Yes, JWST caught a baby galaxy cluster being born!
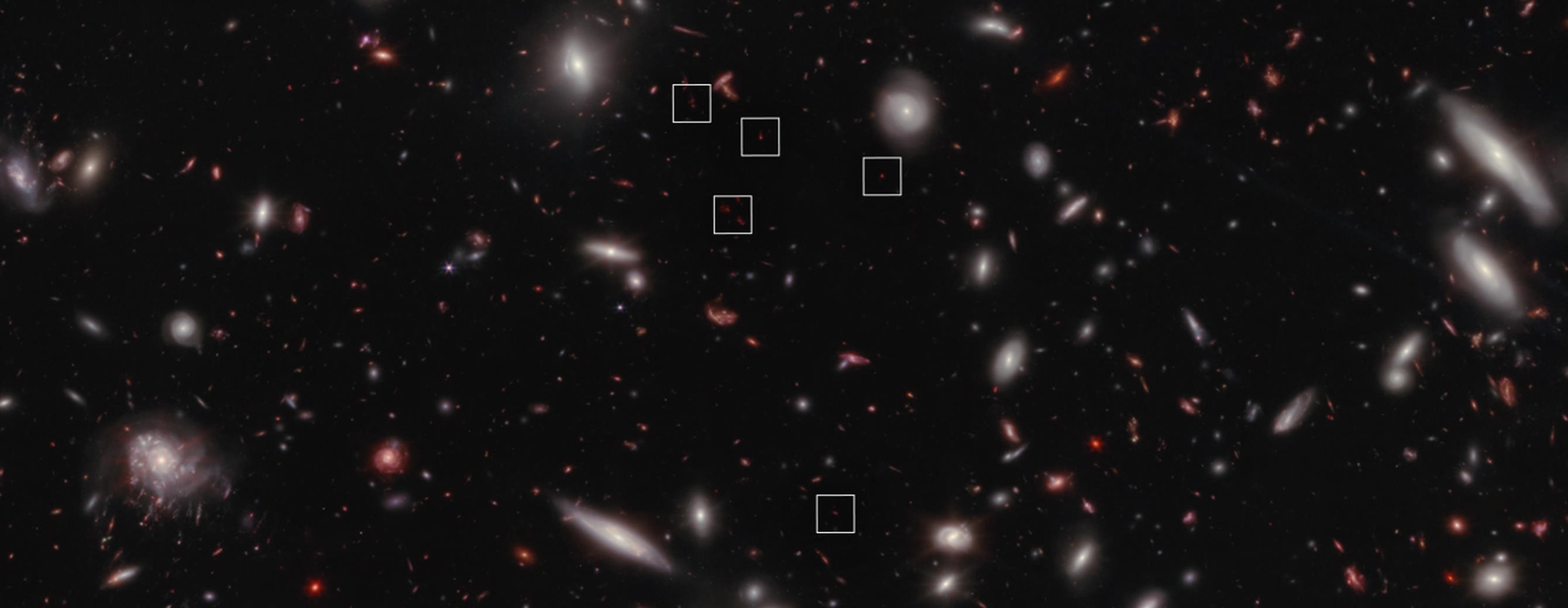
- With its large primary mirror, its distant location from Earth, its ultra-low temperatures and its infrared-optimized instruments, JWST is science’s greatest “time machine.”
- It’s already seen back farther into the early Universe, finding more distant stars and galaxies than ever before, than any observatory in history.
- For the first time, it just caught an ultra-distant, still-assembling galaxy cluster just 650 million years after the Big Bang. Here’s why it’s a triumph for modern cosmology.
How did the Universe grow up? This simple-seeming question was one that puzzled humanity endlessly for all of our civilization’s history: right up until the middle of the 20th century. It was at that point that the cosmic microwave background was discovered, where it was quickly linked with being the remnant glow of long-wavelength predicted by the Big Bang. Since that time, we’ve enhanced and refined our understanding, determining the age of our Universe (13.8 billion years) and what it’s made of at present (a mix of dark energy, dark matter, normal matter, neutrinos and photons).
This knowledge is enough to give us a set of expectations: how and when we think the Universe should have formed stars, galaxies, and even groups and clusters of galaxies, paving the way for our modern cosmic web to take shape. But the key specifics — of precisely how fast the Universe grew up on stellar, galactic, and super-galactic scales — remained beyond the reach of our greatest prior observatories, like Hubble.
But JWST is changing that aspect of the story, answering these questions for the very first time. With its latest discovery, it’s found the earliest proto-galaxy cluster ever discovered, just 650 million years after the Big Bang. Here’s what it teaches us.
