Newest LIGO signal raises a huge question: do merging black holes emit light?
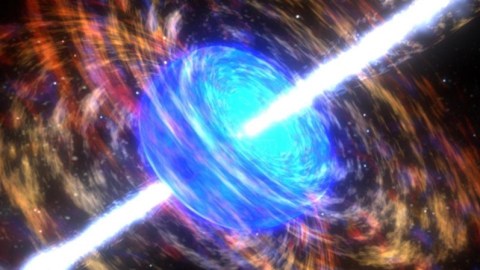
Gravitational waves and electromagnetic ones don’t need to go together. But physics says it’s possible; what do the observations say?
“The black holes collide in complete darkness. None of the energy exploding from the collision comes out as light. No telescope will ever see the event.”
–Janna Levin
Billions of years ago, two black holes much more massive than the Sun — 31 and 19 solar masses each — merged together in a distant galaxy far across the Universe. On January 4th of this year, those gravitational waves, traveling through the Universe at the speed of light, finally reached Earth, where they compressed and stretched our planet by the width of no more than a few atoms. Yet that was enough for the twin LIGO detectors in Washington and Louisiana to pick up the signal and reconstruct exactly what happened. For the third time ever, we had directly detected gravitational waves. Meanwhile, telescopes and observatories all over the world, including in orbit around Earth, were looking for an entirely different signal: for some type of light, or electromagnetic radiation, that these merging black holes might have produced.
According to our best models of physics, merging black holes aren’t supposed to emit any light at all. A massive singularity surrounded by an event horizon might emit gravitational waves, due to the changing curvature of space time as it orbits an inspirals with another giant mass, in line with General Relativity’s predictions. Because that gravitational energy, emitted as radiation, needs to come from somewhere, the final black hole post-merger is about two solar masses lighter than the sum of the originals that created it. This is completely in line with the other two mergers LIGO observed: where around 5% of the original masses were converted into pure energy, in the form of gravitational radiation.
But if there’s anything outside of those black holes, such as an accretion disk, a firewall, a hard shell, a diffuse cloud, or any other possibility, the acceleration and heating of that material could conceivably create electromagnetic radiation traveling right alongside those gravitational waves. In the aftermath of the first LIGO detection, the Fermi Gamma-ray Burst Monitor made headlines as they claimed to detect a high energy burst of radiation coincident within a second of the gravitational wave signal. Unfortunately, ESA’s Integral satellite not only failed to confirm Fermi’s results, but scientists working there uncovered a flaw in Fermi’s analysis of their data, completely discrediting their results.
The second merger held no such hints of electromagnetic signals, but that was less surprising: the black holes were of significantly lower mass, so any signal arising from them would be expected to be correspondingly lower in magnitude. But the third merger was large in mass again, more comparable to the first than the second. While Fermi has made no announcement, and Integral again reports a non-detection, there are two pieces of evidence that suggest there may have been an electromagnetic counterpart after all. The AGILE satellite from the Italian Space Agency detected a weak, short-lived event that occurred just half a second before the LIGO merger, while X-ray, radio and optical observations combined to identify a strange afterglow less than 24 hours after the merger.
If either of these were connected to the black hole merger, it would be absolutely revolutionary. There is so little we presently know about black holes in general, much less merging black holes. We’ve never directly imaged one before, although the Event Horizon Telescope hopes to grab the first later this year. We’ve only just this year determined that black holes don’t have hard shells encircling the event horizon, and even that evidence is only statistical. So when it comes to the possibility that black holes might have an electromagnetic counterpart, it’s important to keep an open mind, to look, and to go wherever the data takes us.
Unfortunately, neither one of these observations provide the necessary data to take us to a place where we’d conclude that merging black holes really do have a light-emitting counterpart. It’s very difficult to get compelling evidence in the first place, since even the twin LIGO detectors, operating with their incredible precision, can’t pinpoint the location of a gravitational wave signal to better accuracy than a constellation or three. Since gravitational waves and electromagnetic waves both travel at the speed of light, it’s extraordinarily unlikely that there would be nearly a 24 hour delay between a gravitational wave signal and an electromagnetic signal; in addition, that transient event appears to occur at a distance far too great to be associated with the gravitational wave event.
But the AGILE observations may potentially provide a hint that something interesting is going on. At the moment that the gravitational wave event occurred, AGILE was pointed at a region of space that contains 36% of the candidate LIGO region. And they do claim an “excess of detected X-ray photons” coming from somewhere on the sky over the standard, average background. But when you look at the data yourself, you have to ask yourself: how compelling is this?
Over a few seconds before-and-after the LIGO merger, they pulled out an interesting event that they identify as “E2” in the three charts above. After doing a full analysis, where they account for what they saw and what sort of random fluctuations and backgrounds just naturally occur, they can conclude that there’s about a 99.9% chance that something interesting happened. In other words, that they saw an actual signal of something, rather than a random fluctuation. After all, the Universe is full of objects that emit gamma rays and X-rays, and that’s what the background is made of. But was it related to the gravitational merger of these two black holes?
If it were, you’d expect other satellites to see it. The best we can conclude, so far, is that if black holes do have an electromagnetic counterpart, it’s one that’s:
- incredibly weak,
- that occurs mostly at lower energies,
- that doesn’t have a bright optical or radio or gamma-ray component,
- and that occurs with an offset to the actual emission of gravitational waves.
Also, everything we see is perfectly consistent — and arguably, more consistent — with the notion that merging black holes don’t have any electromagnetic counterparts at all. But the truth about it all is that we don’t have sufficient data to decide just yet. With more gravitational wave detectors, more black hole mergers of high masses, better pinpointing of the location, and better all-sky coverage of transient events, we just might find out the answer to this. If the missions and observatories proposed to collect this data are successfully built, operated, and (where necessary) launched, then 15 years from now, we can expect to actually know the scientific answer for certain.
Ethan Siegel is the author of Beyond the Galaxy and Treknology. You can pre-order his third book, currently in development: the Encyclopaedia Cosmologica.