Why we’ll never shoot Earth’s garbage into the Sun
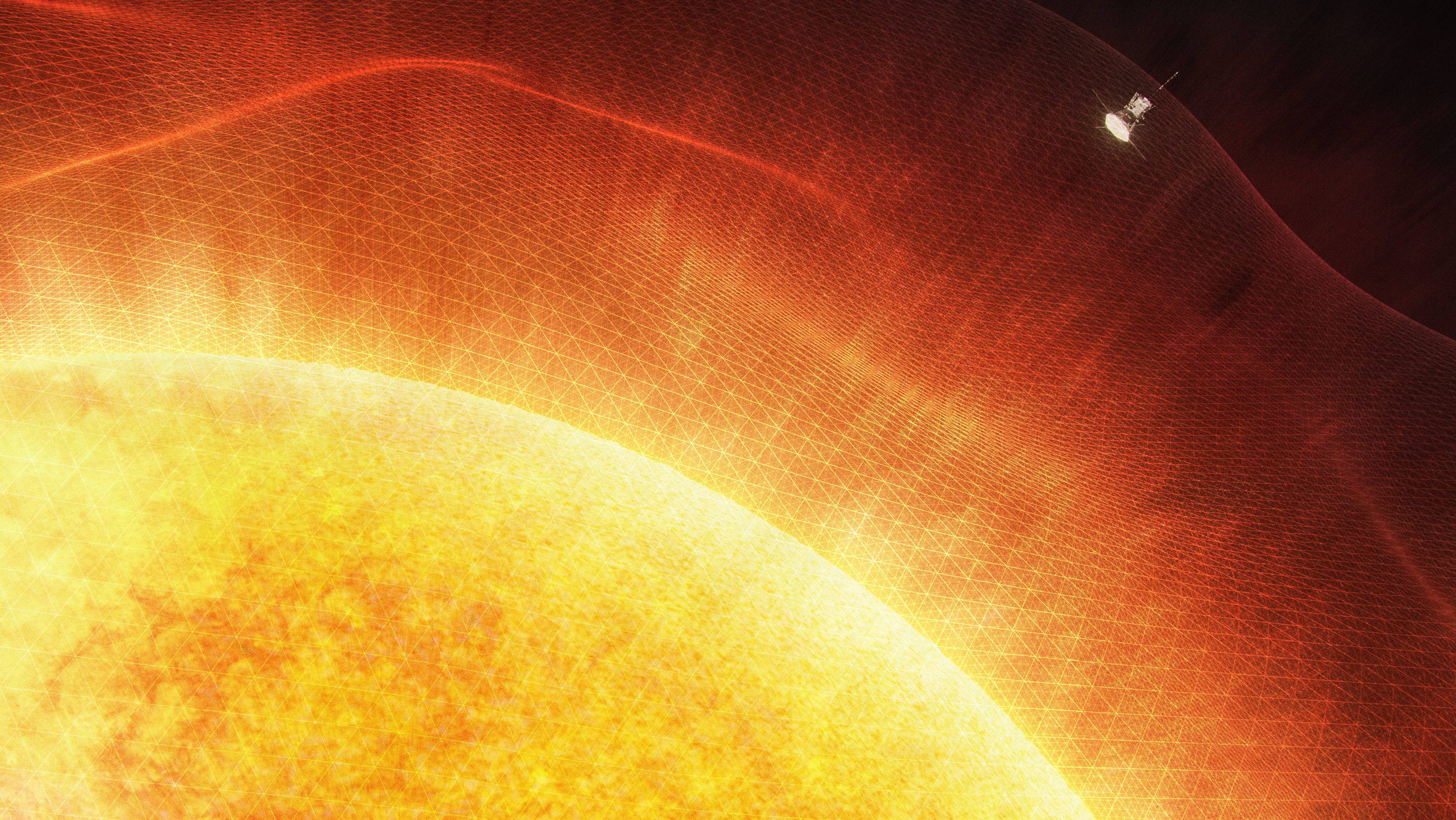
- With more humans than ever (over 8 billion) on Earth, the amount of waste we cumulatively produce on an annual basis continues to rise and rise: a problem that’s getting worse with time.
- Much of that waste is hazardous, some of it is radioactive, and all of it needs to be kept out of food and water supplies. Environmentally, there’s no 100% safe place to put it.
- Periodically, people suggest that we launch our waste into the Sun, destroying it forever while keeping Earth clean and pristine. But this is something we must never do, and physics explains why.
For billions of years on planet Earth, the greatest threats to any and all forms of biological activity came from predators, competitors, and the hazards of our natural environment. If you encountered an organism that wanted to fight, kill, or eat you, you had to figure out how to defend yourself, escape, or somehow survive. If you encountered a living or non-living threat to your essential resources like food, water, or sunlight, you’d have to protect and preserve the nutrients you need for your survival. And if you encountered any one of a set of natural disasters:
- fires,
- volcanoes,
- earthquakes,
- tsunamis,
- asteroid strikes,
- hurricanes,
- and many more,
you’d have to either escape those disasters or ensure that other populations of your species escaped them in order for your genetic line to continue to survive and thrive.