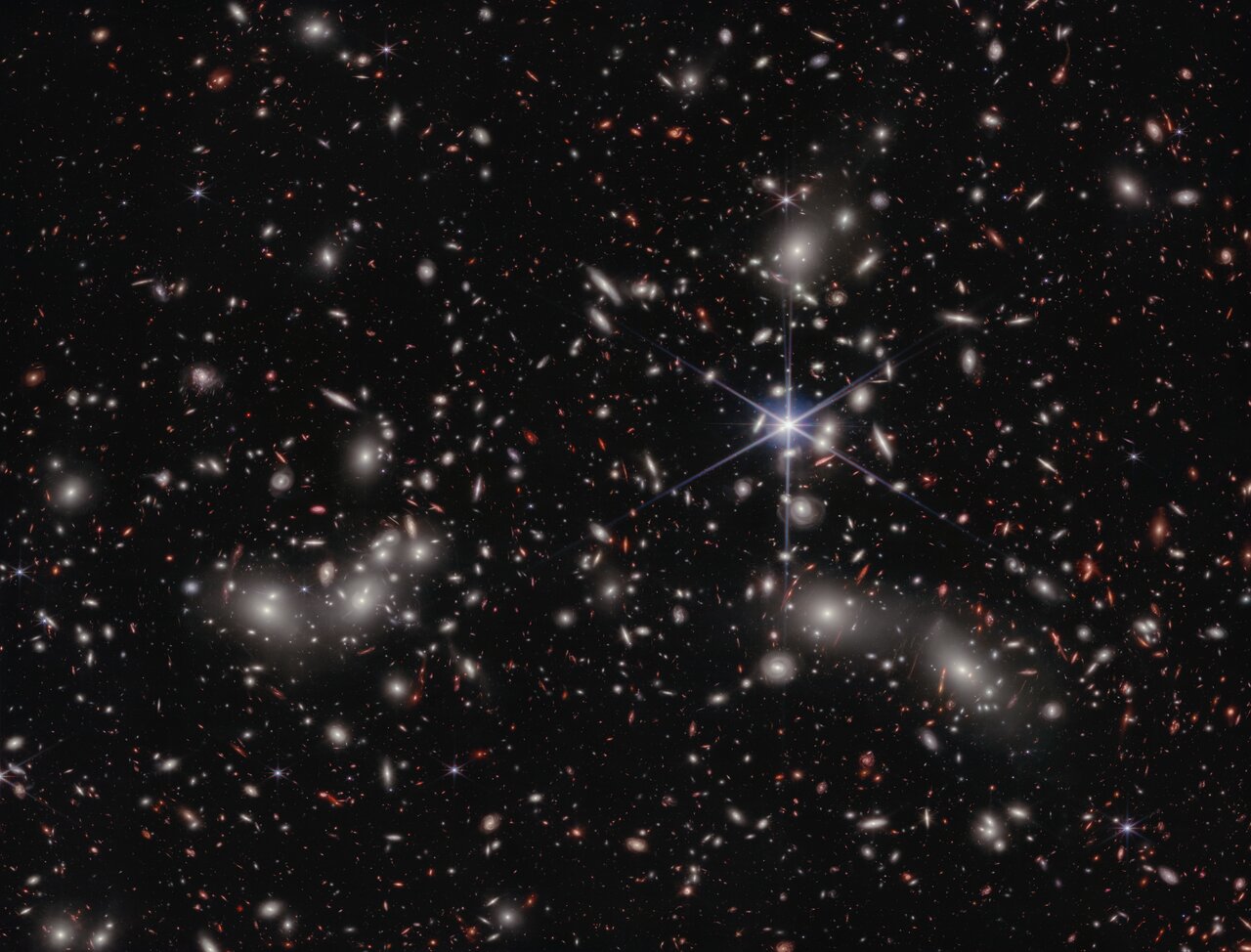
In theory, there’s a hierarchy to how things grow up in the Universe. In the very early stages of the hot Big Bang, the Universe was almost perfectly uniform: all of the matter-and-energy was distributed evenly throughout space, with tiny, 1-part-in-30,000 fluctuations superimposed atop that uniform background. These fluctuations were generated by cosmic inflation, which preceded and set up the Big Bang, and occur on all cosmic scales: small, intermediate, and large.
Because matter-and-radiation interact, and also because the Universe expands, the smallest-scale fluctuations get washed out, the intermediate scales experience peaks-and-valleys in whether the density fluctuations are enhanced or suppressed, and the largest cosmic scales are unaffected. This information gets encoded into the leftover glow from the Big Bang: the cosmic microwave background, where they’re observable even today.
Then, once neutral atoms form, the overdense regions begin to gravitationally grow, while the underdense regions give up their matter-and-energy to their denser surroundings. But gravitational growth, despite the fact that gravitation is an infinite-range force, doesn’t occur equally across the Universe.
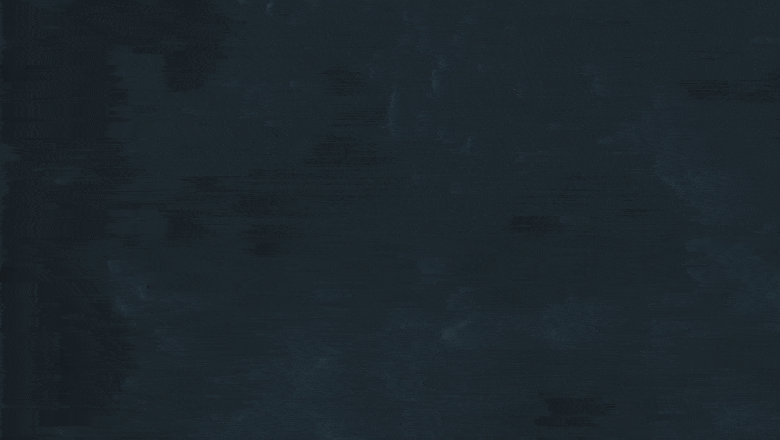
The key is to remember this: that gravity, like all signals in the Universe, doesn’t reach everywhere instantaneously, but rather is limited by the speed of light. If you have an overdense region located at one point in space, it can attract matter that’s nearby in a certain amount of time, but matter that’s ten times as distant will require at least ten times the amount of time (probably more, given that the Universe is expanding) to feel the gravitational attraction from the very same object. The larger and grander a cosmic scale is — from star clusters to galaxies to groups and clusters of galaxies and beyond — the more time it requires for gravitational attraction to initiate.
Then, once a larger-scale region begins feeling the effects of gravitational attraction, several events need to take place before a bound structure forms, all of which require time.
- The receding matter needs to slow down as it speeds away from the center of the gravitational overdensity.
- The overdense region needs to grow to a critical mass — about 68% above the average density — in order to trigger gravitational collapse.
- Then, the larger-scale structure needs to cease in its recession, reverse direction, and begin collapsing.
