Ask Ethan: Do JWST’s results contradict the Big Bang?
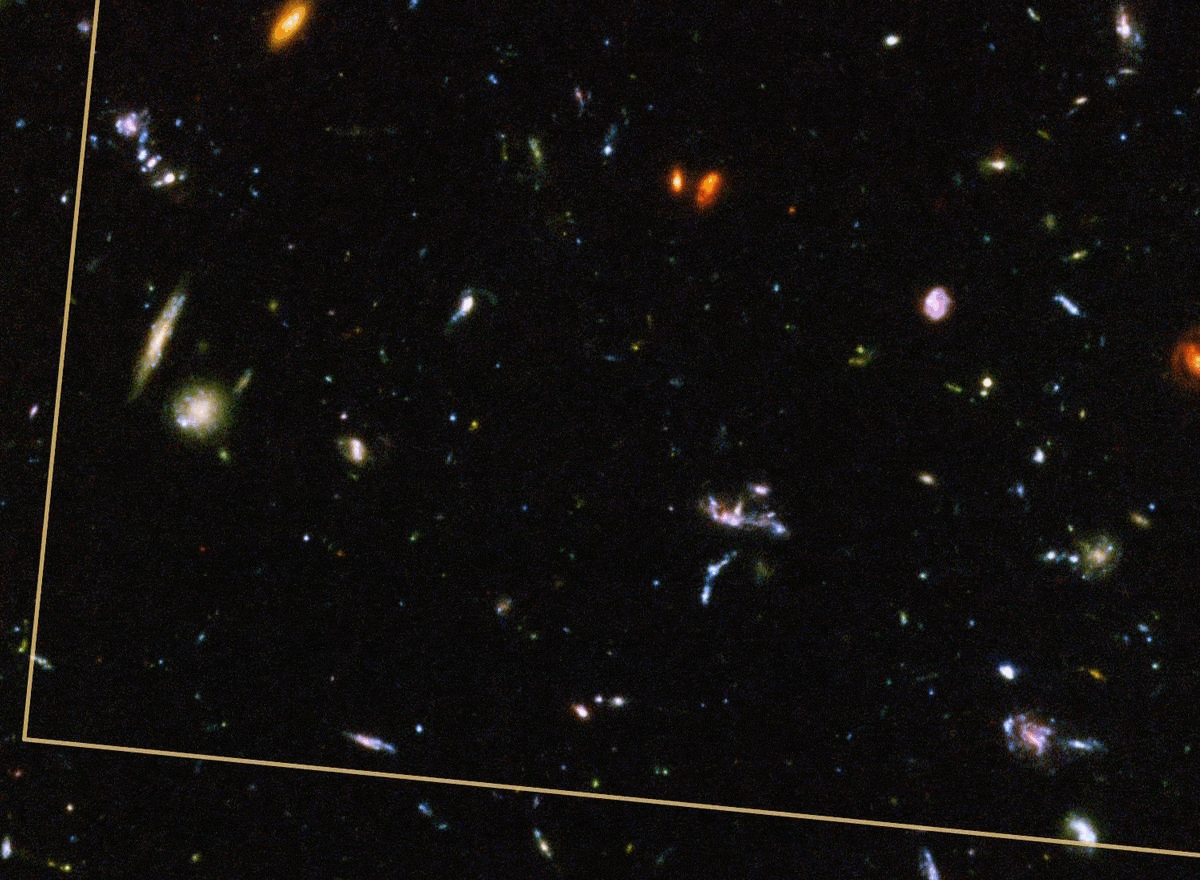
- When JWST first opened its eyes and peered into the deep Universe, what it found was quite surprising: lots of young, massive, evolved-looking galaxies.
- According to our standard model of cosmology — with dark matter, dark energy, normal matter and radiation — these types of galaxies would require substantial amounts of time to form, grow, and evolve.
- With all of these galaxies found between 300-400 million years after the Big Bang, is modern cosmology (and, by association, the Big Bang) in trouble? Results are mixed.
When it comes to the science of cosmology — the history of the Universe and how it came to be the way it is today — one of the crowning achievements of the past 100 years is the development of a “standard model” of cosmology. The dominant factor in determining how the Universe evolves is gravitation, which is governed by General Relativity and accounts for the expanding Universe as well as the assembly of large-scale cosmic structure. The contents of the Universe have been determined to be dark energy, dark matter, normal matter, neutrinos, and photons. And the Universe as we know it began some 13.8 billion years ago with an event known as the hot Big Bang, with density imperfections seeded by a preceding phase known as cosmic inflation.