Whenever environmental changes were extreme and global, rather than the more common gradual and/or isolated, mass extinctions occurred. For the first time in our planet’s history, extreme, global changes are happening rapidly, and it’s not caused by any natural disaster at all, but instead by human activity. In addition to deforestation, habitat loss, war, and climate change, there’s another problem that the ever-growing human population brings along: waste management.
How can we deal with our most hazardous waste, including biohazards, deadly chemicals, and radioactivity? The answer isn’t to shoot it into the Sun, despite what many have proposed. Here are the physical reasons why.
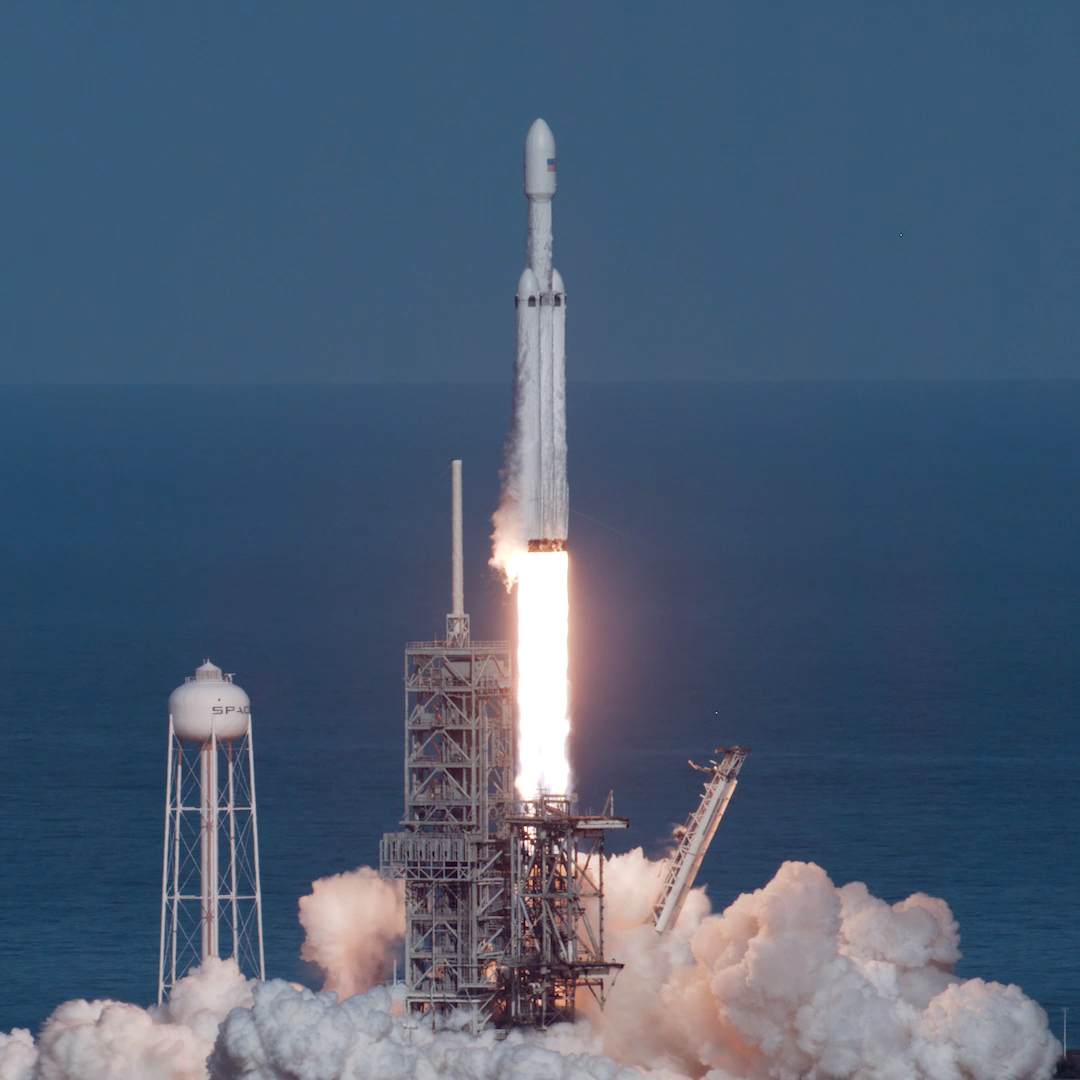
At present, here in 2024, there are more than 8 billion human beings on planet Earth. Since the mid-20th century, our rocketry technologies have advanced to the point where we’re now capable of breaking the gravitational bonds that had previously kept all Earthly inhabitants shackled to our world. We’ve extracted rare and valuable minerals from the Earth’s surface and interior, we’ve synthesized novel chemical compounds and even new atomic elements and isotopes, we’ve developed nuclear fission and fusion, and in many ways have produced a variety of devices and technologies that far surpass even the wildest dreams of our ancestors.