And finally, what we’ll wind up with is a bound object: with sub-components that are all part of some larger, bound, large-scale structure.
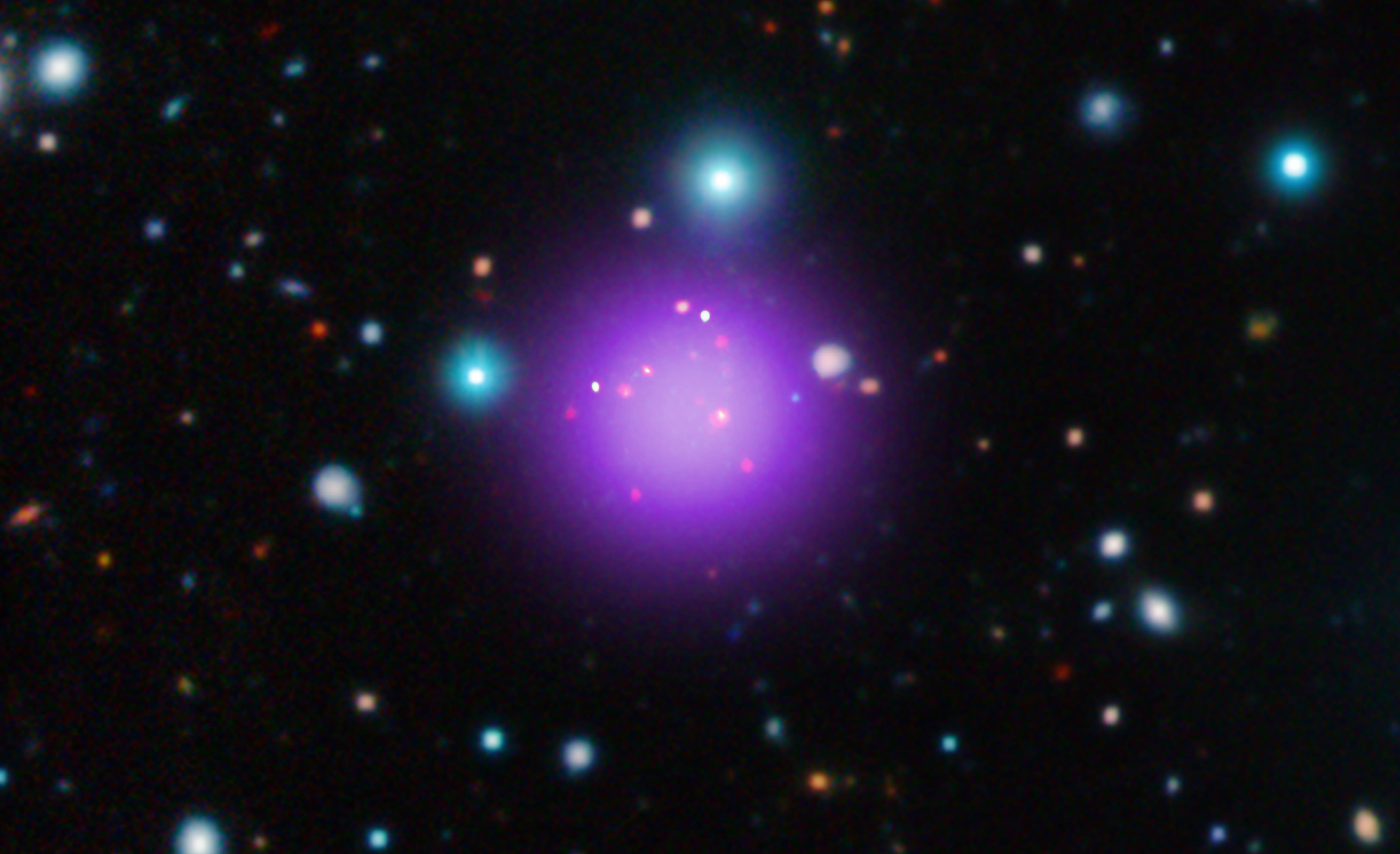
On the smaller end of the cosmic scale, molecular clouds of gas, dust, atoms, and dark matter become the first structures to collapse, eventually leading to the first stars and star clusters. While it may take around 200-250 million years for the most common of these overdense regions to collapse, the earliest ones to do so (i.e., the ones with the greatest initially overdense conditions) might be able to do so in just 50-100 million years. As stars form, they emit radiation and winds, and that creates unfathomably complicated environments, leading to great difficulties in predicting any sort of specifics about these early structures.
As these early clumps of matter draw more and more matter into them, they also find one another and merge together, building up the earliest massive galaxies within the Universe. At the limits of what JWST has seen so far, we’ve discovered richly evolved galaxies as early as ~320 million years after the Big Bang, with many of these early ones being massive, rich in heavy elements, and with copious amounts of ongoing star-formation. JWST was fully expected to discover these objects, and we still have every reason to hope that populations of absolutely pristine stars, as well as even earlier galaxies, will be revealed by JWST’s capabilities.

But on the larger end of the cosmic scale, this “messy physics” doesn’t play much of a role at all. Whereas, on the scale of individual galaxies, one must contend with:
- ongoing star-formation,
- winds and radiation from massive stars,
- stellar deaths and cataclysms,
- cooling and infall of gas and other atom-based matter,
- mergers and accretion,
- ionization,
- and the interplay of dark matter with normal matter,
these factors play only an extremely minor role when it comes to the formation of galaxy clusters.
Instead, the formation of galactic groups and galaxy clusters largely depends only on three factors, all of which are well-known.
- The expansion of the Universe, which is fully determined at all cosmic times once we know the contents of what’s actually in the Universe.