Despite all the observational evidence we have supporting this picture, it may not be fully correct. Each time we observe the Universe in a new way, we have to check that what we’re seeing is still consistent with this model. With the recent addition of JWST to the arsenal of tools astronomers have, is this picture in trouble? That’s what many, including Patreon supporter Chad Marler, want to know:
“The newest fad [among armchair physicists] is that the JWST observations of galaxies that are more mature than expected in far reaches of the universe ‘disproves’ the Big Bang. I’m not sure there has been enough time or data accrued to actually make a real account of the results yet, but I sure haven’t heard anyone with any credentials say that, either.”
Certainly, a lot of extraordinary claims have been made, but what’s the full truth? Here’s the current status.

The first thing we have to do is lay out, based on our picture of the Universe, how we expect events to unfold in our Universe. This picture — sometimes called the standard model of cosmology, sometimes called the inflationary hot Big Bang, and sometimes called ΛCDM (because of dark energy, i.e., Λ, and cold dark matter) — has been remarkably successful, explaining features ranging from:
- the internal motions of individual galaxies,
- the motions of galaxies relative to one another,
- the motions of galaxies within groups and clusters of galaxies,
- weak and strong gravitational lensing on all cosmic scales,
- the structure and growth of the cosmic web,
- and the features found in the Big Bang’s leftover glow: the cosmic microwave background.
It also predicts that, as we look farther and farther back in time — i.e., to greater and greater cosmic distances — that the galaxies we see will be inherently smaller, bluer, less evolved, less rich in heavy elements, and that at some point beyond where we’ve been able to look, we should cease to see stars or galaxies of any type, as we’ll reach the Universe’s “dark ages.”

But that’s simply a picture of what happens. What we need, if we want to compare theory to observations, is to quantitatively figure out not just what happens, but when it happens and, quantitatively, how much it happens by. Even though the laws of physics are well-known, and the “starting point,” or our initial conditions, are also well-known, our best quantitative predictions still come along with a large amount of uncertainty.