It’s no exaggeration to say that the quality of life of most people on the planet, here in the 21st century, is far beyond what the quality of life was for all but the richest of royals and nobility throughout the majority of human history. However, despite how positively these technological advances have transformed our world, there are indisputably a host of negative side effects that have come along for the ride. These include:
- environmental damage,
- deforestation,
- atmospheric pollution,
- ocean acidification,
- runoff into rivers, lakes, and other stores of fresh water,
- nuclear waste,
and many more. Although Earth can self-regulate these problems away in the long-term, these are all problems that human activity continues to exacerbate. On human timescales, these problems are ours to deal with, or to ignore at our (and our civilization’s) own peril.
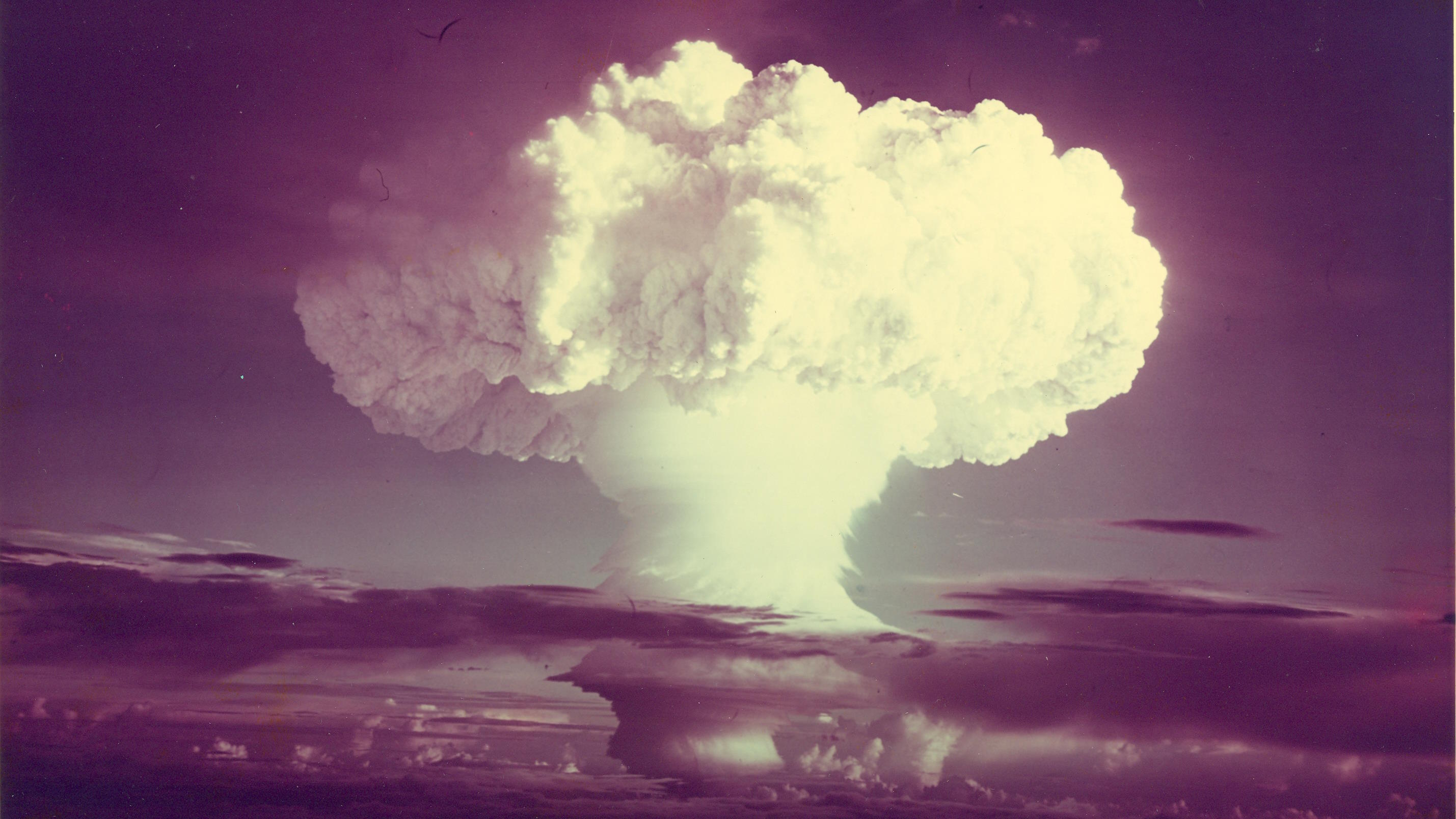
In addition to the foreseeable short-term problems that these types of pollution can cause, many of these problems will pose a long-term danger to the human population as well. Over centuries, millennia, or even longer timescales, our most dangerous pollutants include:
- nuclear waste and by-products of nuclear reactions,
- hazard chemicals,
- biohazards,
- and plastics that off-gas and don’t biodegrade.
If any of these types of pollutants affected the environment in the wrong way, including if they got into the drinking water supplies, into the food chain or human food supplies, or were incinerated and then breathed in by human beings, the havoc they could wreak could lead to millions or even billions of casualties.
