The nuclear fusion era has arrived, if we choose it

- In December of 2022, the National Ignition Facility reached an unprecedented milestone in nuclear fusion research: passing the vaunted "breakeven" point.
- Now, for the second time and with even better efficiency, more energy was liberated from a fusion reaction than was used to create the fusion reaction: a repeat, verification, and improvement of the original breakthrough.
- The old saying of "fusion is 50 years away, and always will be" is no longer the case. But will we invest enough resources in the right places to bring it to fruition?
Last year, on December 5, 2022, an incredible milestone was achieved: for the first time, a nuclear fusion reaction experienced what’s known as a net energy gain. This means, remarkably, that the energy liberated from a nuclear fusion reaction exceeded the (useful) energy that was inputted into the reaction. This wasn’t achieved by a magnetic confinement fusion reactor, which is where most of the worldwide fusion funding is centered, nor by any among the hundreds of private laboratories dedicated to bringing commercial fusion to the public, but rather by a largely forgotten source: the National Ignition Facility at Lawrence Livermore National Laboratory.
This year, on July 30, 2023, the National Ignition Facility did it again, and in an even superior fashion: repeating their results and achieving an even higher energy yield than in the December prior. All of this was achieved despite a paltry amount of funding being directed toward nuclear fusion research by the U.S. government: an average of just half-a-billion dollars per year across all endeavors, combined. With this recent confirmation, the path toward developing widespread nuclear fusion as the anchor to a clean, carbon-neutral energy economy is now clearer than ever. But in order to truly achieve it, we not only need to be brave and bold, but also focused, as the distractions and pitfalls could truly divert us from the ultimate goal.

The ultimate goal
Let’s not mince words about this: the ultimate goal is to create virtually unlimited amounts of energy via the process of nuclear fusion, enabling humanity’s transition to a clean-energy economy. This would be an economy free from:
- the pollutive effects of fossil fuels,
- the need to mine and extract rare raw materials,
- the risk of a nuclear power plant melting down or exploding,