- The magnitude of the initial overdensity on the relevant cosmic scale, which enables us to calculate the rate of gravitational growth of any such object.
- And how that gravitational growth proceeds over time, including the relevant interplay of various cosmic scales.
All of the messy stuff that occurs inside an individual galaxy — sometimes disparagingly referred to as “gastrophysics” — has a negligible effect on the formation and growth of galaxy clusters; only gravitation matters.
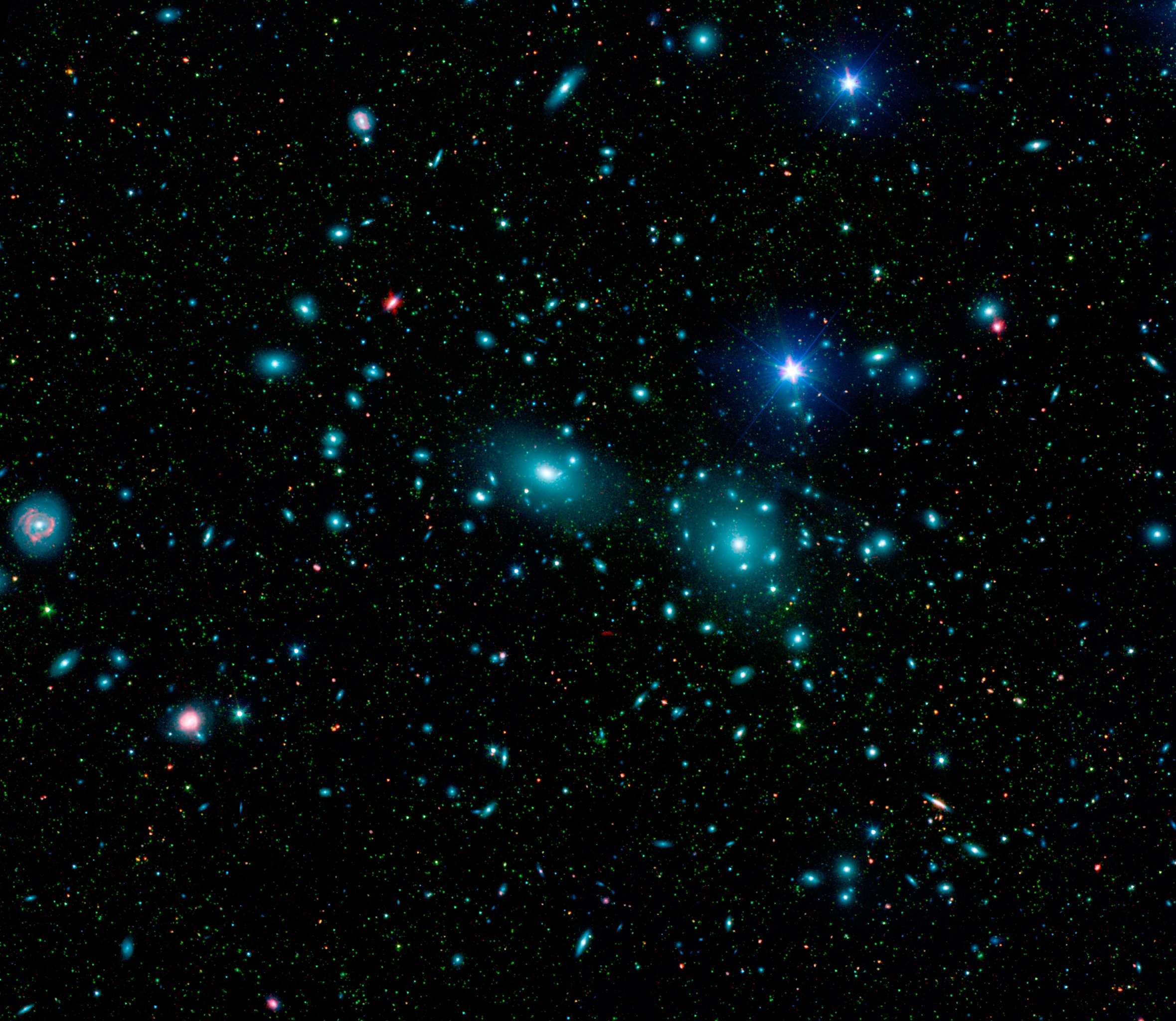
Prior to JWST, we’ve had a number of ways of revealing these galaxy clusters throughout cosmic history. The simplest and most straightforward was simply to identify large numbers of galaxies that existed within the same field-of-view, at identical redshifts/distances to one another, but with a significant velocity dispersion: where galaxies within the cluster moved at speeds of several hundred or even a few thousand km/s relative to one another. Nearby galaxy clusters, such as Coma and Virgo, were easy to identify this way.
Galaxy clusters that undergo heating, such as from the collision of fast-moving gas clouds or from intense star-formation events, emit X-rays throughout the intergalactic medium within the cluster, leaving an identifying signature if we probe them in the correct wavelengths of light. These X-ray emitting clusters aren’t just ways to identify clusters, but also provide vital information about their masses, gas contents, and merger histories.
And finally, galaxy clusters have also been revealed through the collective effects of their gravity: through the phenomenon of strong-and-weak gravitational lensing. Because it’s the cumulative amount of mass that exists along a particular line-of-sight, a massive galaxy cluster will be distinguishable from a set of unclustered galaxies because of the lensing features owing to the intracluster matter: the mass within the cluster that’s between the individual galaxies.