How should we eliminate the worst of these offensive waste products? Some have suggested burying them in a geological subduction zone, where plate tectonics would eventually carry them into Earth’s mantle. Others recommend encasing them in concrete and allowing them to gradually degrade. Still others propose burying them in a geologically stable region, where they shall remain until future humans are ready to deal with them. All of these proposed solutions have pros and cons, but are worthy of consideration.
However, what we mustn’t do is the frequently suggested:
- pack them onto a rocket,
- launch them into space,
- and set on a collision course with the Sun,
where they won’t plague Earth ever again. (Yes, if you were mining the depths of your memory, this was indeed the plot of Superman IV.)

Sure, from a physics point of view, such an endeavor is possible. We could launch a rocket off of Earth’s surface, and we could then change that rocket’s velocity — either through providing thrust, through gravitational encounters, or a combination of both — so that it would collide with the Sun, achieving our goal. But just because something is physically possible doesn’t mean it’s a good idea, a good use of resources, or even a smart, useful solution to a problem. We can evaluate this notion by considering how gravitation works: on Earth, in our Solar System, and with respect to the garbage-containing rocket so many have dreamed about launching.
It was first realized by Newton that the same force that causes objects to fall “downward” on Earth, toward its center, is the same force that keeps the Moon (and any other satellite) orbiting the Earth: the force of gravity. Although we came to understand the basics of how gravity worked all the way back in the 17th century, it wasn’t until the 20th century that we developed the technologies necessary to break the gravitational bonds of Earth, and actually escape the influence of Earth’s gravity.
The gravitational pull exerted by our massive planet is only dependent on our distance from Earth’s center, which causes spacetime to curve and causes all objects on or near it — including humans — to constantly accelerate “downward,” or toward the Earth’s center. The farther away we are from Earth’s center, as our distance r from it increases, the weaker the gravitational force from the Earth becomes, decreasing as ~1/r².
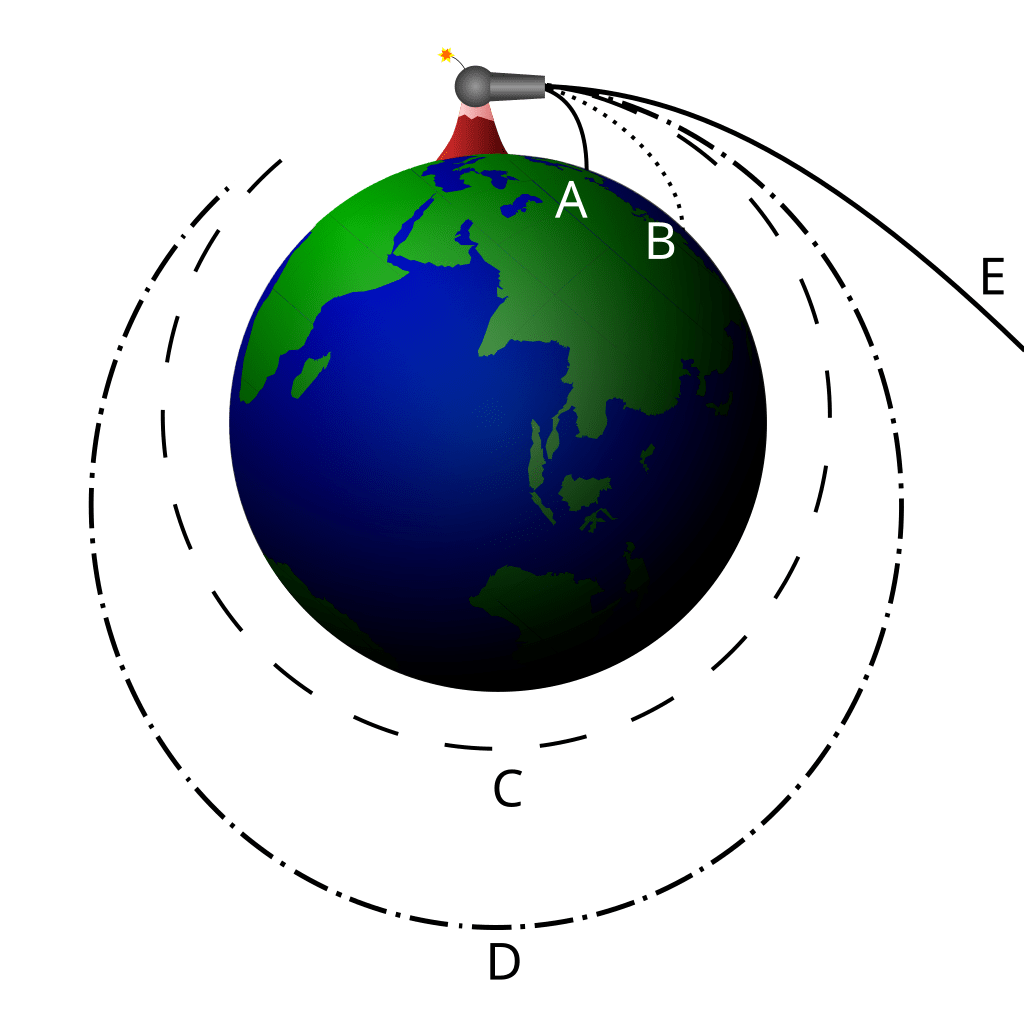
Although Newton wasn’t exactly well-versed with the quantitative notion of energy, we recognize today how important energy considerations are for any type of rocketry-driven endeavor. Here on Earth, there’s a certain amount of energy that keeps a massive object bound to it: gravitational potential energy. If the kinetic energy, or the energy of motion, that an object possesses exceeds its gravitational potential energy, we conclude that it can escape from Earth. In particular, in spaceflight, there are two thresholds that are worth keeping in mind.