- and the fickle, unsteady supply arising from current green energy sources like wind, hydroelectric, or solar power.
We’re all well aware of the pollutive effects of energy generation, as well as the effects that rising carbon dioxide levels are having on global temperatures, water availability, ocean acidification, and many other ecological aspects of our world. We’re also keenly aware of the need for a safe, reliable, but extremely productive source of energy in order to power the modern lives of more than 8 billion (and rising) humans on planet Earth.
Yet the very power that’s locked inside the Sun, where light elements fuse into heavy ones under high densities and extreme temperatures, could hold the ideal solution to our energy generation needs. That’s the promise of nuclear fusion.
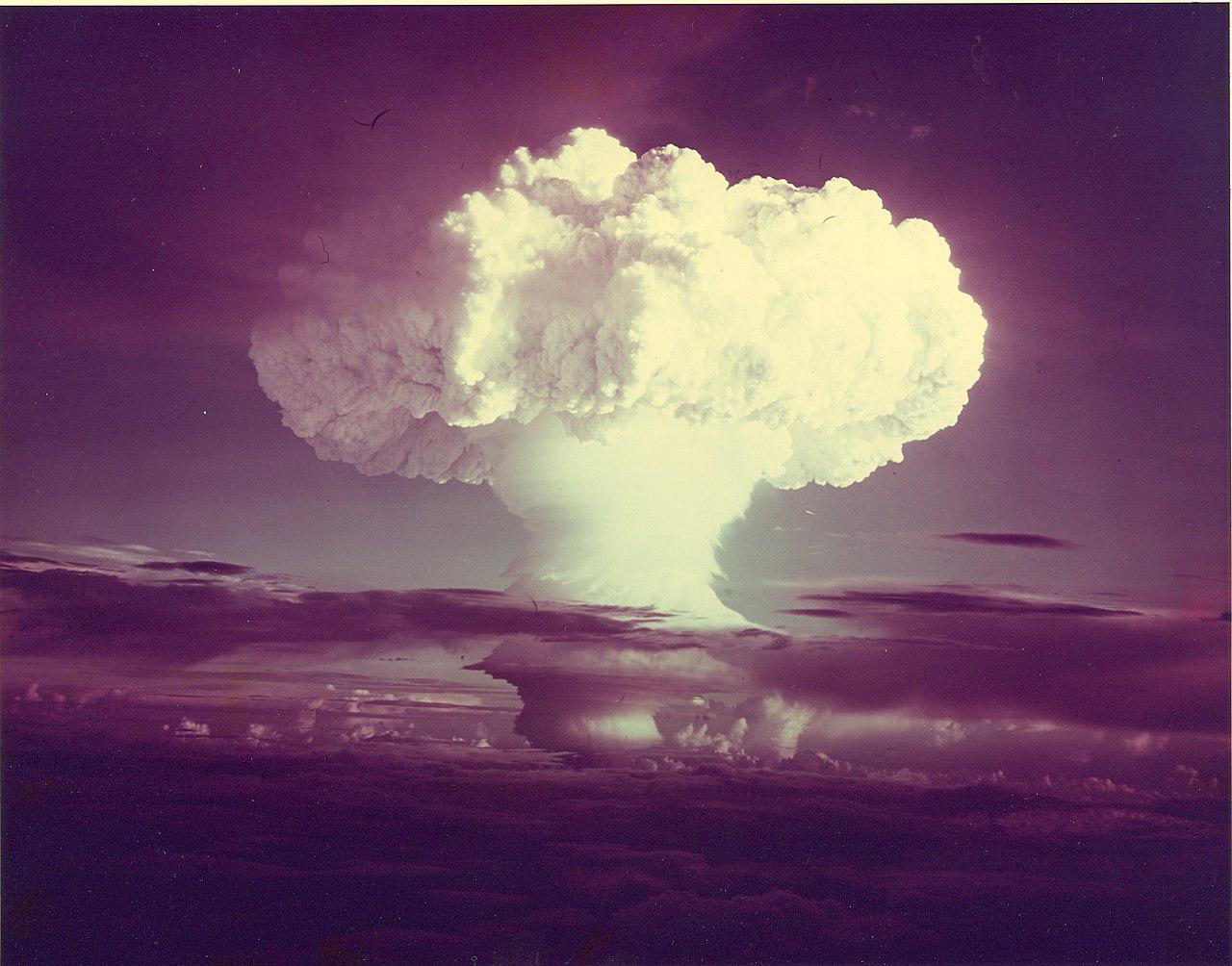
But in order to get there, it isn’t simply enough to generate these nuclear fusion reactions: something we’ve been capable of doing for more than 70 years. The hydrogen bomb, masterminded by Edward Teller, is a spectacular example of nuclear fusion here on Earth. However, that energy can’t be readily converted into usable electrical power, as it’s far too great in magnitude — too much of an “all at once” phenomenon — to harness.
Instead, we need to generate nuclear fusion reactions in a controlled, repeatable fashion. That fusion energy must be emitted in small doses, either continuously or in bursts, where that energy can then be used to do things like boil water, turn a turbine, or perform mechanical work that can then be extracted and transformed into usable electrical energy, just like conventional power plants.
Then, there will be engineering and efficiency concerns, like:
- how can we maximize the net energy gain,
- how can we minimize the energy required to initiate the fusion reactions,
- how can we generate the needed energy in an on-demand fashion,
- how can we transport this fusion-generated energy over long distances,
- how can we successfully maintain the equipment used for generating these reactions,
- and how do we successfully absorb any stray neutrons emitted in the fusion process, and prevent any radioactive materials generated from these reactions from contaminating our environment?
But in order to get there, we first need to pass the breakeven point: the point at which more energy is generated from fusion reactions than is required to initiate those reactions in the first place.