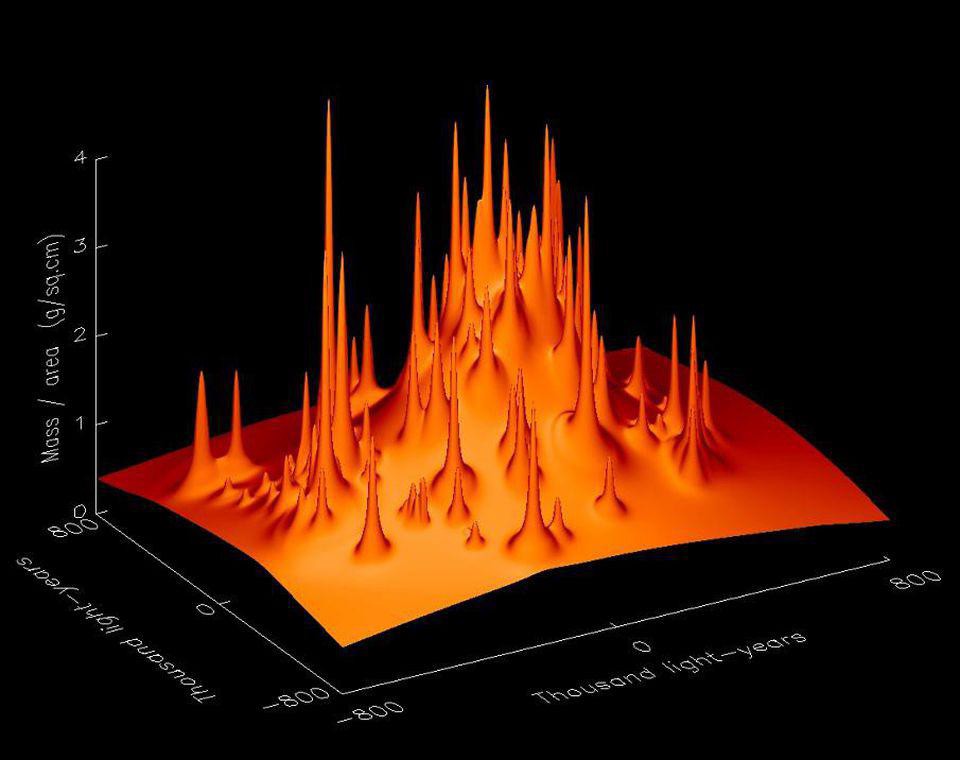
The oldest mature galaxy cluster is relatively close by: CL J1001+0220, which was discovered through its X-ray emissions and whose light comes to us from just 2.7 billion years after the Big Bang. With 17 identifiable galaxies within it, more than half of which are starburst galaxies (i.e., forming stars in a great burst that encompasses the entire galaxy). However, galaxy clusters aren’t born as “mature” objects, but evolve from an unformed state through a proto-cluster phase. Therefore, if we want to find the first such objects, we have to look for proto-clusters of galaxies: collections that haven’t yet heated up their gas to emit X-rays.
Just prior to the JWST era, a 2019 survey using our premiere ground-based observatories like Subaru, Keck, and Gemini revealed two very distant collections of several galaxies in the very early Universe: one consisting of 44 galaxies at a redshift of 5.7 (corresponding to an age of 1 billion years after the Big Bang) and another consisting of 12 galaxies at a redshift of 6.6, or an age of just 800 million years after the Big Bang. These proto-clusters were the earliest examples of collections of galaxies that occupied a similar vicinity in space, with their speeds and masses indicating that they’re definitely undergoing the process of becoming gravitationally bound, and may have already crossed that threshold.
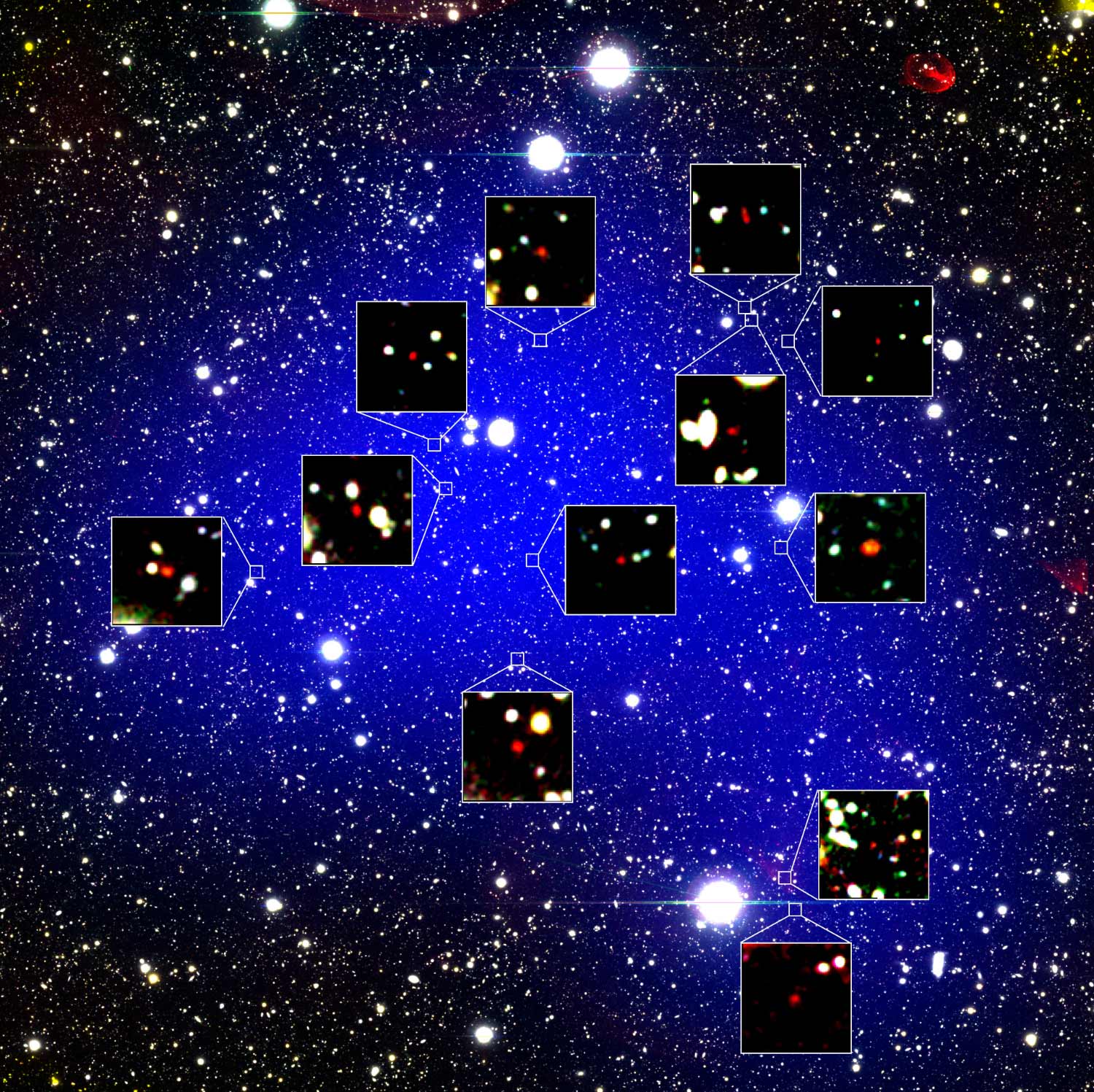
With JWST’s incredible cosmic vision, we fully expected that we’d someday break this cosmic record, pushing the earliest known cluster back to unprecedented times. However, it was also expected that this would take some time, as robustly identifying galaxy clusters typically requires two sets of observations