- The threshold of a stable orbital speed, which keeps an object in orbit around Earth from ever colliding with our planet, which turns out to be about 7.9 km/s, or ~17,700 mph.
- The threshold of escape velocity, which is how fast an object needs to travel to escape from the bonds of Earth’s gravity: 11.2 km/s, or ~25,000 mph.
A human at the equator on Earth’s surface, where our planet’s rotation is maximized, moves at only 0.47 km/s (~1000 mph), which is why most rocket launches occur at near-equatorial latitudes: to maximize the “boost” we get from the spinning Earth. To get a rocket into Earth’s orbit (or to escape from Earth’s gravity entirely), we require at least the amount of energy needed to boost it to those speeds mentioned just above. Humanity first accomplished this in the 1950s and 1960s. Once we leave the Earth, we can easily see that so much more that’s of interest is occurring on larger scales.
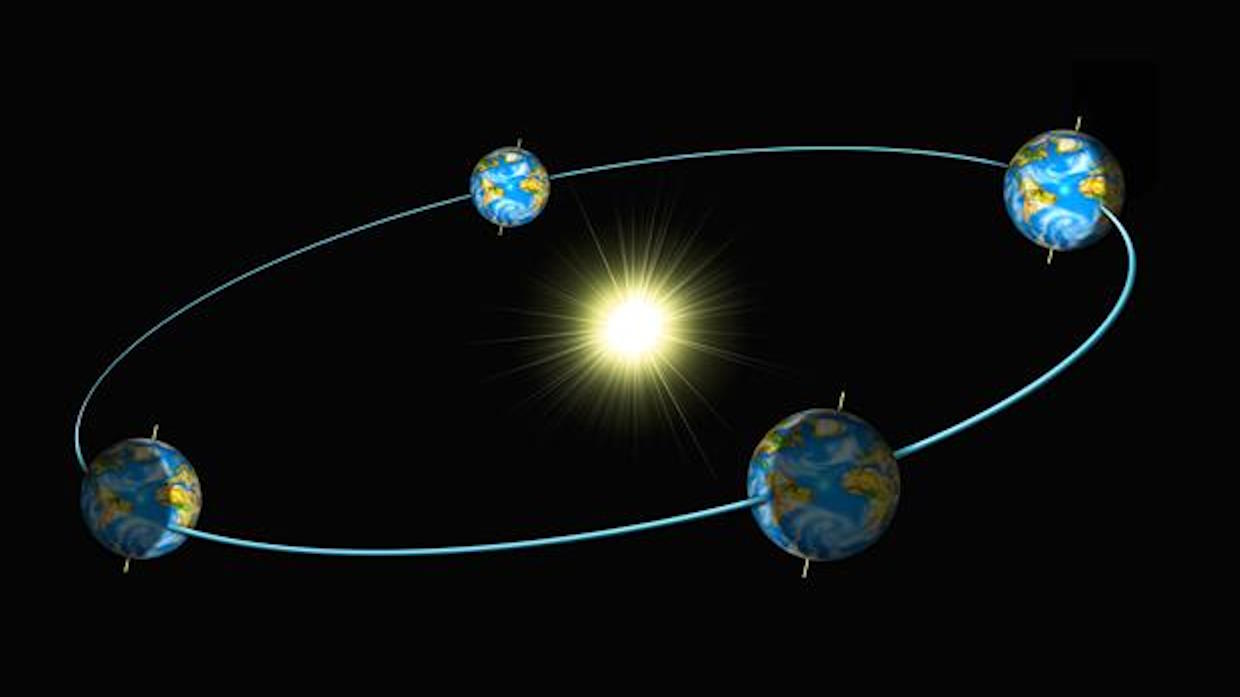
Planet Earth, for example, isn’t stationary in space, but rather orbits the Sun at an extremely fast speed: 30 km/s, or around 67,000 mph. Even if you escaped from Earth’s gravity — itself no small task — you’d find yourself gravitationally bound to the Sun, moving in a stable, elliptical orbit around it: an orbit that was determined by whatever speed you were moving at with respect to the Earth that you just gravitationally escaped from.