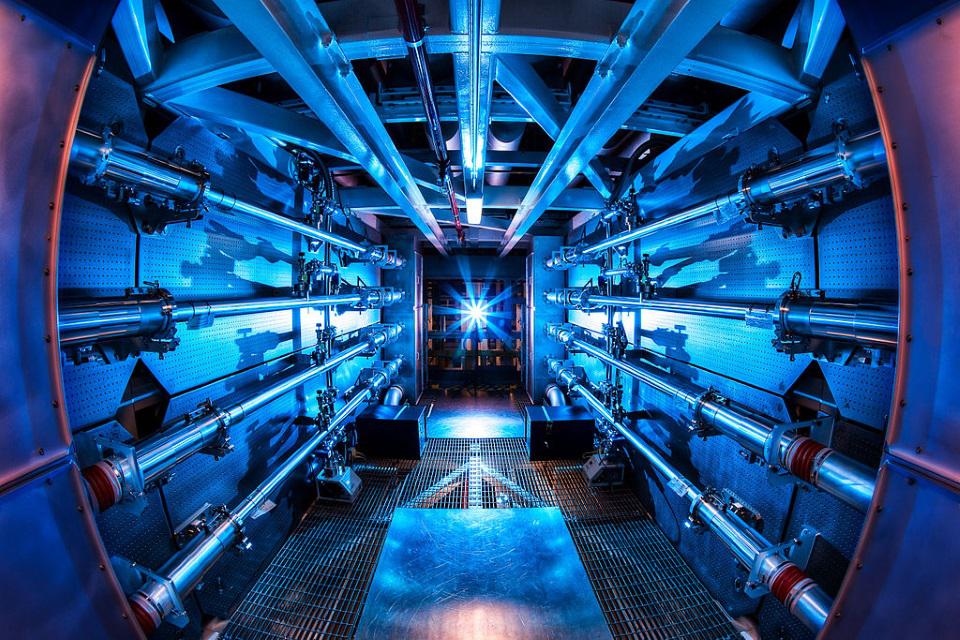
The present achievement
This was a big deal when the National Ignition Facility first announced it in December of 2022, and it’s a big deal that they did it again (proving reproducibility) at the end of July 2023: they have demonstrated that they have passed the breakeven point in a fusion reaction. They have achieved a net energy gain, where if you look at the amount of energy that goes into creating the fusion reaction — the amount of energy incident on the target — and compare it to the amount of energy that gets generated via fusion in the ensuing reaction, the energy generated is greater than the energy that went into it.
To be clear, however, this passes the “breakeven” point the way scientists talk about it, not the way that most people, and most businesses, talk about it. The difference is as follows.
- When a scientist talks about the “breakeven” point (or “net energy gain” in this context), they talk about the amount of energy that goes into this specific step of the reaction versus the energy that comes out of it. They do not include the energy used in the preparatory steps to set up the system, nor do they include any steps that would be necessary to extract this energy and convert it into some sort of energy that’s usable downstream.
- On the other hand, most of us, when we talk about “breaking even” in terms of energy (or anything else), we only care about the overall, beginning-to-end totals: how much, total, that we put in versus how much that’s usable we get out.

If we stick to that second definition, as some insist upon, then we are still a long way from the breakeven goal. All of the “engineering and efficiency” concerns must still be dealt with, and overcome, before we have a commercially viable nuclear fusion power plant.
But we must not undersell the importance of what the National Ignition Facility has achieved. In December of 2022, they fired 192 lasers, from all directions, onto the walls of a pellet that was filled with light elements: the same elements and isotopes that are found ubiquitously in the Sun. The total amount of energy from the lasers that hit the target, as measured in joules (the physicist’s unit of energy, where one joule-per-second is the more familiar watt, the unit of power), was 2.05 megajoules. The amount of energy liberated, or released in the ensuing fusion reaction, was more than that: 3.15 megajoules. That’s a net energy gain from the reaction, and improvements in either:
- the number of firing lasers,
- the total amount of incident energy,
- or the geometry of the laser strike on the pellet,
would only be expected to increase the energy gain. Although the laboratory performing the experiment, Lawrence Livermore National Laboratory, has not released the final figures and details just yet, they have confirmed that there was an even greater net energy gain during the July 2023 test than the earlier December 2022 test. We are now in the post-breakeven era, where “energy out” is greater than “energy in” for the most important portion of the reaction: the one where fusion reactions are created.