From the theory of cosmic inflation and the patterns of fluctuations that we see in the cosmic microwave background, we know that our Universe began, at the start of the hot Big Bang, from an almost-perfectly uniform state. There were the seeds of structure — density imperfections — imprinted atop that near-uniform background, leading to underdensities and overdensities at about the 1-part-in-30,000 level, that were almost but-not-quite the same on all cosmic scales: about 3% larger on size-of-the-Universe scales than on size-of-a-galaxy scales.
We know that early on, these imperfections grew gravitationally, but also had to contend with interactions with and pressure from radiation, like photons, creating a pattern of peaks-and-valleys in how overdense/underdense various regions were on a variety of cosmic scales.
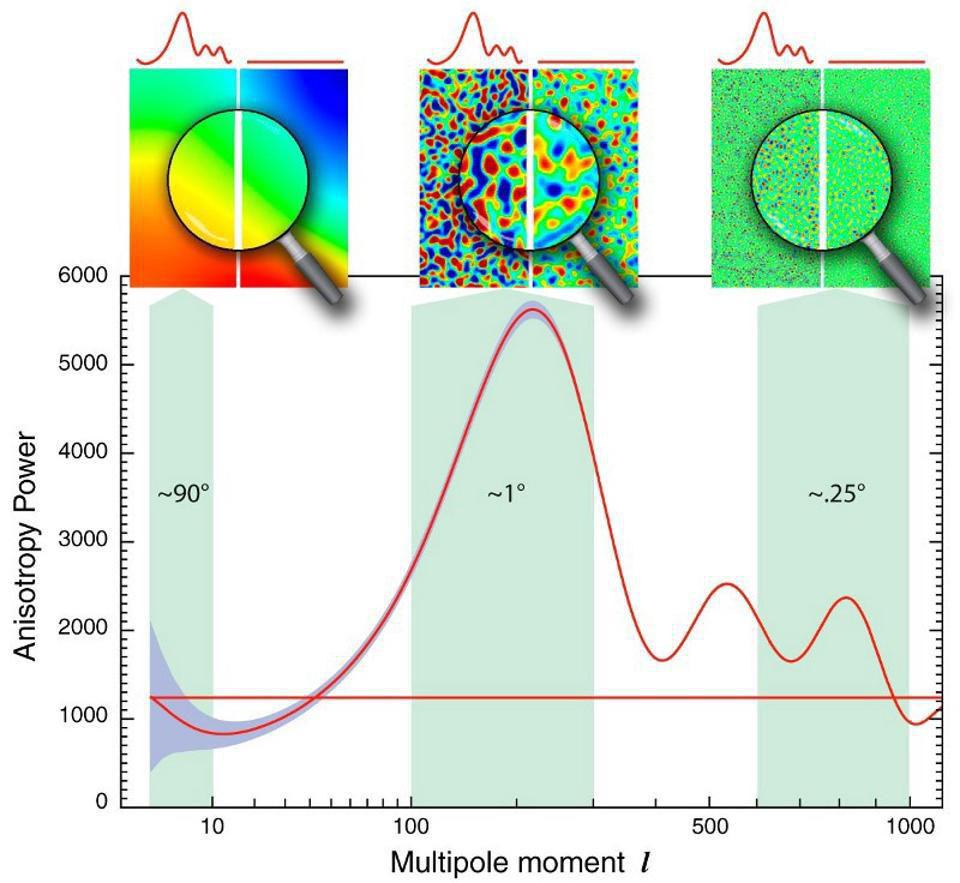
Then, the Universe forms neutral atoms (about 380,000 years after the hot Big Bang) and expands, cools, and gravitates according to the laws of General Relativity. As long as these density imperfections remain small compared to the average density of the Universe, it’s easy and straightforward to compute how they grow.
But as they grow larger, a series of effects all come into play, making the question of “How big do they grow, and how quickly?” very assumption-dependent. For example:
- As large amounts of gas begin to accrue in these overdense regions, how efficiently does that gas cool?
- As these overdense regions grow within the expanding Universe, with some small-scale regions superimposed atop larger-scale overdensities, how do these high-density regions interact in these overlapping locations?
- Some overdense regions will occur close to other overdense regions; how is the growth of structure affected when these regions interact?
- As normal matter accumulates in the centers of these overdense regions, it slows down, collides, and heats up. As that heat gets radiated away, how does that feedback affect the growth rate of these regions, including both the normal matter and the dark matter?