This surprises most people when they first learn about it. You might have thought, from our perspective here on Earth, that Earth’s gravity was the dominant factor affecting us here in the Solar System. That’s not true! Instead, the gravitational pull of the Sun far exceeds the gravitational pull of the Earth. The reason we don’t feel that is because the Sun pulls on us with the exact same acceleration that it pulls on the Earth; we’re in “free fall” around the Sun, and as a result, are all accelerated by it at the same relative rate.
If we were in space and managed to escape from Earth’s gravity, we’d still find ourselves moving at approximately 30 km/s with respect to the Sun, and at an approximate distance of 150 million km (93 million miles) from our parent star. If we wanted to escape from the Solar System, we’d have to gain about another 12 km/s of speed to reach escape velocity, something that only five of the spacecraft ever launched by humanity — Pioneer 10 and 11, Voyager 1 and 2, and New Horizons — have ever achieved.
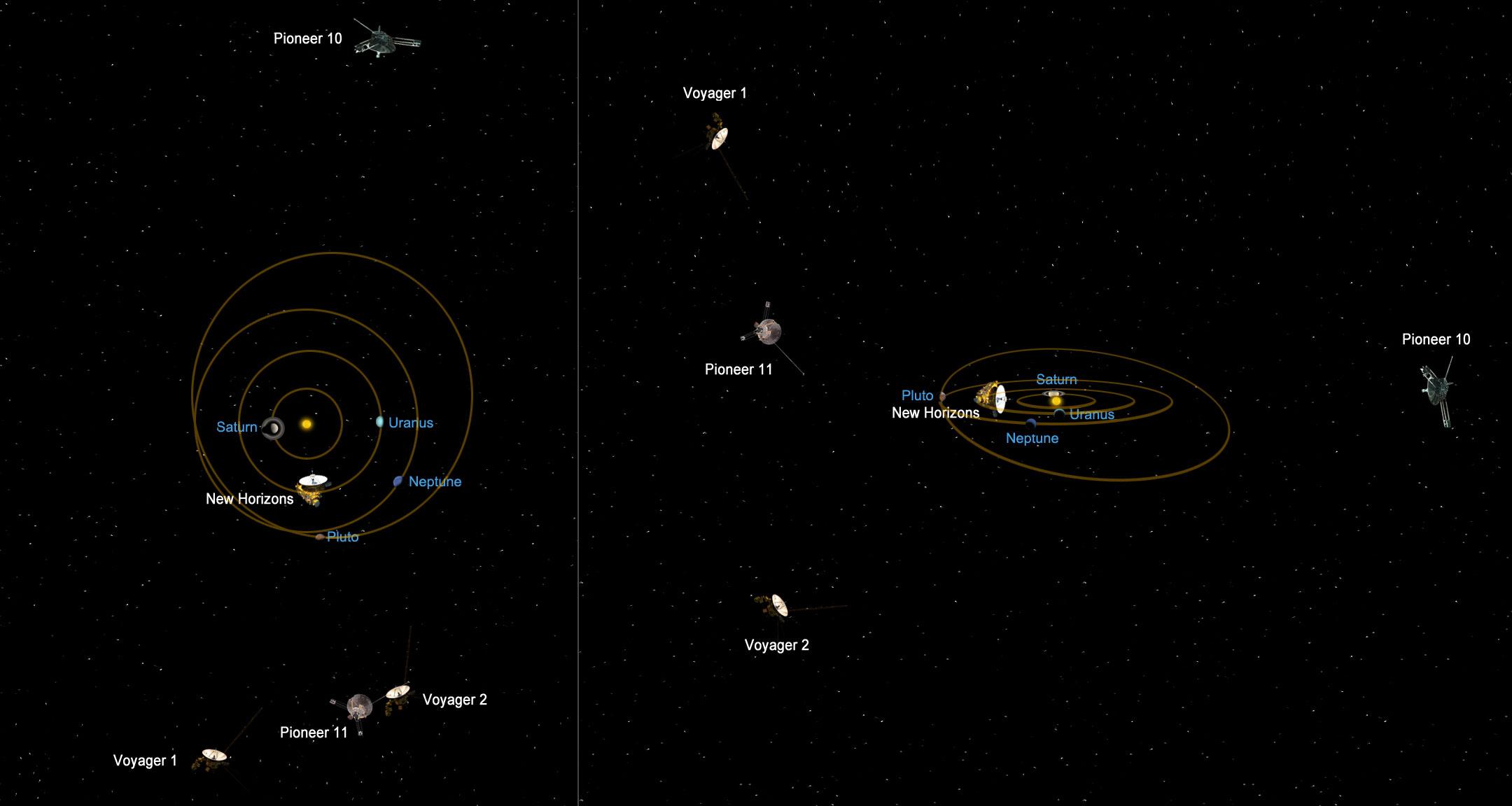
That’s a tall order. It takes an enormous amount of energy to get a payload up above the Earth’s atmosphere and into a stable orbit around our planet. It then takes even more energy (and thrust) to escape from Earth’s gravity, and to make it into interplanetary space. We’d then need to increase our kinetic energy by a very large amount — adding ~12 km/s (or 27,000 mph) to our speed at the distance of Earth from the Sun — in order to escape from the Solar System. Historically, this has never been accomplished entirely by a fueled rocket launch, but only from leveraging the gravitational slingshot effect of at least one, and normally several, other planets in our Solar System.