to coexist. First, you need a wide-field photometric survey, one capable of covering a large enough area so that galaxy cluster candidates — that is, galaxies whose colors are consistent with them all being very distant and at the same distance — can be identified.
And then, you need the ability to perform spectroscopic follow-ups on those galaxy candidates, determining which ones are true galaxies and what their actual redshift/cosmic distances are. The only two proposals within JWST’s first year of science operations — which we’re still in even in April of 2023, by the way — are PANORAMIC and COSMOS-Web, neither of which has released their findings yet.
However, three other first-year surveys that covered smaller areas:
- JADES: the JWST Advanced Deep Extragalactic Survey,
- GLASS, which looked at the deeply lensed galaxy cluster Abell 2744,
- and CEERS, the Cosmic Evolution Early Release Science Survey,
have already published, with CEERS finding four galaxies in the same narrow area of sky at the same distant redshift of 4.9, corresponding to a proto-cluster just 1.2 billion years after the Big Bang.
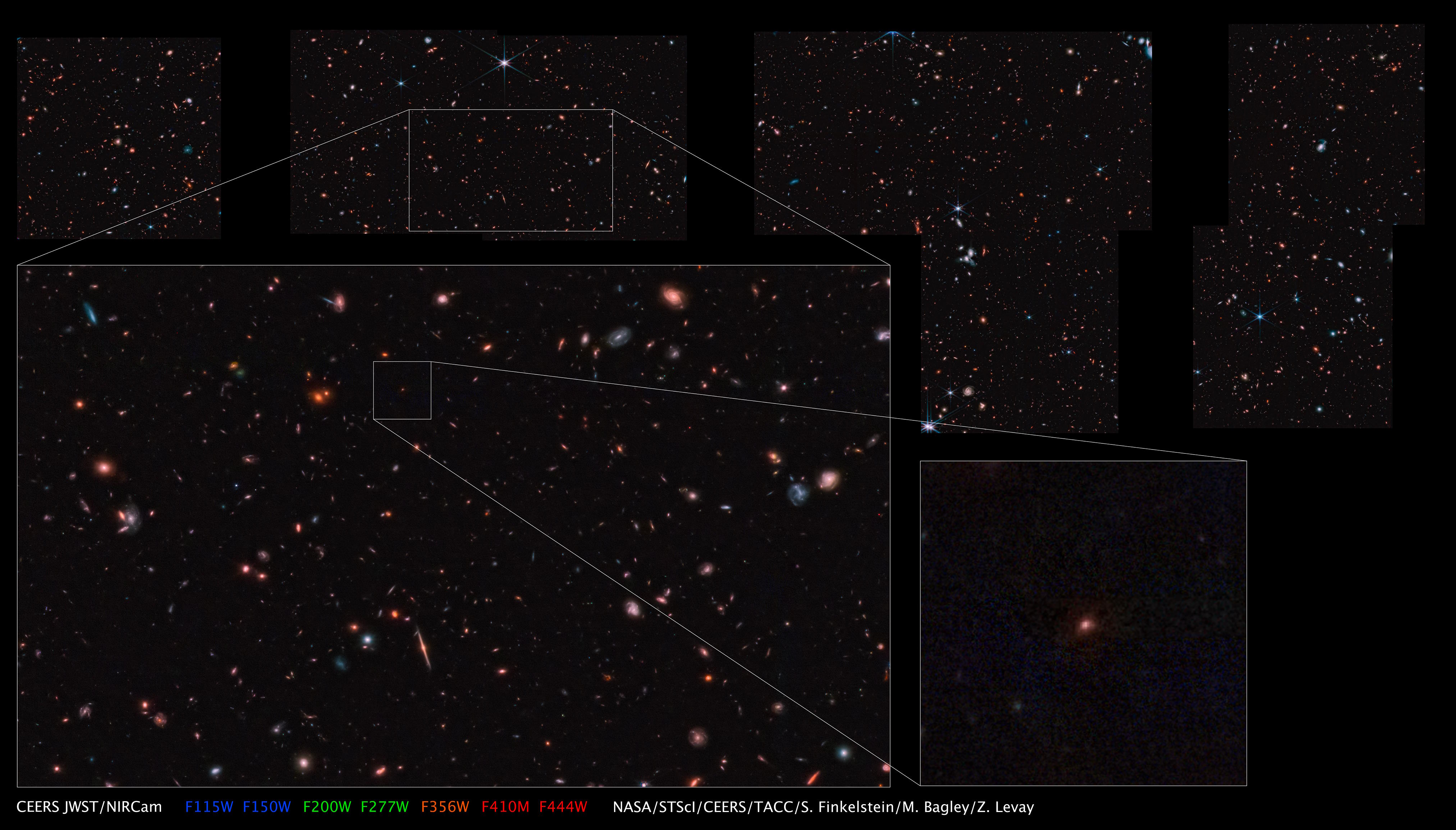
But within the GLASS field, where you have the additional effects of a magnifying foreground galaxy cluster (Abell 2744), the potential for going even deeper abounds. As luck would have it — and, as far as we can tell, it really is just luck — seven independent galaxies have been found in the same region and have been spectroscopically confirmed to be at the same redshift, 7.88, which corresponds to a time merely 650 million years after the Big Bang: the earliest proto-cluster of galaxies ever identified. The cluster’s name, at least for right now, is quite a mouthful: A2744z7p9OD, because:
- it was discovered in the lensing field of Abell 2744 (A2744),
- at a redshift of 7.88 (which rounds to 7.9, and hence the “z7p9” part of the name),
- and where its redshift was confirmed through the detection of doubly-ionized oxygen in each of the seven member galaxies (leaving it ambiguous whether the “OD” part is for “oxygen detection” or because this proto-cluster represents an “overdensity”).
This galaxy cluster was previously imaged with the Hubble Space Telescope, which revealed about 130 times the “average” number of galaxies within a very small region of space, which includes this now-identified proto-cluster. However, the most compelling galaxy candidate from the Hubble study was called YD4, which now turns out (with spectroscopy) to be at a redshift of 8.38, meaning it’s not a part of this proto-cluster, but rather a still more distant background object. Of the eight galaxies highlighted in the inset image (below), it’s the only one to not be a cluster member.
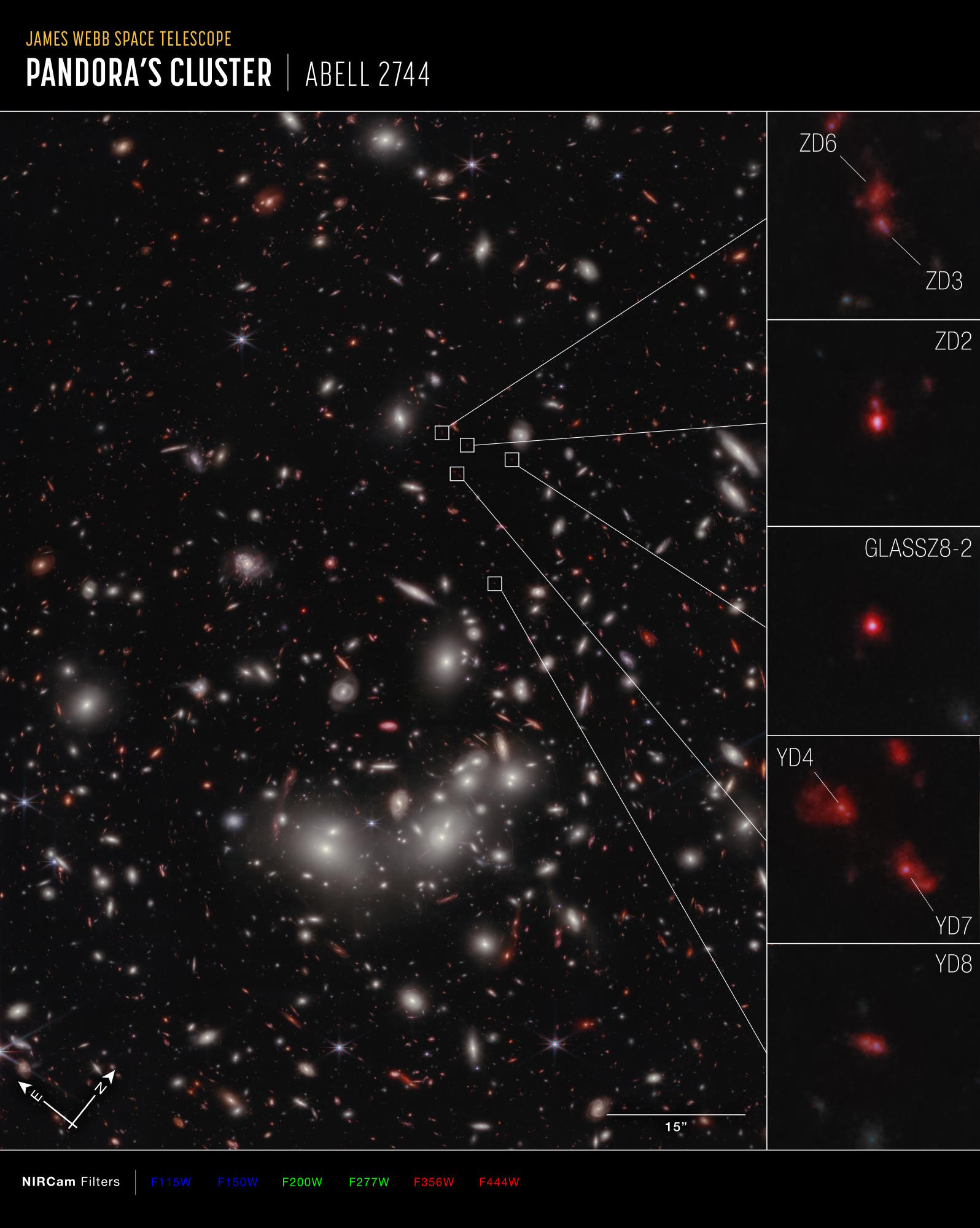
This study doesn’t simply reveal the most distant known proto-cluster of galaxies in the entire Universe to date, but also highlights how remarkably important it is to observe and spectroscopically confirm all of the distant galaxy candidates that we suspect will belong to a single object. The earlier Hubble study suggested a much larger, more expansive proto-cluster than actually exists: there are “only” about ~24 times the number of galaxies in this cluster, not the previously-estimated ~130. Some of the galaxies found were not associated with the proto-cluster, but were located elsewhere along the line-of-sight. Additionally, some candidate galaxies remain without spectra, highlighting the importance of observing them.