- And, finally, when stars finally form in these very different environments to the ones we find today, how long do they live, how do they die, how does that impact the normal and dark matter that doesn’t become stars, and what implications does that have for subsequent generations of stars and the growth of these early cosmic structures?
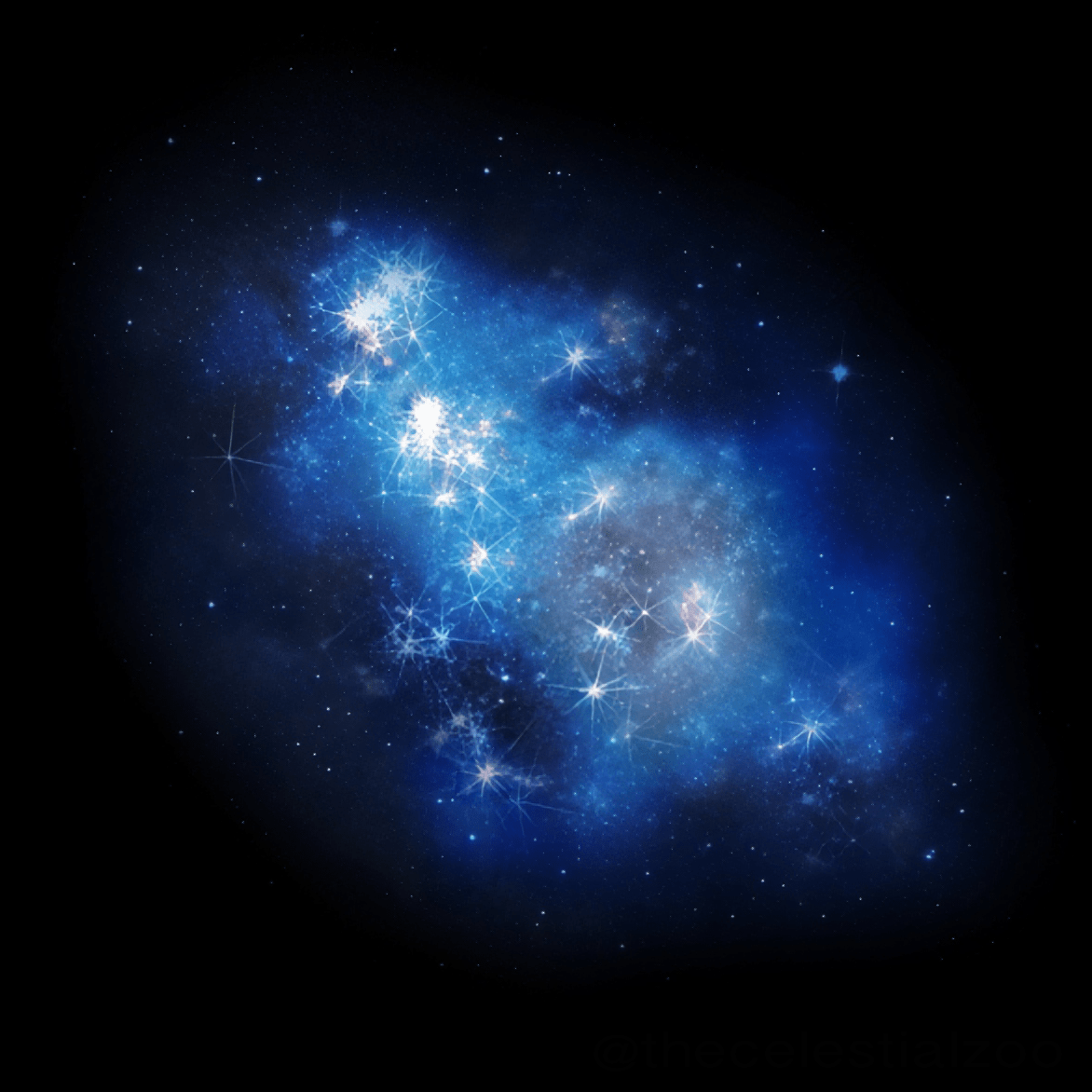
It’s important to understand that the answers to all of these questions are uncertain: they’re firmly in the realm of the purely theoretical and are dependent on what details we include and exclude in our models and simulations. Are we using the correct models to identify haloes, where a “halo” represents an individual overdensity in space, or are we incorrectly treating interdependent haloes as independent entities or vice versa? Are we modeling the first stars correctly, including their initial mass functions and their death throes, or are they heavier and more likely to directly collapse to black holes than we think?
For that matter, do we even need stars to form black holes, or can these intersecting, inflowing streams of gas form the seeds of supermassive black holes directly, possibly with masses that are 10,000+ times the mass of our Sun right away?
It’s pretty clear that the very first objects — stars, black holes, and star clusters — begin forming no later than about ~150 million years after the Big Bang and perhaps as early as only 50-100 million years after the Big Bang, but these ought to be relatively rare occurrences. How many big, bright, evolved galaxies we should see at slightly later times, like 200, 300, or 400 million years after the Big Bang, is a much murkier question.
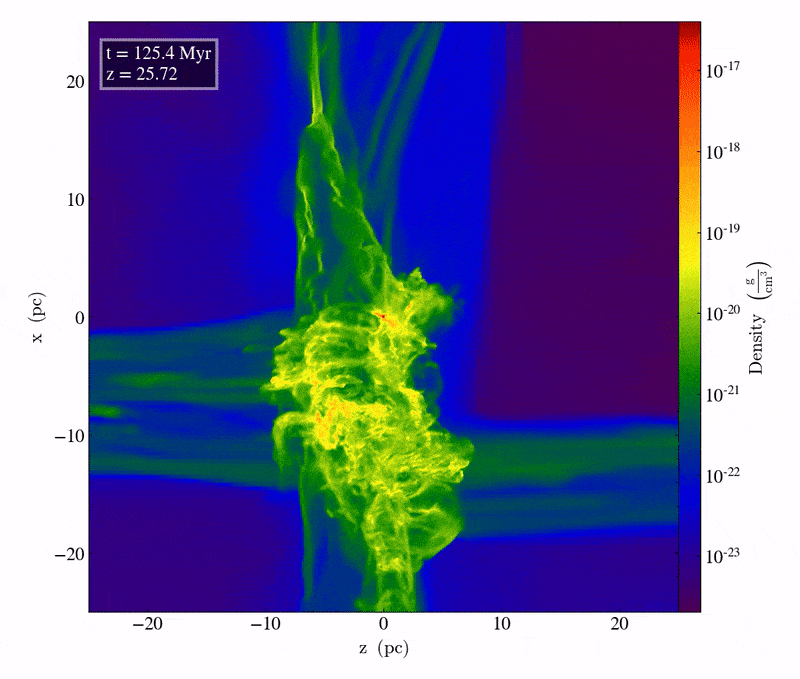
But we don’t simply have our theoretical expectations, even with the appropriate uncertainties, to work off of. For the first time, owing to the unprecedented capabilities of the James Webb Space Telescope (JWST), we’re beginning to discover and characterize galaxies found in these very, very early stages of our cosmic history. Prior to JWST, we had one confirmed galaxy (GN-z11) at an age of about 400 million years after the Big Bang, and only one other galaxy candidate (unconfirmed), possibly at an age of around 330 million years after the Big Bang. No other stellar or galactic signals from such early epochs had ever been seen.