That’s to eject an object from the Solar System. What if we wanted to go in the opposite direction, and launch a spacecraft payload into the Sun? It turns out that’s an even more challenging problem, as we’d have to lose so much kinetic energy that what was previously a stable, elliptical orbit around the Sun would be transformed into an orbit that came close enough to the Sun to actually collide with the Sun’s surface. The only time in history this has ever come close to being accomplished was with the launch of the Parker Solar Probe, which underwent a large number of successive gravitational encounters with first Earth and then Venus, all stealing kinetic energy from the spacecraft, until it became the closest spacecraft in history in terms of close proximity to the Sun.
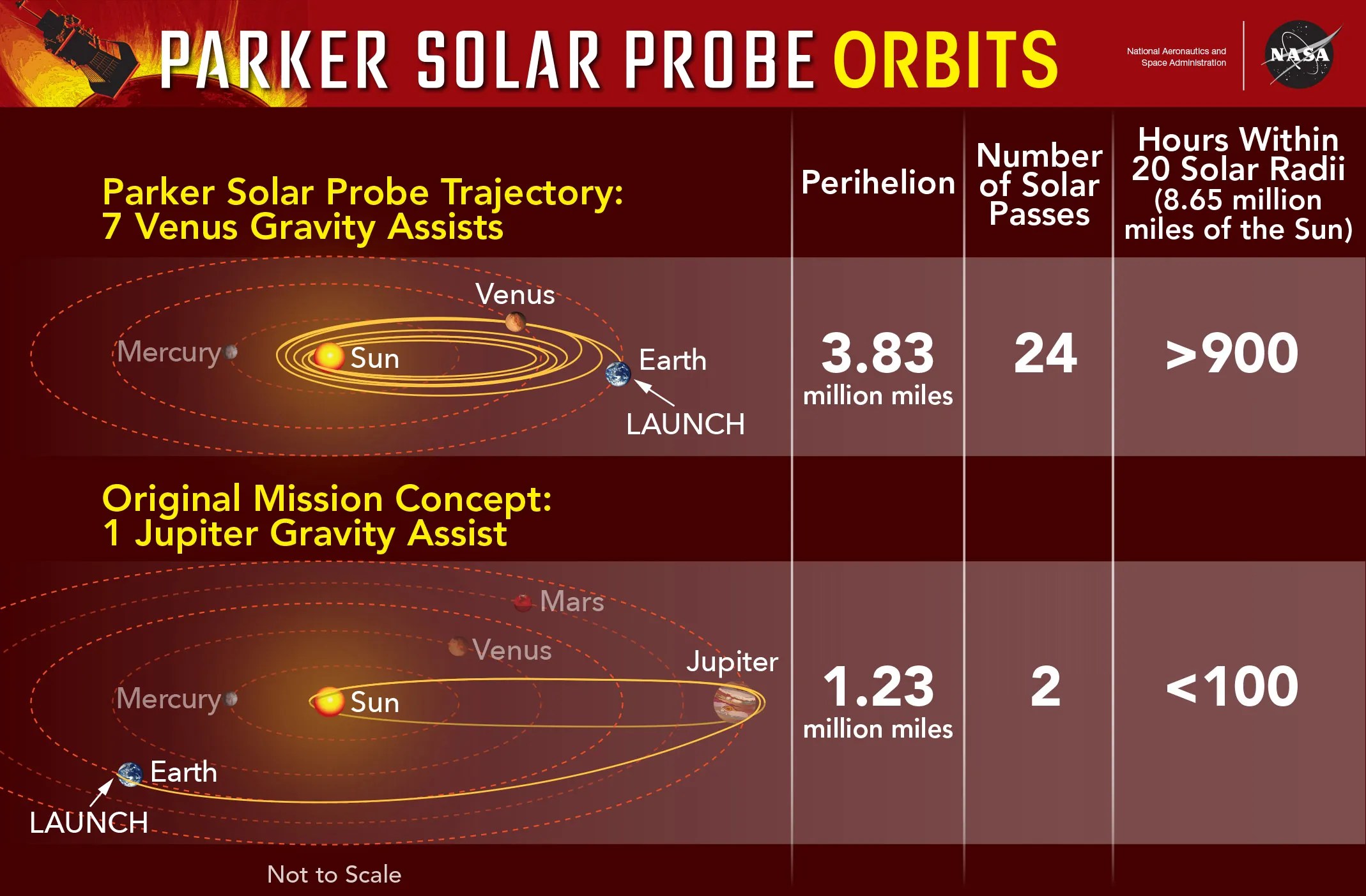
This took an enormous amount of effort and planning to achieve, and was a far superior option to the “obvious” solution of bringing enough fuel with you to decelerate your payload sufficiently. You might think to yourself, “Oh, I’ll just bring enough fuel on board, with my payload, to have it lose as much speed as is necessary, relative to the Sun, so that it just falls right onto it.” If you attempt that, it turns out that approximately 99% of your payload needs to be rocket fuel, and just the remaining 1% — which must include the rocket and the waste you want to launch into the Sun — would actually be effectively hurtled into the Sun. That’s a prohibitively large ratio of fuel-to-payload; it’s simply too inefficient of a process.