The authors also attempt to estimate the mass and velocity dispersion (i.e., how fast the galaxies are moving relative to one another) inside this proto-cluster, and found something remarkable. The total masses of the seven member galaxies, combined, is about 400 million Suns: almost the mass of the modern Milky Way, and this sets a lower limit on the proto-cluster’s mass. By today, it should have grown to at least 5000 times that amount, or the mass of the modern-day Coma Cluster. And the estimated velocity dispersion, of ~1100 km/s, although highly uncertain, looks remarkably consistent with known high-mass galaxy clusters.
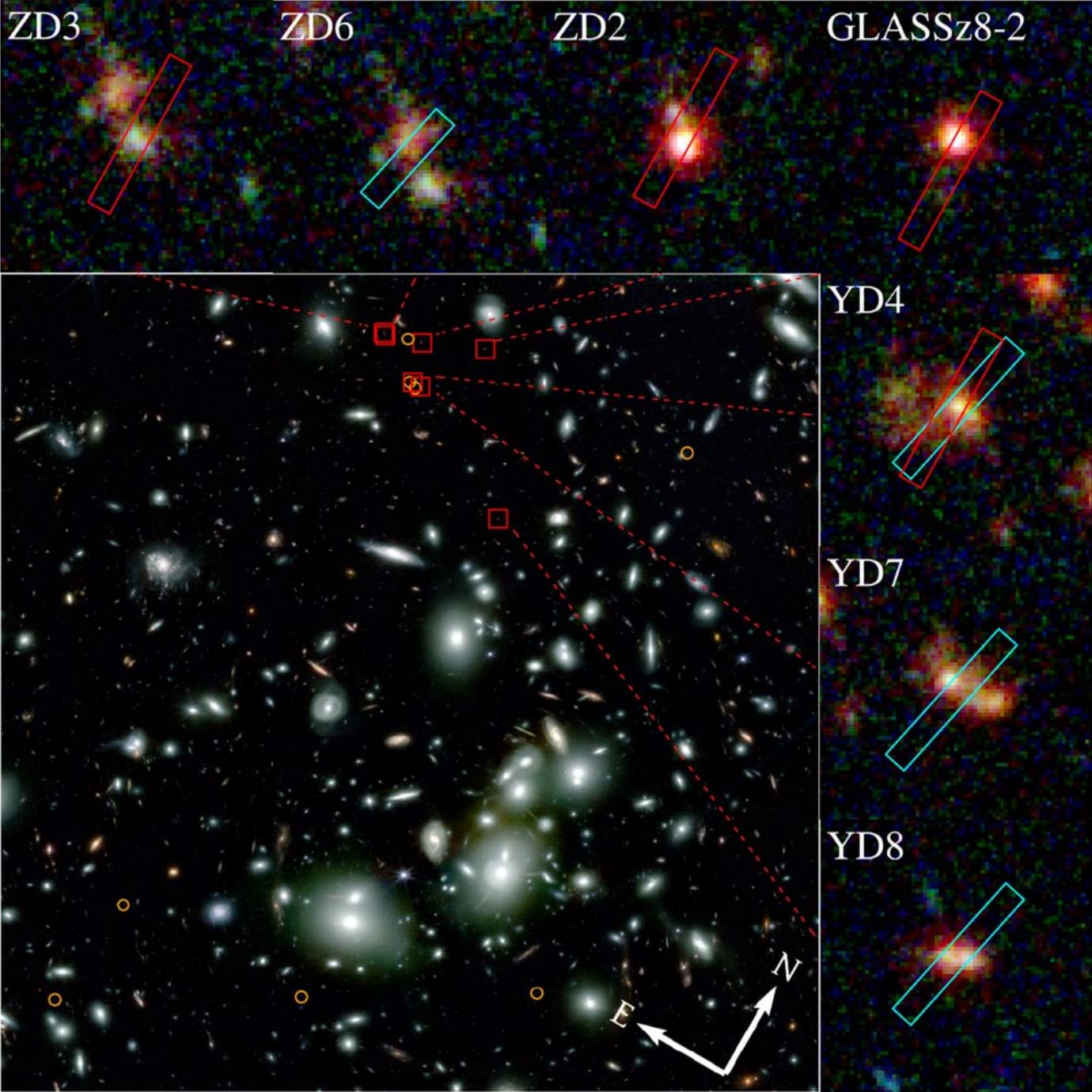
For the first time, we’re not just guessing, but actually seeing how the Universe grew up. Thanks to the incredible capabilities of JWST and the remarkable work of the scientists acquiring and analyzing data from the distant Universe, we’re constructing a more complete, comprehensive, and accurate picture of how our Universe went from a small, star-free, almost-perfectly uniform state to our tremendous, galaxy-rich cosmos of today.