Fast forward to the present day, less than one year after JWST began science operations, and the story has changed dramatically. We have over 100 galaxy candidates from JWST that take us back to the first 400 million years of the Universe, and several of them have already been confirmed to indeed be ultra-early and ultra-distant. GN-z11, already, is now only the fifth most distant galaxy known, and it’s fully expected that over the next 12 months, not only will the current cosmic record for distance (JADES-GS-z13-0, from a time just 320 million years after the Big Bang) be broken, but that at least dozens of new ultra-early, ultra-distant galaxies will soon be confirmed.

Many of these early galaxies that JWST is finding have peculiar, puzzling properties about them that appear difficult to reconcile with this theoretical picture that the Universe has painted for us. They appear, for example, to be:
- very massive,
- very bright,
- very rich in heavy elements,
- very actively forming new stars,
- and very rich in gas.
The fact that we see so many galaxies with these properties so early on is puzzling, because we believe we understand how gas — i.e., atom-based matter — infalls onto these early galaxies, and how star-formation feeds back and prevents future gas from falling in. There’s a limit for how fast material can accrete onto these objects, and although certain physical conditions can lead to an object temporarily overcoming that limit, it shouldn’t be sustainable over such long timescales.
Therefore, when we look at these very early galaxies, we do get the impression that something is amiss.
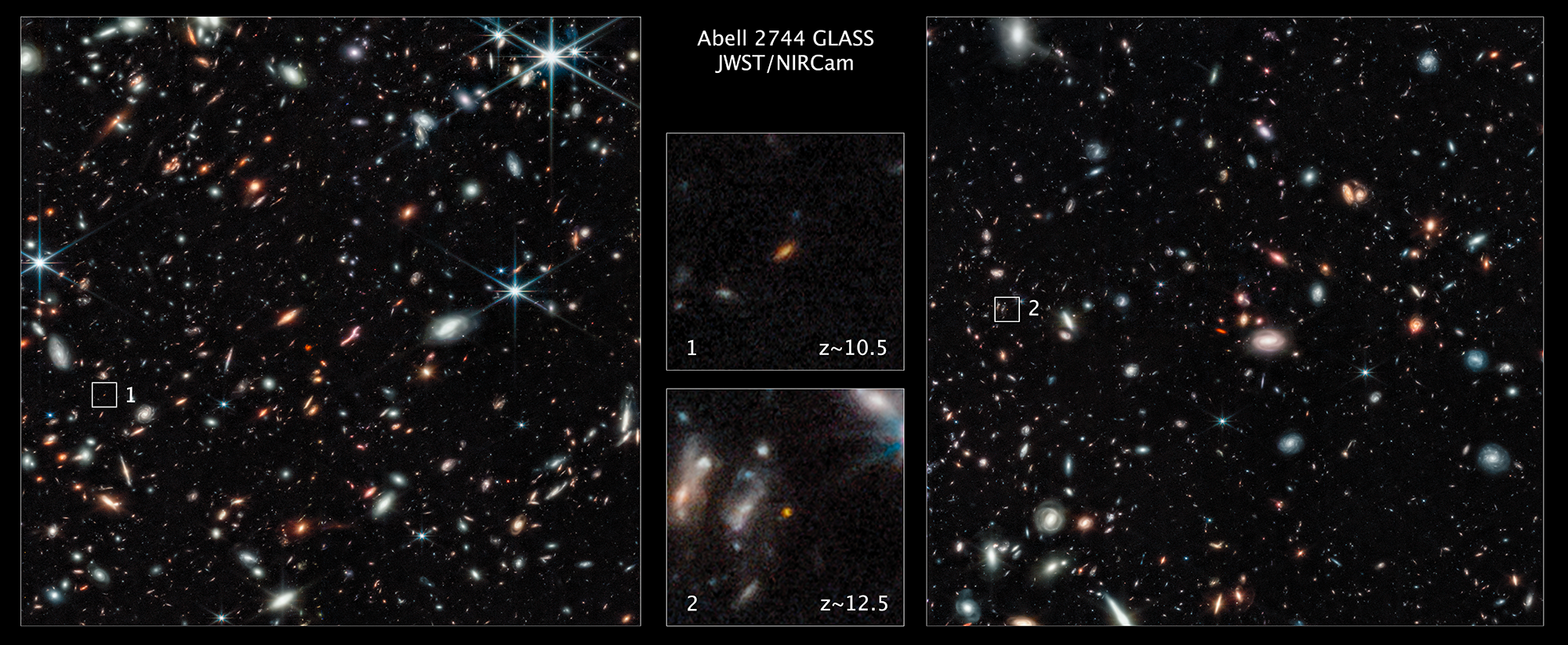
But what is it, exactly, that’s amiss?
A lot of people immediately resort to the spectacular or fantastical as far as explanations go. They assert that all of standard cosmology might be wrong, and perhaps we should throw out the Big Bang as the explanation for the origin of our Universe as well. They hypothesize new laws of physics (like a new, early form of dark energy that existed early on) or new physical phenomena (like the Universe being born with supermassive black holes) or new exotic forms of matter (like a novel, long-lived particle that decays into normal matter at relatively late cosmic times).
But that shouldn’t be your first resort, as a scientist. That should be your last resort: after you’ve exhausted all of the mundane explanations. And there are a number of things that are quite mundane to consider. We should first make sure that these effects aren’t playing a major role in causing these galaxies to appear with the properties they appear to have, and we should also make sure that our expectations for how the Universe ought to behave are in line with the way the Universe actually behaves.
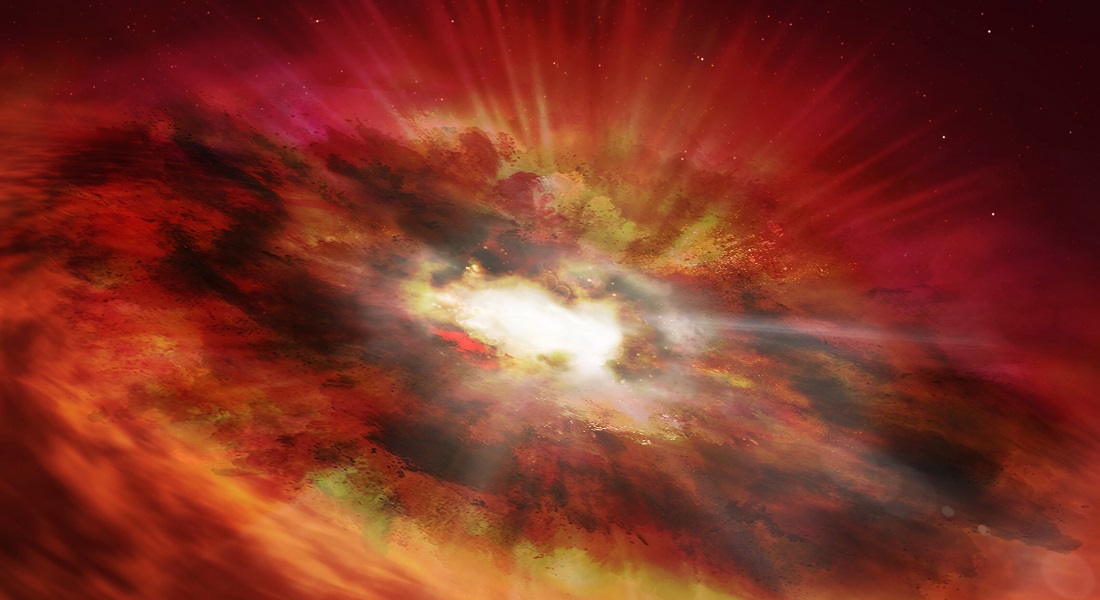
For one, the early surveys that are pointing to these conflicts are coming from very small, and possibly atypical, regions of the sky. There are surveys coming that will cover some ~50 times the area that are showing these early galaxies, and we might well see a “regression to the mean” of this apparent effect.
