three stars. There’s a very good chance that each of the dust rings, with masses of 74, 168, and 245 Earth masses (moving outward), will go on to eventually form one or more planets apiece, meaning that GW Orionis will likely evolve into a very rich planetary system indeed.
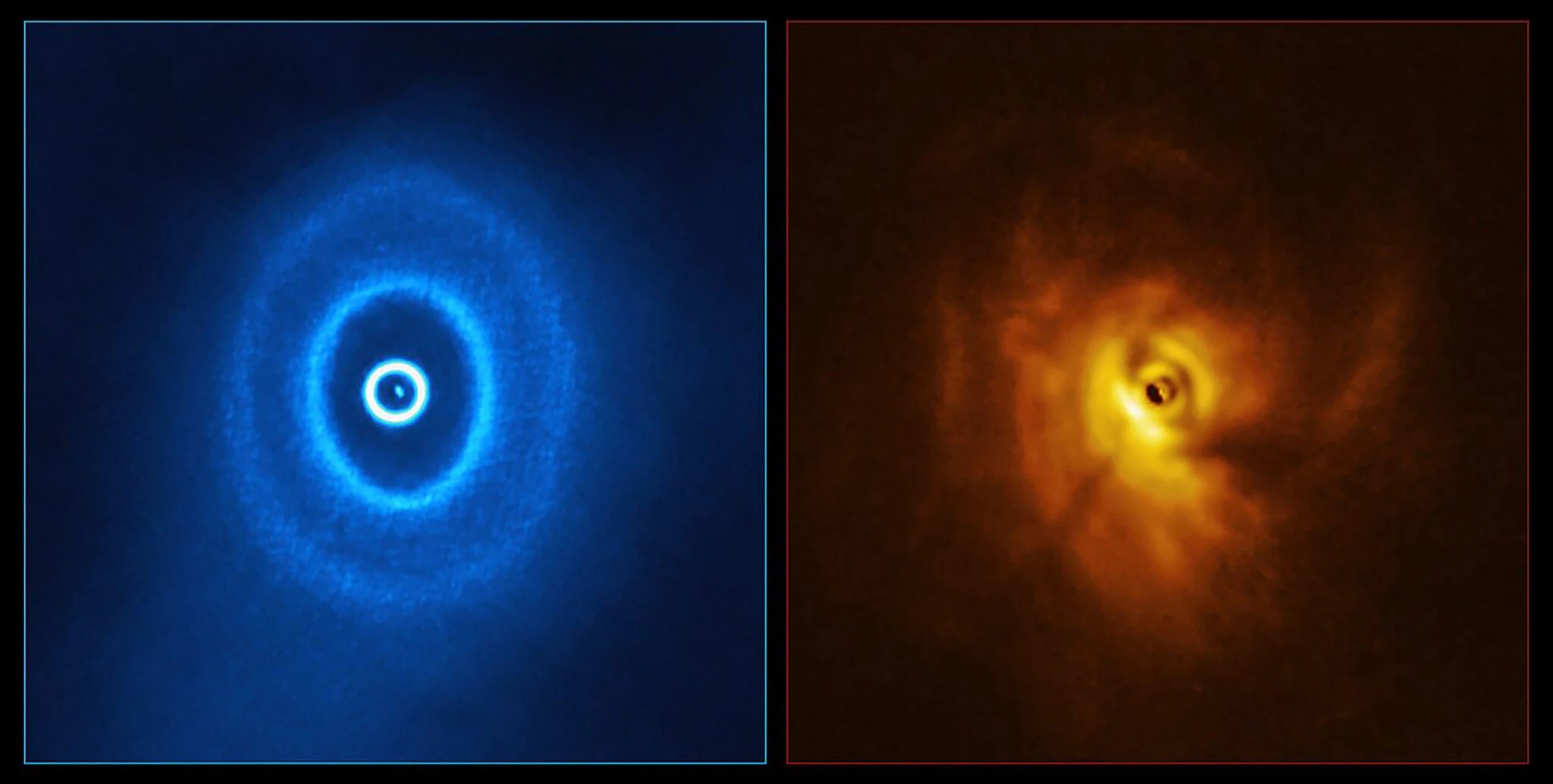
In the span of a mere 30 years, we’ve gone from discovering the very first planets around stars other than our own to learning how and where planets can form. We’ve discovered that they not only are abundant in star systems like our own, but also in binary and trinary systems, including a population of planets that orbits all of its parent stars at once in at least some of those systems. As of today, we have 32 known exoplanet systems where there are at least three stars for the planets to orbit. One of them, GW Orionis, has three tilted and large outer dust rings, separated by major gaps. This can now confidently be interpreted as clear evidence for planets that orbit at great distances from these parent stars.
Although it remains to be seen whether these orbits will persist in a stable fashion over multi-billion year timescales, this new research strongly suggests that planets don’t just form and persist in the inner or intermediate regions of trinary star systems, but also well beyond the orbits of all three stars. It also sets us up for some fascinating tests down the road, because simulations predict that the planets that exist should be further inclined to both the disk and the orbiting stars. These planets could either be quite massive or low in mass, as both scenarios can reproduce conditions consistent with these observations.
For the first time, humanity can not only go beyond the double-sunned world of Tatooine, where we know of systems with three stars in a planet’s sky, but beyond all other trinary systems as well: to a set of planets that orbits around all three stars at once. Constrained only by the laws of physics, it’s a certainty that nature still has a vast array of stellar and planetary configurations just awaiting our discovery.
This article was first published in September of 2021. It was updated in October of 2024.