The other approaches: a mix of science and hype
The approach used by the National Ignition Facility, where a small pellet is heated to tremendous temperatures, triggering fusion reactions inside this hot, hydrogen-rich volume, is known as inertial confinement fusion. It’s like a very, very small explosion, where the mass of the surrounding material (i.e., the inertia of the outer layers of the pellet) is what confines the fusion reaction, enabling it to last long enough to achieve a net energy gain.
However, it isn’t the only valid approach.
There’s also magnetic confinement fusion, where a heated plasma is confined within a torus-like shaped device called a tokamak, and magnetic fields are used to keep the plasma confined. At these temperatures and densities, nuclear fusion reactions occur, but keeping the plasma confined — preventing it from hitting either the inside or outside walls and keeping it in a state where fusion reactions can continually occur in a sustained fashion — is the most challenging aspect of this. There are small-scale, independently operating tokamaks all across the world, and the largest international effort to build such a fusion reactor, ITER, is already looking forward to interesting results. At present, the JET tokamak in England, in December of 2021, produced 59 megajoules of sustained energy over a period of 5 seconds, but no one is expecting magnetic confinement fusion to surpass breakeven until ITER is operational.

There are also “hybrid” approaches, which attempt to leverage aspects of both the inertial confinement method (such as compression) and the magnetic confinement method (such as the use of magnetic fields and plasmas) to achieve fusion, with enormously varying levels of success, but none approaching what the various tokamaks and the National Ignition Facility have achieved.
Many of these hybrid approaches are interesting from a physics perspective and have many bona fide physicists involved, but charlatans are ubiquitous in this arena as well, including all endeavors that are involved with so-called “cold fusion” or its attempted rebrand as low-energy nuclear reactions (LENR), as those attempts have failed every experimental test so far. (With the exception of muon-catalyzed fusion, which is not a viable pathway to commercial fusion.)
The key to any fusion endeavor’s success is the long-term production of fusion reactions that achieve a net energy gain. While there are many wild claims that many private sector firms are claiming, only tokamak-type approaches and the National Ignition Facility have any prospects for achieving and surpassing that vaunted breakeven point in the foreseeable future.

The problem of underinvesting in fusion research
One of the most puzzling aspects of nuclear fusion is simply, if climate change is so expensive and we’ve now passed the breakeven point (the major physical obstacle to commercial viability) with nuclear fusion, then why aren’t we investing any substantial monetary resources to bring nuclear fusion to fruition?
According to publicly available data, the United States government, through the Department of Energy, has been funding nuclear fusion research since 1954. Using inflation-adjusted numbers (to modern-day dollars) over that time, we’ve invested a cumulative total of $34 billion in fusion research: including contributions to ITER. For comparison, it’s estimated that climate change-related extreme weather events cost the United States $165 billion last year.
Think about that. In 2022, extreme weather events, fueled by climate change, cost the United States (alone) $165 billion. And cumulatively, from 1954-2021 (the last year I have data available for), over that 68 year time period, we invested a total of $34 billion toward nuclear fusion research: an average of just $0.5 billion per year.