Configuring a launch to result in a series of gravitational encounters with the innermost three planets of the Solar System, Mercury, Venus, and Earth, requires an enormous amount of gradual de-boosting, eventually leading to a close enough encounter with the Sun so that it will inevitably be swallowed. The fact that there are only these “low mass” planets, rather than the higher mass worlds like Jupiter, Saturn, Uranus, and Neptune, means that it is a much more challenging problem to launch something into the Sun as opposed to launching something out of the Solar System entirely. From an energy perspective, it’s far from the ideal solution to a purely gravitational problem, although at least it’s possible, depending on the orientation of your spacecraft’s approach, to either boost (adding speed) or de-boost (subtracting speed) from a gravitational encounter between a spacecraft and a massive planet orbiting the Sun.
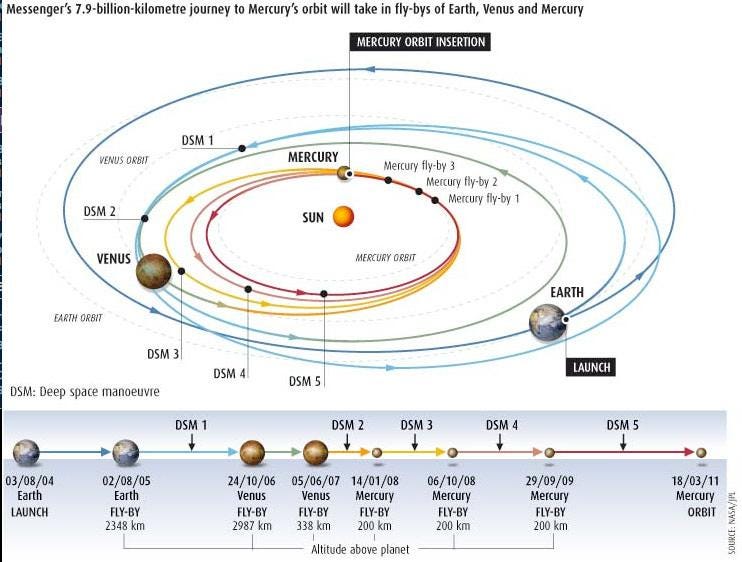
It was a full two decades ago, back in 2004, that we first successfully used the gravitational slingshot method to send a spacecraft into a stable orbit around our Solar System’s innermost planet: Mercury. This was NASA’s Messenger mission, and it enabled humanity to construct our first all-planet mosaic image of Mercury itself: one of the major science goals of the mission. Of course, it took a total of:
- seven years,
- six gravity assists (one with Earth, two with Venus, and three with Mercury),
- as well as five additional deep-space maneuvers (firing of the thrusters),
for Messenger to wind up successfully in orbit around Mercury.
More recently, that same technique has been used to launch the Parker Solar Probe into a highly elliptical orbit that takes it to within just a few solar radii of the Sun at closest approach. A carefully calculated set of future trajectories is all that’s required to reach the Sun, so long as you orient your payload with the correct initial velocity. It’s difficult to do, but not impossible, and the Parker Solar Probe is perhaps the poster child for how we would, from Earth, successfully launch a rocket payload into the Sun.
As much fun as this is to consider, however, there are three barriers to the idea of launching our garbage, even the worst of it, into the Sun.
- Launch failures are still a real possibility; more than 1% of even the most reliable launch vehicles (like the Ariane V rocket or the Soyuz spacecrafts) still fail. If you have a launch failure with hazardous or radioactive waste on board, that waste will be uncontrollably returned to the Earth, with potentially disastrous consequences.
- Energetically, it’s a much easier and safer task to launch your payload out of the Solar System than into the Sun; it’s choosing a harder problem over an easier one to accomplish the same task: ridding the Earth of its waste.
- And even with the recent advances of commercial and private spaceflight, the cost to lift our waste off of Earth’s surface and into orbit, alone, is prohibitively expensive for the sheer amount of hazardous and radioactive waste we already possess.

Considering that the United States alone is storing about 60,000 tons of high-level nuclear waste, it would take approximately 8,600 Soyuz rockets to remove this waste from the Earth. Even if our launch failure rate were reduced to an unprecedented 1-in-1000, we’d still anticipate dozens of tons of hazardous waste winding up in our oceans and over our planet’s continents: an unacceptable catastrophe for our environment.
It might be an attractive solution to dream about shooting our hazardous waste into the Sun, but until launches without failure become routine and cost-effective, we cannot seriously consider it. Even when they do, we’ll greatly prefer a solution that ejects our waste into interstellar space, rather than into the Sun itself. While there’s no denying that we’ve collectively made a mess here on planet Earth, the solution to our problems simply isn’t to shoot all the messy bits into the Sun.