For another, it’s possible that light from an active supermassive black hole at the centers of these galaxies is “polluting” our view, and making us think that these galaxies are more massive and rich in stars than they actually are. A full spectroscopic analysis of these galaxies, not yet available, will be needed to determine if this is the case.
For yet another, it’s possible that these galaxies aren’t actually brighter and more massive than we expect — at least, not by the amount we’ve initially concluded — because JWST is overperforming. It could simply be the case, at least in part, that JWST’s better-than-expected eyes make these galaxies appear brighter than they will turn out to be when properly calibrated.
And finally, it’s possible that we’ve gotten some detail like gas cooling, halo identification, the nonlinear growth of structure, or the effects of stellar feedback or magnetic fields incorrect.
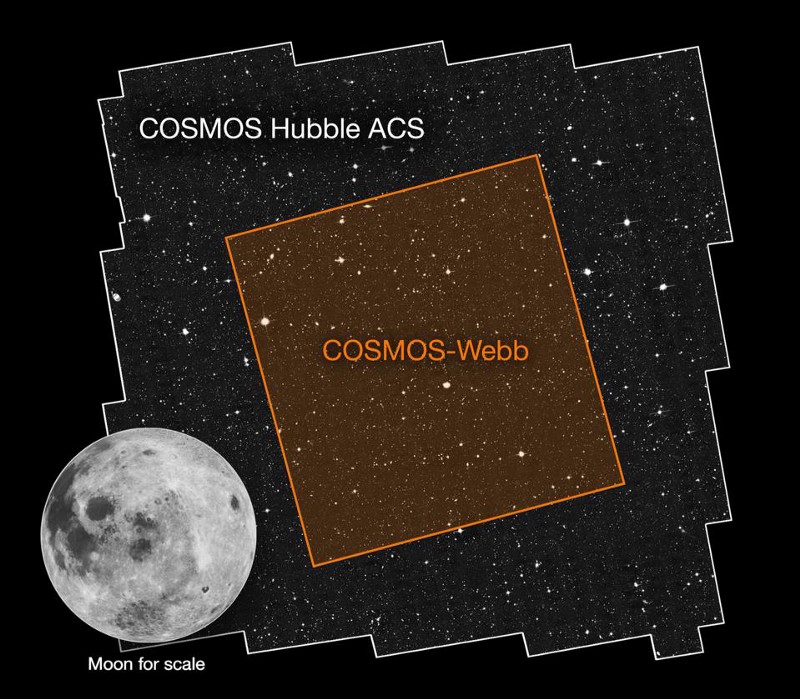
In other words, it’s possible that either the preliminary data is unreliable or that our assumptions for how the early stages of cosmic structure formation proceed are flawed. While there are some early observations that could wind up pointing to a tension between what JWST is seeing and what our current understanding of the laws and composition of the Universe are, any such assertions that “the Big Bang/ΛCDM/standard cosmology is in trouble” are definitely premature at this point. Without better data — i.e., a deep, large-area, robustly calibrated, spectroscopic survey — we don’t even know if these galaxies truly possess anomalous properties. An in-progress JWST survey, COSMOS-Web, should settle the issue.
And even if they do, there are an enormous number of astrophysical possibilities that invoke no fundamentally new physics that could potentially account for why these galaxies would exist with these large masses and brightnesses. The only thing that would truly shock us at this point, and to be clear, this is not what the data indicates at present, is if there’s more mass inside these early galaxies than the normal matter known to be in the Universe could potentially account for. It’s entirely possible, even if these early galaxies are as bright and massive as the most optimistic estimates are, that boring old gravitation, electromagnetism, and stellar/gas physics can explain what we see.
A lot of people are making a lot of early, extraordinary claims about these galaxies, but we have to keep in mind that sound, responsible science progresses slowly, and always follows the evidence. The important thing isn’t to be the first one to speculate as to what the ultimately right answer is, but to get it right without unjustifiably crying “wolf” along the way.
Send in your Ask Ethan questions to startswithabang at gmail dot com!