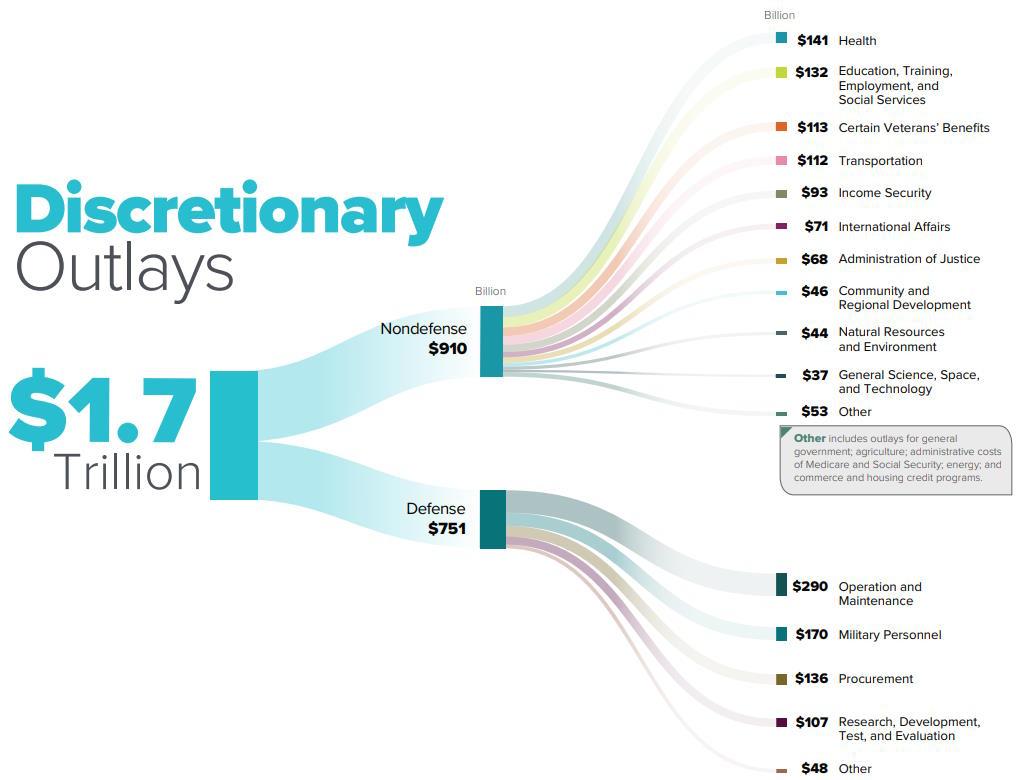
It’s truly amazing how much scientists have accomplished with so little; out of the $1.7 trillion that the USA annually spends on discretionary outlays, only $0.0005 trillion of that, or less than 0.03%, goes toward the scientific investigation of the clearest pathway to solving Earth’s energy and climate issues. If ever there was a place where we should be putting our money to get the greatest long-term return-on-investment, this is it.
Yes, there is a lot of work left to be done on all fronts. If ITER can achieve breakeven with magnetic confinement fusion, then the pathway to commercial fusion becomes clear: with advances needed in the fields of:
- advanced modes of plasma operation,
- better understanding and controlling plasma-wall interactions,
- the testing of materials that can withstand the conditions inside a tokamak,
- and power extraction methods,
- in order to bring this to commercial viability.
While many assert that the engineering associated with inertial confinement fusion is farther away from bringing it to commercial viability, the fact remains that what the NIF achieved — a net energy gain from a fusion reaction — shows us that, if we want it, there’s at least one path readily available that will clearly take us to a commercial fusion reactor.

The path to success
If we want it, the way to make a viable fusion reactor using the National Ignition Facility’s setup looks as follows.
- We need to prepare a way to efficiently create incident energy on a fusion pellet, repeatably, of between 2.05 MJ (where breakeven was achieved) all the way up to 5 MJ, which is where maximum energy yield/efficiency is expected.
- We need to find a way to add new and remove used pellets from the setup and prepare the new sample for fusion in a repeatable, rapid fashion.
- We need to build a mechanism capable of converting this produced energy into extractable, usable energy for transmission to the electrical grid.
- We need a way to successfully store and produce energy so that it can be distributed as needed, as “creation on demand” may be difficult due to the pulsed energy generation nature of this type of fusion.
- And we need to solve the problem of free neutrons created during this fusion reaction, in order to prevent the accumulation of dangerous radioactive waste.
None of these, however, are insurmountable scientific problems; they are problems that should be addressable within the normal course of research and development. The enormous point is: the science is now here, and has been demonstrated in a repeatable fashion, to bring nuclear fusion technology into our lives during the second half of the 21st century. The only question is whether we’ll continue to be pound-foolish and penny-wise when it comes to developing this technology, or whether we’ll finally embrace cutting-edge science, along with all the good it has the potential to bring to planet Earth.